ABSTRACT
This paper illustrates the geological and structural map (scale 1:10,000) of the Castellaccio Pluton (Asinara island – Italy), a peraluminous granodioritic intrusion of Variscan age that belongs to the Corsica-Sardinia Batholith. This small pluton, about 15 km2, emplaced within Paleozoic amphibolite-facies rocks during the post-collisional exhumation of the chain. The map shows the fine details of the magmatic structure of the pluton. Field-structural survey, coupled with remote sensing and major-elements geochemical mapping, allowed to recognize the occurrence of five magmatic units and the magmatic flow trajectories within the pluton. These outcomes provide useful insights into the architecture of the magmatic system. The results presented improve substantially the cartographic database of the Variscan crust of Sardinia and, finally, may serve as a robust background supporting further studies on the emplacement mechanism, or targeted to more specific petrologic studies.
1. Introduction
In spite of some recent papers about the evolution of Variscan granites in the Corsica-Sardinia massif, the geometry of plutons is still poorly known (CitationBarbey, Gasquet, Pin, & Bourgeix, 2008; CitationCasini, 2012; CitationCasini et al., 2015; CitationCasini, Cuccuru, Maino, Oggiano, & Tiepolo, 2012; CitationCasini, Cuccuru, Puccini, Oggiano, & Rossi, 2015; CitationCasini, Puccini, Cuccuru, Maino, & Oggiano, 2013; CitationCocherie, Rossi, Fanning, & Guerrot, 2005; CitationCuccuru et al., 2016; CitationKruhl & Vernon, 2007; CitationPaquette, Ménot, Pin, & Orsini, 2003). Upper-crustal, fine-grained plutons are often characterized by weak shape preferred orientation (SPO) of K-feldspar phenocrysts, micro-granular mafic enclaves (mme) and other flow markers (CitationJohnson et al., 2003; CitationPaterson, Pignotta, & Vernon, 2004), yielding to poorly constrained magmatic fabric. In these cases, the orientation of magmatic flow trajectories can be obtained by combining microstructural analysis (CitationMainprice & Nicolas, 1989; CitationMuller, Leiss, Ullemeyer, & Breither, 2011; Cuccuru, Casini, Oggiano & Cherchi, Citation2012), geophysical methods (CitationKratinova et al., 2010; CitationRomán-Berdiel, Pueyo-Morer, & Casas- Sainz, 1995; CitationSchöpa, Floess, de Saint Blanquat, Annen, & Launeau, 2015) and geochemical mapping (CitationPuccini et al., 2014). On the other hand, flow markers are generally widespread in coarse-grained plutons emplaced at deep crustal levels; therefore, the internal structure can be thoroughly determined from field-structural analysis (CitationJohnson et al., 2003; CitationMarre, 1986).
This paper focuses on the geometry and structural setting of the Castellaccio Pluton (CP), a small late-Variscan intrusion well exposed in the Asinara Island (), northern Sardinia (CitationCarosi, Di Pisa, Iacopini, Montomoli, & Oggiano, 2004). The detailed (1:10,000) geological-structural map has been obtained by integrating field geological mapping, structural analysis at the outcrop scale, remote sensing and extensive major-elements analysis, providing a substantial improvement to the cartographic database of Variscan Sardinia. Overall, the intrusion forms a thin (500–700-m-thick), elliptical, funnel-shaped sill elongated in the N100-120° direction, that is, almost parallel to the main late-Variscan shear zones crossing N Sardinia (CitationCasini et al., 2015a, Citation2015b). The pluton emplaced into amphibolite-facies metamorphic rocks forming two principal granodioritic batches of peraluminous composition. The more internal batch, exposed in the western part of the pluton, is characterized by a strongly porphyritic fabric, which is given by the occurrence of huge K-feldspar phenocrysts, mme and metamorphic xenoliths. The widespread occurrence of these suitable flow markers allowed to portray the fine details of the magmatic flow trajectories within the pluton. Hence, this map offers an excellent opportunity to study the internal geometry of a middle-crustal granitic intrusion, providing the basis for developing further emplacement models and petrogenetic studies.
2. Geological setting
The Variscan Orogeny (Devonian-Permian) reflects the subduction of the Rheic Ocean and the subsequent continental collision of Laurussia and a set of Gondwana-derived microplates (CitationMatte, 2001; CitationRossi, Oggiano, & Cocherie, 2009; CitationStampfli, von Raumer, & Borel, 2002; CitationVon Raumer, Stampfli, & Bussy, 2003). The Variscan crust () is discontinuously exposed throughout most of Central Europe, from the Bohemian Massif to the Vosges-Schwartzwald, French Massif Central, the Alps and the Iberian Massif (CitationMatte, 2001; CitationVon Raumer, Finger, Veselá, & Stampfli, 2014). The southern branch of the European Variscan Belt includes the Corsica-Sardinia Massif, a microplate drifted away from Europe in the Oligocene (CitationAlvarez, Cocozza, & Wezel, 1974). From south to north, the Sardinian crust consists of Ediacarian-Carboniferous low grade to greenschist facies metamorphic units underthrusted below the migmatitic rocks of the High-Grade Metamorphic Complex exposed in the north and north-eastern side of Sardinia (CitationCarmignani et al., 1994; CitationMassonne, Cruciani, & Franceschelli, 2013). The metamorphic rocks are intruded by a large late-Variscan volcano-plutonic province, namely the Corsica-Sardinia Batholith (hereafter C-SB). The C-SB formed in Carboniferous-Permian times, in response to crustal thinning related to the post-collisional exhumation of the chain (CitationCasini et al., 2015; CitationCasini et al., 2015; CitationPaquette et al., 2003; CitationRossi & Cocherie, 1991). The batholith is compositionally heterogeneous and composed of three main magmatic suites, distinguished on the basis of their emplacement age, chemical and isotopic composition (CitationCasini et al., 2015; CitationFerré & Leake, 2001; CitationPaquette et al., 2003):
U1 suite, the oldest plutons and equivalent volcanic sequences emplaced in the interval 345–337 Ma. U1 rocks consist of calc-alkaline Mg-K rich quartz-monzonites, monzogranites and ultra-potassic mafic rocks exposed only in the north-western side of Corsica (CitationRossi & Cocherie, 1991).
U2 calc-alkaline suite emplaced between 322 and 285 Ma within crustal-scale shear zones (CitationCasini et al., 2015; CitationCasini & Funedda, 2014; CitationCuccuru et al., 2016; CitationPaquette et al., 2003) forming the dominant component of the C-SB. These U2 granitoids, mainly monzogranites and subordinately granodiorites and leucogranites, show generally a metaluminous or slightly peraluminous character. U2 basic rocks, such as quartz-diorite and gabbroes, are rare (2–3%) and have a tholeiitic composition (CitationCasini et al., 2015; CitationCocherie et al., 2005).
the U3 sub-alkaline to alkaline suite formed in Permian times within the time-span 290–270 Ma (CitationCasini et al., 2015; CitationGaggero, Oggiano, Buzzi, Slejko, & Cortesogno, 2007; CitationRenna, Tribuzio, & Tiepolo, 2006). These rocks emplaced as small intrusions associated with volcanic and sub-volcanic complexes. U3 complexes are characterized by a large compositional variability, including intermediate to mafic terms such as gabbro, diorite and quartz-diorite, as well as more acidic compositions such as alkaline to sub-alkaline granites (CitationCocherie et al., 2005).
3. Methods
3.1 Remote sensing
A preliminary investigation of the granite-host rock contacts , as well as the geometry of the main faults and fractures network, has been carried out by the means of GIS-based morphological analysis of geo-referenced high-resolution aerial ortophotographs (property of Regione Autonoma della Sardegna, RAS) and IKONOS 2005 satellite images (for detailed information about correlated errors, spatial resolution and image accuracy, see CitationVirdis, Oggiano, & Disperati, 2012).
3.2 Field-structural analysis
A classical field-structural survey has been done to reconstruct the overall geometry of the pluton and the fabric acquired during emplacement and solidification of the magma. Large, idiomorphic K-feldspar phenocrysts, commonly present in all the granitic units, were preferentially used as flow markers and measured to reconstruct the orientation of the magmatic flow trajectories within the pluton (CitationMarre, 1986; CitationVernon, Johnson, & Melis, 2004). The flow patterns defined by k-feldspar phenocrysts have been integrated by analysing the orientation of micro-granular mafic enclaves (mme), metamorphic xenoliths and mafic schlieren, occasionally observed within specific rock units. The orientation data were then projected into stereonet diagrams to obtain information about the statistical distribution of the magmatic foliation and lineation, as well as the orientation of the main metamorphic foliation in the country rocks (). Post-magmatic faults were first detected by remote sensing, then verified on the field in order to constrain the principal offsets accommodated by the fault network.
Figure 3. The first three stereonets (a–c) show the contour plot of structural data as multiples of uniform distribution, lower-hemisphere equal area projection. The last diagram (d) shows the orientation of post-Variscan faults as rose diagram. (a) Magmatic lineation (number of data = 84, maximum density = 6.15%), (b) poles to magmatic foliation (number of data = 81, maximum density = 7.64), (c) poles to metamorphic foliation (number of data = 42, maximum density = 7.85) and (d) fault orientation pattern (number = 34, largest bin = 11%).
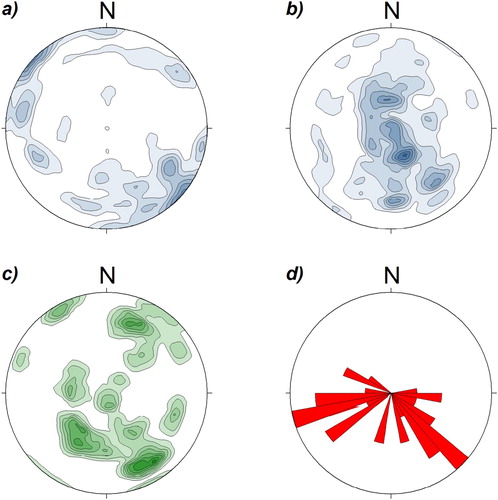
3.3 Major-elements composition
An extensive major-elements mapping was carried out to characterize the composition of granitic rocks in terms of SiO2, Na2O, K2O, CaO and Al2O3 distribution. The Aluminium Saturation Index (A/CNK) was calculated from the major-element composition as the ratio between the molar abundance of Al2O3 and the sum of the molar abundances of Na2O + CaO + K2O. About 50 representative samples were picked up avoiding weathered outcrops and keeping the sampling sites as far as possible from the main fault zones. Then, the composition of 28 selected samples () was determined by fusion ICP-MS methods at Activation Laboratories (ActLab, Ontario). Geochemical analysis has been interpolated using a natural-neighbours algorithm and the results are shown as compositional maps ().
Figure 4. Major-elements distribution maps. In all maps, the margin of the pluton is indicated by a solid line, the dotted line crossing the pluton indicates the approximate contact between the root zone (to the W) and the apical part of the pluton (to the E): (a) spatial variation of A/CNK ratio, (b) spatial variation of SiO2, (c) spatial variation of Al2O3, (d) map of Na2O, (d) K2O map and (e) spatial variation of CaO.
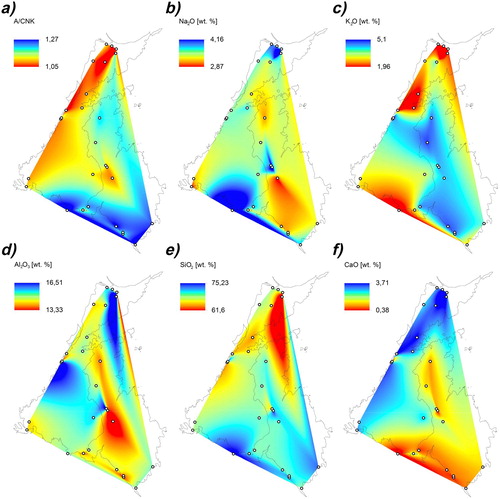
Table 1. Major-elements composition and A/CNK value of selected samples.
4. Results
4.1. Cartographic Units
The metamorphic rocks around the pluton form a rather homogeneous sequence of staurolite ± kyanite ± garnet micaschists, paragneisses and quartzites. Therefore, as the map focuses on Variscan granites, metamorphic rocks have been merged into a single rock unit labelled as the Medium Grade Metamorphic Complex (MGMC), following on from the formational name proposed in CitationCappelli et al. (1992). Five different magmatic units have been distinguished within the CP, such as: (i) Tumbarino (CPa), (ii) Castellaccio (CPb), (iii) Scalpellini (CPc), (iv) M.te Garau (CPd) and, finally, (v) the late-plutonic dykes (CPe). The proposed classification scheme is primarily based on field-structural criteria, such as mean grain size, occurrence/absence of muscovite, pegmatite pockets, relative abundance and size of K-feldspar phenocrysts, and abundance of mafic enclaves.
4.1.1. Contact metamorphic aureole
The contact metamorphic aureole developed around the pluton has been distinguished into two zones on the basis of mineral assemblages. The inner zone (MMCa) is characterized by the coexistence of cordierite, andalusite and biotite. This high-temperature assemblage is apparently developed only along the northern side of the pluton, reaching a maximum thickness of about 100–120 m. In the outer zone (MMCb), the contact metamorphic assemblage consists of only biotite. This relatively low-temperature zone is very wide (500–1200 m) along the northern side of the pluton and vanishes in the southern margin, reaching a minimum thickness of about 10–20 m. The inner zone of the contact metamorphic aureole is characterized by the occurrence of several melt-bearing ductile shear zones that deform the main Variscan foliation. These structures are generally narrow (1–10 m) and contain thin (0.1–0.5 m), discontinuous, dykes or pods of highly evolved leuco-monzogranitic melts composed of the typical assemblage quartz + k-feldspar + plagioclase + muscovite + garnet ± biotite ± tourmaline.
4.1.2. Tumbarino Unit
The Tumbarino Unit (CPa) dominates in the north-eastern part of the pluton and is exposed, more discontinuously, along the western margin. CPa consists of coarse-grained granodiorites with a mean grain size of 3–5 cm ((a)). These rocks are characterized by the widespread occurrence of cumulitic domains (CitationVernon & Collins, 2011) consisting of up to 60–75% vol. of large K-feldspar phenocrysts, micro-granitoid enclaves and mme ((a,b)). In the type locality close to the Tumbarino village (Main map), the CPa granodiorites contain often 15–20 cm-large idiomorphic K-feldspar phenocrysts and abundant mme with strongly elliptical shape.
Figure 5. Magmatic units of the Castellaccio Pluton: (a) Granodiorite with mafic enclaves (CPa); (b) porphyritic granodiorite (CPb); (c) Late-magmatic acid dyke (CPe) intruding granodioritic-monzogranitic rocks (CPc); (d) detail of fine-grained leuco-monzogranite (CPd); (e) contact between a coarse-grained granodiorite (CPb) and leuco-granodiorite (CPc), the contact is injected by a thin acidic dyke (CPe).
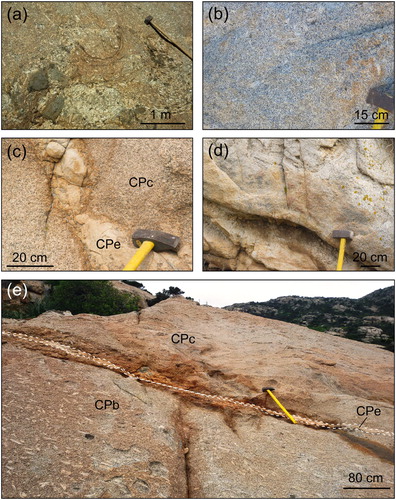
Figure 6. Magmatic structures: (a) detail of cumulitic domains (cd) within the Tumbarino Unit (CPa); (b) close-up of magmatic mafic enclave (mme) cut by a metamorphic xenolith (xe), Tumbarino Unit (CPa); (c) detail of a large idiomorphic K-feldspar with several overgrowths, Castellaccio Unit (CPb); (d) late-magmatic acid dyke (CPe) parallel to the main magmatic foliation defined by K-feldspar phenocrysts in Castellaccio Unit (CPb).
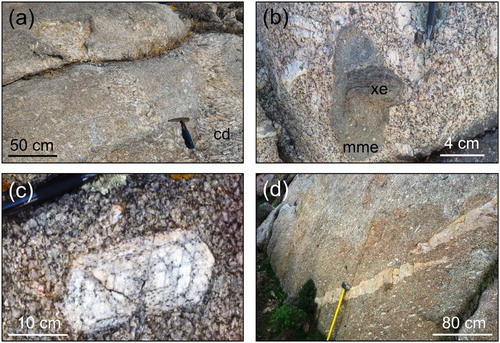
4.1.3. Castellaccio Unit
The Castellaccio Unit (CPb) is exposed in the western part of the pluton and represents the volumetrically dominant rock type. CPb consists of large- to medium-grained porphyritic granodiorites characterized by huge K-feldspar phenocrysts that range in size from about 4 up to 10 cm ((b) and (c)). In some places, rare and generally small mme can be observed altogether with thin and somewhat discontinuous acidic dykes marking the trajectory of the magmatic fabric ((d)).
4.1.4. Scalpellini Unit
The Scalpellini Unit (CPc) crops out in the eastern side of the map area and can be also observed discontinuously along the contact with the metamorphic host rocks in the south-western and north-eastern margins of the pluton (Main map). CPc is characterized by much finer grain size (0.5–3 cm) and contains rare pegmatites. Micro-granular mafic enclaves are very rare or absent and K-feldspar phenocrysts are generally smaller (1–3 cm) than those exposed in the other units ((c)).
4.1.5. Mte Garau Unit
The M.te Garau Unit (CPd) appears in the south-eastern part of the study area and lies structurally above the CPc unit. It consists of muscovite ± garnet-bearing leuco-monzogranites that are characterized by very fine grain size (0.5–1 cm) and shows distinctive features of chilled margins, including: (i) globular or interstitial quartz domains, (ii) biotite schlieren, (iii) metamorphic xenoliths and finally (iv) diffuse micro-pegmatite pockets. K-feldspar phenocrysts and mme are extremely rare or absent ((d)). At places, melts with a composition similar to CPd unit and characterized by the presence of large and idiomorphic garnet crystals can be found within the melt-bearing shear zones developed in the inner part of the metamorphic aureole (Main Map).
4.1.6. Late-Plutonic Dykes
Finally, the late-plutonic dike complex and the system of aplitic veins, widely exposed in the north-western part of the study area, have been collectively indicated as CPe Unit ((c,e)). Dykes are extremely variable in thickness (0.1–10 m) and range in orientation from sub-vertical, steep, to nearly horizontal. At the map scale, dykes increase toward the north-western side of the pluton and become progressively thicker and more steep around the northern termination of the pluton (Main map).
4.1.7. Post-Variscan Units
All post-Variscan Units, represented mainly by Early Permian to Holocene sedimentary covers and hydrothermal quartz veins (HQVs), are poorly exposed in the study area. Sedimentary rocks (PVS) were grouped into a single lithostratigraphic unit for the sake of simplicity. The HQVs generally appear along the main post-Variscan faults, though they reach a thickness suitable for mapping only along two of the main NNE-SSW sinistral and N-S dextral strike-slip faults (Main map).
4.2. Geometry of the CP
All the magmatic units show a well-oriented magmatic fabric, marked by the strong SPO of K-feldspar phenocrysts, mafic enclaves and metamorphic xenoliths. The poles to the magmatic foliation generally show a bimodal distribution with two local maxima oriented toward NNW and SSE directions, respectively (). The magmatic foliation defined by k-feldspar phenocrysts, metamorphic xenoliths and mme is generally flat or gently dipping, though it may turn to be locally steep close to the margins of the pluton (). Only the northern zone of the pluton, around the Tumbarino village, is characterized by a sub-vertical foliation oriented ENE-WSW, which is almost parallel to the contact with the wall rock (Main Map). On the other hand, the magmatic lineation is always gently dipping to the SE or NW, with a minor cluster oriented toward NE, and only few data are slightly dipping toward W-SW (Main Map). Overall, the geometry of the pluton is therefore consistent with that of a stretched oblate ellipsoid that can be interpreted in terms of an NW-SE-elongated sill-like body, or a funnel-shaped laccolith. The contacts between the granitic units and the metamorphic basement along the southern and south-eastern margins of the pluton are mostly decorated by a thick, continuous, belt composed of chilled margin-type rocks (CPd or CPc) that reach a maximum thickness of about 20–30 m These rocks show distinctive features typical of quenched melts, such as very fine grain size (0.5–2 mm), globular quartz aggregates, and rare to absent k-feldspar phenocrysts. These observations are broadly consistent with the occurrence of diffuse micro-pegmatite pockets and metamorphic xenoliths along the southern margin of the pluton, confirming that both the CPd and CPc units represent rapidly cooled magmatic batches emplaced in the external and apical part of the magmatic chamber. Melts with a strikingly similar composition to that of CPd unit also occur within steep, almost vertical, melt-bearing ductile shear zones that characterize the inner contact metamorphic aureole in the NW sector of the map (Main Map). In the northern side of the pluton, the chilled margin is more discontinuous and usually very thin (<10–50 cm) or absent, indicating that the CPa and CPb units represent slowly cooled, internal magma batches. The internal contacts between the different granitic units of the CP are hot, mostly ductile and transitional and, unless local perturbations, are mostly parallel to the magmatic flow foliation. Pegmatite pockets and aplitic dykes are only rarely observed along these internal contacts, indicating slow and relatively homogeneous cooling of the different magma batches. Conversely, the contacts between the metamorphic basement and the granites, as well as the contacts between dykes and all the other units, are generally cold and sharp. Along the contact with the granite, in fact, the metamorphic fabric is generally discordant with, and truncated by, the magmatic flow trajectories, indicating a clear relative timing.
4.3. Major-elements composition
The spatial variation of major-element composition within the CP, at a resolution of about 50 × 50 m, is obtained by interpolating the SiO2, Na2O, Al2O3, CaO, K2O and A/CNK values of 28 selected samples using a natural-neighbours algorithm. The geochemical maps () show that both the Na2O and K2O contents tend to increase as the SiO2 content of the rocks increases. On the other hand, the Al2O3 and CaO components have an opposite behaviour, being more abundant in the less acidic rocks. These general trends correspond to the observed increase of the A/CNK toward the eastern and southern margins of the CP (). Therefore, the spatial variation of major-elements composition and A/CNK values indicates that the southern and south-eastern margins of the pluton represent the more acidic, and thus more evolved, part of the magmatic system. Overall, this observation is fairly consistent with the extensive presence of muscovite and garnet in the Mte Garau and Scombro units (CPd, CPe), indicating these as late-magmatic batches. The occurrence of lowest A/CNK values coupled to the increase of both the Al2O3 and CaO components toward the northern side of CP indicates this zone as the less evolved part of the magmatic system, that is, the pluton’s roots zone where the melt was sourced from. The development of a steep magmatic fabric close to the contact with the metamorphic basement around the Tumbarino village, together with the substantial increase of mme in the CPa rock, confirms this simplified pluton model.
4.4. Tectonic structures
The study area is characterized by the occurrence of both melt-bearing ductile shear zones and brittle faults. Shear zones are localized in the north-western sector of the map, along the contact between the metamorphic basement and the pluton. These narrow (1–10 m of thickness) structures are apparently related to the growth of the magmatic system, as they are injected by sin-tectonic aplitic-pegmatitic dykes characterized by a composition which is indistinguishable from that of the more evolved facies of the pluton (i.e. CPd). Several strike-slip faults likely related to the Oligo-Miocene rotation and extension of the Corsica-Sardinia massif (CitationFunedda, Oggiano, & Pasci, 2000; CitationOggiano, Funedda, Carmignani, & Pasci, 2009) offset the pluton and the melt-bearing shear zones modifying their original shape. The main brittle structures consist of two dextral strike-slip faults with a roughly E-W direction and an NNE-SSW sinistral fault that cut through the pluton in the northern part of the map area, between Cala Scombro bay and Tumbarino village (Main Map). Other conjugate minor faults show both SW-NE and NW-SE directions. Finally, a large, E-dipping low-angle normal fault (LANF) was identified in the south-eastern side of the pluton, between Fornelli and P. Galetta localities (Main Map). Based on structural correlations with similar faults observed elsewhere in N Sardinia at the contact between granites and metamorphic rocks, this LANF can be tentatively interpreted as one of the regional structures developed during the late-Variscan extension of the chain (i.e. Casini & Oggiano, Citation2008). However, in this case a post-Variscan age (Triassic-Oligocene) cannot be ruled out in the absence of reliable stratigraphic markers. Regardless of the timing of fault motion, given the general sill-like geometry of the pluton, this extensional structure accounts for both the exhumation of pluton roots in the western and northern part of the study area, and preservation of the uppermost levels and remnants of the apical part in the hanging wall of the LANF.
5. Conclusions
Field-structural analysis coupled to extensive major-element geochemical mapping allowed to draw an accurate geological and structural map of the geometry of the CP, a Variscan granite exposed in the southern Asinara island. The orientation of magmatic flow markers, such as k-feldspar phenocrysts and mme, provided robust constraints on the magmatic flow trajectories and the relevant geometry of the pluton. Overall, the flat magmatic foliation and the nearly horizontal lineation indicate that the geometry of the pluton match that of a funnel-shaped sill, slightly elongated in an NW-SE direction. This geometry is typical of middle- to upper-crustal intrusions emplaced within strike-slip shear zones. The preservation of a steep fabric only around the Tumbarino village indicates that the root zone of the pluton is presently exposed to the north, close to the contact with the wall rock. This interpretation is broadly consistent with the observation of a narrow chilled margin around the northern side of the pluton, which testifies a small temperature difference between the magma and the wall rock at the time of emplacement. On the other hand, the presence of diffuse micro-pegmatite pockets and the overall flat foliation observed around M.Te Garau indicate that the south-eastern margin of the granitic massif likely represent the apical part of the pluton, that is, the roof zone.
Software
The map was digitized using Esri ArcMAP10.0. Stereoplots were realized with the software Stereonett and then redraw with Macromedia Freehand10. Geological sections were drawn with Macromedia Freehand10. Geostatistical interpolations were carried out by Esri ArcMAP10.0.
Main Map.pdf
Download PDF (2.1 MB)Acknowledgements
The institution Parco Nazionale Isola dell’Asinara is greatly acknowledged for authorizing access to the study area and for logistic support provided to E. Simula during fieldwork. The authors are grateful to the referees for their useful comments.
Disclosure statement
No potential conflict of interest was reported by the authors.
ORCID
Stefano Cuccuru http://orcid.org/0000-0003-1236-1498
Leonardo Casini http://orcid.org/0000-0001-8965-4390
Giacomo Oggiano http://orcid.org/0000-0003-1236-1498
References
- Alvarez, W., Cocozza, T., & Wezel, F. C. (1974). Fragmentation of the Alpine orogenic belt by microplate dispersal. Nature, 248, 309–314. doi: 10.1038/248309a0
- Barbey, P., Gasquet, D., Pin, C., & Bourgeix, A. L. (2008). Igneous banding, schiere and mafic enclaves in calc-alkaline granites: The Buddusò pluton (Sardinia). Lithos, 104, 147–163. doi: 10.1016/j.lithos.2007.12.004
- Cappelli, B., Carmignani, L., Castorina, F., Di Pisa, A., Oggiano, G., & Petrini, R. (1992). A Hercynian suture zone in Sardinia: Geological and geochemical evidence. Geodinamica Acta, 5(1–2), 101–118. doi: 10.1080/09853111.1992.11105222
- Carmignani, L., Carosi, R., Di Pisa, A., Gattiglio, M., Musumeci, G., Oggiano, G., & Pertusati, P. C. (1994). The Hercynian chain in Sardinia (Italy). Geodinamica Acta, 7, 31–47. doi: 10.1080/09853111.1994.11105257
- Carosi, R., Di Pisa, A., Iacopini, D., Montomoli, C., & Oggiano, G. (2004). The structural evolution of the Asinara Island (NW Sardinia, Italy). Geodinamica Acta, 17(5), 309–329. doi: 10.3166/ga.17.309-329
- Casini, L., & Oggiano, G. (2008). Late orogenic collapse and thermal doming in the northern Gondwana margin incorporated in the Variscan Chain: a case study from the Ozieri Metamorphic Complex, Northern Sardinia, Italy. Gondwana Research, 13, 396–406.
- Casini, L. (2012). A MATLAB-derived software (geothermMOD1.2) for one-dimensional thermal modeling, and its application to the Corsica-Sardinia batholith. Computers and Geosciences, 45, 82–86. doi: 10.1016/j.cageo.2011.10.020
- Casini, L., Cuccuru, S., Maino, M., Oggiano, G., Puccini, A., & Rossi, P. (2015b). Structural map of Variscan northern Sardinia (Italy). Journal of Maps, 11(1), 75–84. doi: 10.1080/17445647.2014.936914
- Casini, L., Cuccuru, S., Maino, M., Oggiano, G., & Tiepolo, M. (2012). Emplacement of the Arzachena Pluton (Corsica–Sardinia Batholith) and the geodynamics of incoming Pangaea. Tectonophysics, 544–545, 31–49. doi: 10.1016/j.tecto.2012.03.028
- Casini, L., Cuccuru, S., Puccini, A., Oggiano, G., & Rossi, P. (2015a). Evolution of the Corsica–Sardinia Batholith and late-orogenic shearing of the Variscides. Tectonophysics, 646, 65–78. doi: 10.1016/j.tecto.2015.01.017
- Casini, L., & Funedda, A. (2014). Potential of pressure solution for strain localization in the Baccu Locci Shear Zone (Sardinia, Italy). Journal of Structural Geology, 66, 188–204. doi: 10.1016/j.jsg.2014.05.016
- Casini, L., Puccini, A., Cuccuru, S., Maino, M., & Oggiano, G. (2013). GEOTHERM: A finite difference code for testing metamorphic P-T-t paths and tectonic models. Computers and Geosciences, 59, 171–180. doi: 10.1016/j.cageo.2013.05.017
- Cocherie, A., Rossi, P., Fanning, C. M., & Guerrot, C. (2005). Comparative use of TIMS and SHRIMP for U–Pb zircon dating of A-type granites and mafic tholeiitic layered complexes and dykes from the Corsican Batholith (France). Lithos, 82, 185–219. doi: 10.1016/j.lithos.2004.12.016
- Cuccuru, S., Casini, L., Oggiano, G., & Cherchi, G. P. (2012). Can weathering improve the toughness of a fractured rock? A case study using the San Giacomo granite. Bulletin of Engineering Geology and Environment, 71, 557–567. doi: 10.1007/s10064-012-0416-9
- Cuccuru, S., Naitza, S., Secchi, F., Puccini, A., Casini, L., Pavanetto, P., … Oggiano, G. (2016). Structural and metallogenic map of late Variscan Arbus Pluton (SW Sardinia, Italy). Journal of Maps, 12(5), 860–865. doi: 10.1080/17445647.2015.1091750
- Ferré, E. C., & Leake, B. E. (2001). Geodynamic significance of early orogenic high-K crustal and mantle melts: Example of the Corsica Batholith. Lithos, 59, 47–67. doi: 10.1016/S0024-4937(01)00060-3
- Funedda, A., Oggiano, G., & Pasci, S. (2000). The Logudoro basin: A key area for the tertiary tectono-sedimentary evolution of North Sardinia. Bollettino Società Geologica Italiana, 119, 31–38.
- Gaggero, L., Oggiano, G., Buzzi, L., Slejko, F., & Cortesogno, L. (2007). Post-Variscan mafic dykes from the late orogenic collapse to the Tethyan rift: Evidence from Sardinia. Ofioliti, 32, 15–37.
- Johnson, S. E., Fletcher, J. M., Fanning, C. M., Vernon, R. H., Paterson, S. R., & Tate, D. (2003). Structure, emplacement and lateral expansion of the San José tonalite pluton, Peninsular Ranges batholiths, Baja California, México. Journal of Structural Geology, 25, 1933–1957. doi: 10.1016/S0191-8141(03)00015-4
- Kratinova, Z., Jezek, J., Schulmann, K., Hrouda, F., Shail, R. K., & Lexa, O. (2010). Noncoaxial K-feldspar and AMS subfabrics in the Land's End granite, Cornwall: Evidence of magmatic fabric decoupling during late deformation and matrix crystallization. Journal of Geophysical Research-Solid Earth, 115(9), B09104. doi: 10.1029/2009JB006714
- Kruhl, J. H., & Vernon, R. S. (2005). Syndeformational emplacement of a tonalitic sheet complex in a late-Variscan thrust regime: Fabrics and mechanism of intrusion, Monte’e Senes, northeastern Sardinia. Canadian Mineralogy, 43, 387–407. doi: 10.2113/gscanmin.43.1.387
- Mainprice, D., & Nicolas, A. (1989). Development of shape and lattice preferred orientation: Application to the seismic anisotropy of the lower crust. Journal of Structural Geology, 11(1/2), 175–189. doi: 10.1016/0191-8141(89)90042-4
- Marre, J. (1986). The structural analysis of granitic rocks. Oxford: North Oxford Academic.
- Massonne, H.-J., Cruciani, G., & Franceschelli, M. (2013). Geothermobarometry on anatectic melts-a high-pressure Variscan migmatite from northeast Sardinia. International Geology Reviews, 55(12), 1490–1505. doi: 10.1080/00206814.2013.780720
- Matte, P. (2001). The Variscan collage and orogeny (480-290 Ma) and the tectonic definition of the Armorica microplate: A review. Terra Nova, 13(2), 122–128. doi: 10.1046/j.1365-3121.2001.00327.x
- Muller, A., Leiss, B., Ullemeyer, K., & Breither, K. (2011). Lattice-preferred orientation of late-Variscan granitoids derived from neutron diffraction data: Implications for magma emplacement mechanisms. International Journal of Earth Sciences, 100(1), 1515–1532. doi: 10.1007/s00531-010-0590-6
- Oggiano, G., Funedda, A., Carmignani, L., & Pasci, S. (2009). The Sardinia–Corsica microplate and its role in the Northern Apennine Geodynamics: New insights from the Tertiary intraplate strike-slip tectonics of Sardinia. Bollettino della Società Geologica Italiana, 128, 227–239.
- Paquette, J.-L., Ménot, R.-P., Pin, C., & Orsini, J.-B. (2003). Episodic and short-lived granitic pulses in a post-collisional setting: Evidence from precise U-Pb zircon dating through a crustal cross-section in Corsica. Chemical Geology, 198, 1–20. doi: 10.1016/S0009-2541(02)00401-1
- Paterson, S. R., Pignotta, G. S., & Vernon, R. H. (2004). The significance of microgranitoid enclave shake and orientations. Journal of Structural Geology, 26(8), 1465–1481. doi: 10.1016/j.jsg.2003.08.013
- Puccini, A., Xhixha, G., Cuccuru, S., Oggiano, G., Xhixha, M. K., Mantovani, F., … Casini, L. (2014). Radiological characterization of granitoid outcrops and dimension stones of the Variscan Corsica-Sardinia Batholith. Environmental Earth Sciences, 71(1), 393–405. doi: 10.1007/s12665-013-2442-8
- Renna, M. R., Tribuzio, R., & Tiepolo, M. (2006). Interaction between basic and acid magmas during the latest stages of the post-collisional Variscan evolution: clues from the gabbro–granite association of Ota (Corsica–Sardinia batholith). Lithos, 90, 92–110. doi: 10.1016/j.lithos.2006.02.003
- Román-Berdiel, T., Pueyo-Morer, E. L., & Casas- Sainz, M. (1995). Granite emplacement during contemporary shortening and normal faulting: Structural and magnetic study of the Veiga Massif (NW Spain). Journal of Structural Geology, 17(12), 1689–1706. doi: 10.1016/0191-8141(95)00062-I
- Rossi, P., & Cocherie, A. (1991). Genesis of a Variscan batholith: Field, petrological and mineralogical evidence from the Corsica-Sardinia batholith. Tectonophysics, 195(2–4), 319–346. doi: 10.1016/0040-1951(91)90219-I
- Rossi, P., Oggiano, G., & Cocherie, A. (2009). A restored section of the “southern Variscan realm” across the Corsica-Sardinia microcontinent. Comptes Rendus – Geosciences, 341(2-3), 224–238. doi: 10.1016/j.crte.2008.12.005
- Schöpa, A., Floess, D., de Saint Blanquat, M., Annen, C., & Launeau, P. (2015). The relation between magnetite and silicate fabric in granitoids of the Adamello Batholith. Tectonophysics, 642, 1–15. doi: 10.1016/j.tecto.2014.11.022
- Stampfli, G. M., von Raumer, J., & Borel, G. (2002). The paleozoic evolution of pre-Variscan terranes: From Gondwana to the Variscan collision. Geological Society of America, 364, 263–280.
- Vernon, R., & Collins, W. J. (2011). Structural criteria for identifying granitic cumulates. The Journal of Geology, 119(2), 127–142. doi: 10.1086/658198
- Vernon, R. H., Johnson, S. E., & Melis, E. A. (2004). Emplacement-related microstructures in the margin of adeformed pluton: The San José Tonalite, Baja California, Mexico. Journal of Structural Geology, 26(10), 1867–1884. doi: 10.1016/j.jsg.2004.02.007
- Virdis, S. G. P., Oggiano, G., & Disperati, L. (2012). A geomatics approach to multitemporal shoreline analysis in western mediterranean: The case of Platamona-Maritza Beach (Northwest Sardinia, Italy). Journal of Coastal Research, 28(3), 624–640. doi: 10.2112/JCOASTRES-D-11-00078.1
- Von Raumer, J. F., Finger, F., Veselá, P., & Stampfli, G. M. (2014). Durbachites-Vaugnerites – a geodynamic marker in the central European Variscan orogen. Terra Nova, 26(2), 85–95. doi: 10.1111/ter.12071
- Von Raumer, J. F., Stampfli, G. M., & Bussy, F. (2003). Gondwana-derived microcontinents – the constituents of the Variscan and Alpine collisional orogens. Tectonophysics, 365, 7–22. doi: 10.1016/S0040-1951(03)00015-5