ABSTRACT
A 1:850 detailed map of the geomorphology of the Dynamisk Creek alluvial fan on Svalbard was produced based on very high-resolution data collected using an unmanned aerial vehicle (UAV) in 2014. The map documents features within the surface of the fan (0.35 km2), emphasizing their relative temporal activity. Fluvial flows were the most important geomorphological processes responsible for aggradation and modification of the fan surface. However, at present most of the fan area is inactive (about 75% of the whole fan surface) and stabilized by vegetation and mosses. Furthermore, in some places, niveo-aeolian (6% of the fan surface) and snow avalanches (1.5% of the fan surface) deposits have been noticed. In addition to the map, detailed morphometric characteristics of the fan and its catchment are also provided. The presented work illustrates the enormous usefulness of small UAVs for the study of fan surfaces in periglacial environments.
1. Introduction
Fan-shaped landforms are notable depositional geomorphic features and significant elements of the landscape. Firstly, fans are potentially valuable archives of current and previous environmental dynamics, all of which are recorded in the fan morphology (e.g. CitationBallantyne & Whittington, 1999; CitationBlikra & Nemec, 1998; CitationBollschweiler & Stoffel, 2010; Citationde Haas, Ventra, Carbonneau, & Kleinhans, 2014; CitationDühnforth, Densmore, Ivy-Ochs, & Allen, 2008; CitationDühnforth, Densmore, Ivy-Ochs, Allen, & Kubik, 2007; CitationStoffel, Conus, Grichting, Lièvre, & Maître, 2008). Therefore, an investigation into contemporary transformations of fan surface is necessary for a proper interpretation of past events. Secondly, some of the low-gradient fans are used for human settlement in mountainous areas. Fan-related processes like sudden debris-flows or snow avalanches can pose a risk to human life and infrastructure (e.g. CitationAllen, Schneider, & Owens, 2009; CitationArbellay, Stoffel, & Bollschweiler, 2010; CitationBollschweiler & Stoffel, 2010; Citationde Scally, Owens, & Louis, 2010; CitationEngel, Česák, & Escobar, 2011; CitationOwen, Hiemstra, Matthews, & McEwen, 2010; CitationPetrakov et al., 2007; CitationStolz & Huggel, 2008). This issue is especially important in densely populated mountain regions; however, such processes are observed and can be dangerous, even in very low populated areas like Svalbard and Iceland (CitationAndré, 1995; CitationGlade, 2005; CitationJahn, 1967; CitationLarsson, 1982). In addition, fans and fan-related processes are also used as modern analogs for extra-terrestrial landforms (cf. CitationArfstrom & Hartmann, 2005; CitationBanks et al., 2008; CitationBernhardt, Reiss, Hiesinger, Hauber, & Johnsson, 2017; Citationde Haas, Kleinhans, Carbonneau, Rubensdotter, & Hauber, 2015; CitationHauber et al., 2009; CitationHauber et al., 2011; CitationReiss et al., 2011).
Fans occur in all climatic zones. However, the significance of specific processes responsible for aggradation and transformation of their surface might differ, depending on local and regional environmental settings (e.g. CitationBallantyne, 2002; CitationBlair & McPherson, 2009; CitationBlikra & Nemec, 1998; Citationde Haas et al., 2015; CitationRaczkowska, 1990; CitationRyder, 1971; CitationSaito & Oguchi, 2005; CitationSenderak, Kondracka, & Gądek, 2017). Therefore, for the monitoring and explanation of environmental changes, as well as a more proper interpretation of extra-terrestrial (e.g. martial) landforms, detailed investigations into the following are essential: (1) the character of the fan surface; (2) the processes operating over time and space; and (3) how these different processes can affect the fan surface.
So far, a large number of fan-related studies have focused on arid environments. In contrary, our knowledge of fans in polar areas is less extensive despite the existing research (e.g. CitationÅkerman, 1984; CitationBlikra & Nemec, 1998; CitationDecaulne & Saemundsson, 2006; CitationDecaulne & Sæmundsson, 2010; CitationDecaulne, Sæmundsson, & Jónsson, 2009; CitationDecaulne, Sæmundsson, Jónsson, & Sandberg, 2007; CitationJahn, 1960, Citation1967, Citation1976; CitationKostrzewski et al., 1989; CitationRapp & Strömquist, 1976). Therefore, there is a need for more detailed studies of fans in cold environments.
The purpose of this paper is to present a detailed map of the contemporary geomorphic surface of the Dynamisk Creek (Norwegian: Dynamiskbekken) fan (Svalbard), contributing to current knowledge on alluvial fans located in periglacial environments. Additionally, we demonstrate the applicability of unmanned aerial vehicles (UAVs, aka drones) for detailed mapping of fan-shaped landforms.
2. Study settings
The study area is located in the vicinity of Petuniabukta, the innermost branch of Isfjorden, in the central part of Spitsbergen Island in the Svalbard archipelago ().
Figure 1. Context maps of the study area: (A) Map of the Arctic area with the location of Svalbard archipelago highlighted; (B) Map of Svalbard archipelago with the vicinity of Petuniabukta study area indicated in pink; (C) Map of the vicinity of Petuniabukta with location of the Dynamisk Creek fan highlighted; (D) The Dynamisk Creek fan area: black lines indicate location of the profiles shown in ; Red rectangles marked with letters A-H indicate locations of the mapped surface, as shown in .
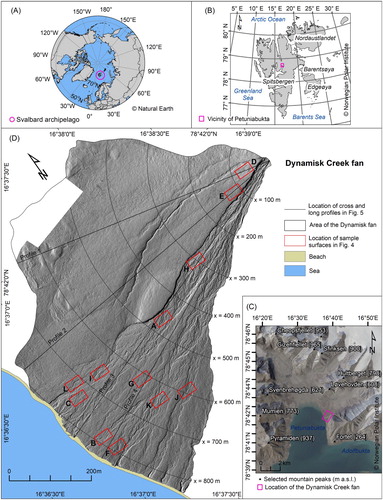
Geologically, the Petuniabukta region is conditioned by the Billefjorden fault zone (a north–south orientation), which results in various types of exposed bedrock related to Precambrian (various crystalline), Devonian (mainly sandstones and mudstones), Carboniferous-Permian (conglomerates, sandstones, mudstones, limestones, coal, gypsum, anhydrites, and dolomites), and Quaternary (alluvium, colluvium, and landslide debris, as well as glacial, fluvioglacial, marine, intertidal and beach deposits) (CitationHarland et al., 1974; CitationLamar & Douglass, 1995).
In comparison to the coastal areas, there is a relatively small ice cover in the central part of Spitsbergen. Moreover, all the glaciers in the study area have lost mass since the end of the Little Ice Age (LIA) (CitationEwertowski & Tomczyk, 2015; CitationMałecki, 2016; CitationRachlewicz, Szczuciński, & Ewertowski, 2007). Modern relief has been shaped by several glacial periods, spread with glaciers and ice-caps retreat, periglacial conditions and marine transgressions (cf. CitationEvans, Strzelecki, Milledge, & Orton, 2012; CitationEwertowski, 2014; CitationEwertowski et al., 2019; CitationEwertowski, Evans, Roberts, & Tomczyk, 2016; CitationEwertowski, Kasprzak, Szuman, & Tomczyk, 2012; CitationGibas, Rachlewicz, & Szczuciński, 2005; CitationGonera & Kasprzak, 1989; CitationHanáček, Flašar, & Nývlt, 2011; CitationKarczewski, 1989; CitationKarczewski et al., 1990; CitationKarczewski & Kłysz, 1994; CitationKłysz, 1985; CitationKłysz, Lindner, Marks, & Wysokiński, 1989; CitationRachlewicz, 2009, Citation2010; CitationRachlewicz & Szczuciński, 2008; CitationStankowski, Kasprzak, Kostrzewski, & Rygielski, 1989; CitationStrzelecki, 2011; CitationSzuman & Kasprzak, 2010). There are a large number of U-shaped post-glacial valleys between mountain ridges, reaching an elevation of up to 1024 m a.s.l. (summit of De Geerfjellet). In addition, fan-shaped landforms around Petuniabukta have developed along valley sides with the largest representation of colluvial fans (n = 229), followed by debris-flow-dominated (n = 49) and then fluvial-flow-dominated (n = 19) fans — see CitationTomczyk and Ewertowski (2017) for details.
Rockfalls, snow avalanches, debris flows, fluvial flows, and slow mass-wasting processes constitute the main processes responsible for transport of material from the catchment onto the fan surfaces (Citationde Haas et al., 2015; CitationTomczyk & Ewertowski, 2017). However, there is continuous permafrost with a maximum depth of active layer thickness that varies from 1.2–2.5 m (CitationRachlewicz & Szczuciński, 2008), limiting the volume of sediments available for transport.
The climate in the central part of Spitsbergen is arctic, and in the vicinity of Petuniabukta in 2009 the monthly mean air temperature ranged from −17.0°C (April) to 7.2°C (July), with the mean average temperature at −4.5°C. The yearly mean precipitation was low, only reaching about 150–200 mm (CitationKostrzewski et al., 1989; CitationRachlewicz, 2003). There was permanent snow cover for about nine months of the year (from the beginning of October to the first half of June) (CitationLáska, Witoszová, & Prošek, 2012). As a result, there is a relatively narrow time-window available for geomorphological processes to take place, although, it is also possible to observe effects of snowmelt during the winter time almost every month of this period (CitationPrzybylak & Araźny, 2006).
Vegetation cover is formed by the high Arctic Dryas octopetala tundra type, with polar willow (Salix polaris) and white dryas (Dryas octopetala) (CitationRønning, 1996). Nevertheless, it can be low in density or absent on steep slopes, exposed summits, crests, near streams, and glacial forelands.
Fluvial-flow-dominated fans are not common within the area. However, we focused on the Dynamisk Creek alluvial fan as it is representative of fans in the broader region, thereby enabling us to study the effects of long-term climate changes on relief and plant succession in the periglacial environments. Therefore, the data presented in this paper are meant to provide a context for further analysis, the result of which will be published elsewhere.
3. Methods
3.1. UAV surveys
The study was carried out based on very high-resolution imagery acquired during a flight campaign at the end of July and beginning of August 2014 with an unmanned airborne vehicle (UAV), followed the framework proposed by CitationEwertowski, Tomczyk, Evans, Roberts, and Ewertowski (2019). We used a DJI Phantom 2 Vision+ quadcopter equipped with a 12-megapixel camera and fisheye lens. Images were acquired at an average altitude of 53 m above surface level. In total, 11 flights were performed, and 880 images were taken, covering approximately 0.8 km2. The images were taken manually with an average overlap of more than 80%. Most were NADIR images taken from several altitudes ranging from 40 to 80 m, with a much smaller subset (5%) of oblique imagery. As the number of off-NADIR imagery was much lower than 10% of an image dataset recommended by CitationCarbonneau and Dietrich (2017), we mostly relied on GCP to mitigate the doming effect. The lighting conditions were usually weak (low clouds), but the pictures were sharp as the UAV was equipped with a 3-way gimbal and the craft hovered over the place while taking each picture. Before the mission, 33 laminated black and white targets (ground control points and check points) were distributed in a regular pattern over the fan surface. Coordinates of the targets were surveyed using differential Topcon Hiper II GNSS and then post-processed.
3.2. Processing of UAV data
The images collected during the flight campaign were processed in Agisoft Photoscan using a structure-from-motion approach (cf. CitationEvans, Ewertowski, & Orton, 2016; CitationWestoby, Brasington, Glasser, Hambrey, & Reynolds, 2012). Firstly, a sparse point cloud was generated and georeferenced into the UTM 33N coordinates using twenty ground control points (GCPs). A set of further ten points served as checkpoints to verify the overall accuracy of the model. At the second step, a dense cloud of 470 million points with an average density of 715 points/m2 was created. The last step included the development of the final products: orthomosaic with 1.8 cm ground sampling distance (GSD) and digital elevation models (DEM) with 4 cm GSD. The root mean square error of the model, as measured against independent check points, was: XY error = 9 cm, Z error = 6 cm. We also checked the distribution of the absolute elevation residuals to ensure that there is no doming effect. As there was no clearly visible spatial pattern in residuals’ distribution (), we assumed that the reconstructed topography was correct, which is also confirmed by topographic profiles shown in . The DEM served as a base layer for mapping and was also used to calculate additional thematic layers (e.g. maps of slope gradient, aspect, and curvatures) and to produce hillshade models generated with different azimuth angles.
3.3. Mapping
The spatial distribution of the Dynamisk Creek alluvial fan features was identified based on analysis of the orthomosaic combined with the digital elevation model, hillshade models, as well as maps of the slope gradient, aspect, and curvatures. The mapping was verified during the geomorphological fieldwork.
Most of the mapping was done on-screen in ArcGIS software. The mapped units were established by considering the morphological character of the fan surface and interpretation of dominant geomorphological processes combined with the relative antiquity of fluvial activity and plant colonization. We took into consideration elements which have been analyzed in the literature (cf. CitationBlair & McPherson, 2009; Citationde Haas et al., 2015; CitationGómez-Villar & Garcı´a-Ruiz, 2000). Morphometric analyses were carried out in ArcMap 10.6. Morphometric fan features were extracted from DEM, based on the UAV-born data (0.04 m GSD) using the geographical information system (GIS). Moreover, we provided information about the catchment properties of the fan which were extracted from airborne DEM (5 m GSD); see CitationTomczyk and Ewertowski (2017) for details on methodology.
3.4. Map production and design
A detailed map illustrating different types of the alluvial fan’s surface morphology was produced at a scale of 1:850 (Main Map 1). We designed a map layout depicting different types of surface morphology represented as polygons. Channels, which were categorized according to the relative antiquity of their fluvial activity, were represented as polylines. Moreover, background information about the study area (such as the extent of the beach and fiord) were marked as polygons. Visualization of the shaded relief was provided. The map was digitally produced in ArcMap 10.6. The reference coordinate system was UTM zone 33N and WGS84 datum.
4. Results
4.1. Morphology of the Dynamisk Creek fan surface
This work presents the geomorphological features’ spatial patterns of the Dynamisk Creek alluvial fan located in a periglacial environment. The fan developed on the junction of the Ebbadallen valley side and fiord margin. Fluvial flows were the most important geomorphological processes responsible for aggradation of the fan surface and character of its morphology. However, the fan comprised active and inactive parts which can indicate temporal variability in the geomorphic dynamics of the fan catchment and fan surface’s depositional processes (cf. CitationGómez-Villar & Garcı´a-Ruiz, 2000). The mapped spatial units of the Dynamisk Creek fan’s geomorphology were as follows (Main Map 1, ):
-
Fresh fluvial deposits (mostly coarse gravels) comprised the active part of the fan which was characterized by fresh channel-and-bar morphology (A). It covered a relatively small area compared to the entire fan surface (11% of the fan surface, more than 39,000 m2). This unit was located along the SE axis of the fan and was separated by an escarpment (up to 2.2 m high) from the inactive northern part of the fan. The escarpment extended over a length of almost 540 m. In the apex zone, the active fluvial area was incised for about 1.65 m and was 23 m wide. At about 250 m downslope from the apex, the channel bifurcated: a branch located on the north was wide (width at up to 106 m), divided by a number of vegetated, inactive patches, and contained the active Dynamisk stream. The stream was filled with water during the summer of 2014. It was charged mostly by snow patches that preserved until late summer in sheltered depressions of the fan catchment. The second branch was narrower, with a width of up to 25 m.
-
Recently stabilized fluvial deposits were characterized by braided channels and bars partly stabilized by mosses and plants and/or partly subdued. However, they were still well visible, especially in the UAV-born images (B). This type of surface occupied almost 19,000 m2 (5% of the entire fan surface) mostly SE fragments of the fan. There had been a relatively wide (up to 73 m wide) belt of fluvial flow activity along the fan earlier before it shrank to a width of about 20 m. As a result, the activity of fluvial flow in the Dynamisk Creek fan is currently restricted mostly to one branch. Moreover, the recently stabilized fluvial deposits were also identified in a proximal part of the fan near the apex zone and in the distal part of the fan along the second branch of fresh fluvial deposits.
-
Fresh fluvial deposits on the older vegetated surface (C) consisted of relatively small patches. They were located mostly in the division zone of two branches and along the northern branch. A veneer of the fluvial deposits was relatively thin (up to 0.1 m). As a result, overburdened plants and mosses in some places survived and protruded through the deposits. In total, this unit covered more than 7000 m2 (2% of the fan surface).
-
Avalanche deposits comprised unsorted, loose sands, gravels and boulders (D) which were formed probably by slush avalanches. The location of these deposits at about 0.5 m above the bed of the Dynamisk stream suggests that these avalanches moved through the feeder channel which was filled with snow in the melting season, allowing them to flow out straight onto the inactive surface instead, along the stream direction just after crossing the apex. Deposits formed a thin debris tail with an area of more than 5000 m2 (1.5% of the entire fan surface). Avalanche deposits were identified in the inactive part of the fan covering plants and mosses; however, some of the plants survived (except those directly hit by boulders) and protruded through the deposits.
-
Niveo-aeolian deposits included multiple, very thin patches of fine-grained deposits located in the inactive part of the fan. In places, they formed characteristic dune-like shapes behind plants (E). The deposits were mostly concentrated in the proximal part of the fan and in depressions related to older vegetated channels. In total, they covered approximately 21,500 m2 (6% of the fan surface). There were also several small patches of niveo-aeolian deposits that overlapped avalanche deposits.
-
Leveled area (affected by exploratory drilling) was defined as visible traces of a vehicle which leveled and distributed deposits during mining-related (drilling) activities in the 1980s (F). It included an area of 2000 m2 (0.6% of the fan surface) located in the distal part of the fan. Moreover, the area was partly stabilized by plants and mosses.
-
Older vegetated surface was characterized by long-inactive parts of the fan (G). This area was covered by dense vegetation. It was the largest unit of the fan surface (almost 260,000 m2, 73% of the entire area). Traces of past snow avalanches, an activity of niveo-aeolian processes, linear fluvial-flows and frost weathering were also identified in these inactive parts of the fan. Small polygons of thermal-contraction-cracks and associated polygonal ground/tundra were visible mainly in the northern part of the fan.
Figure 3. A breakdown of the fan area by the character of the Dynamisk Creek fan surface morphology. Further explanation in the text.
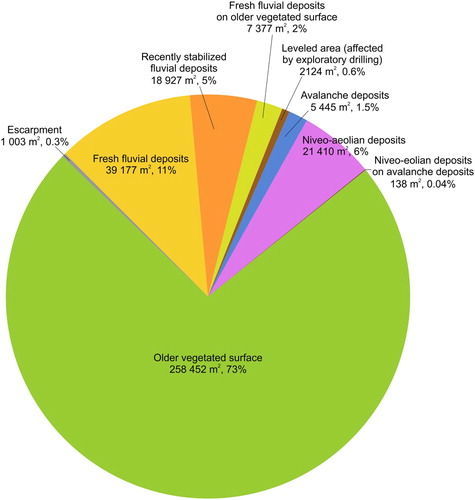
Figure 4. Character of the Dynamisk Creek fan’s surface morphology. Further explanation in the text.
Additionally, channels were significant features of the fan geomorphology. As a result, we categorized them into five types depending on the fluvial activity’s relative antiquity:
-
Active main channels (streams) were well-defined channels with low water flow, visible in the UAV-born images as well as during ground verification (H). The length of the mapped main stream was 1186 m. Discharge fluctuate during a melting season from the first half of June to the end of September (CitationLáska et al., 2012), depending on the amount of snowmelt, permafrost thaw, rainfall and development of permafrost active layer, allowing infiltration into sediments.
-
Minor active channels formed a dense braided network of about 1000 m in length. They transported water mostly during high-water peaks (spring snowmelt). During the field campaign, there was no water in the channels; however, their morphology was clearly visible in both the UAV-born images and in the field (I).
-
Recently reactivated channels comprised old channels in the inactive parts of the fan. They were mostly covered with vegetation but contained fresh traces of fluvial activities (in some cases they were also filled with water). Some of them were partly filled with fresh fluvial deposits (J).
-
Partly vegetated channels were mapped within the recently stabilized fluvial deposits. Channels were partly stabilized by mosses and plants (K). Some segments were slightly subdued, but fluvial deposits were still quite visible in the channels.
-
Traces of the old channels were recognized in the inactive parts of the fan. They were subdued, sometimes partly filled with deposits and covered with vegetation. The channels were especially visible on the hillshade model; however, it was also possible to identify them on UAV-born images and from the ground (L).
4.2. Geomorphometry of the Dynamisk Creek fan and its catchment
The Dynamisk Creek alluvial fan had an area of 0.35 km2 () with an average slope of 9o. Its length reached about 840 m while its width in profiles perpendicular to the slope was from 150 m near the apex to almost 900 m at the bottom of the fan.
Table 1. Outcomes of the Dynamisk Creek fan’s morphometric features and its catchment area. Description of the morphometric properties is based on CitationTomczyk and Ewertowski (2017).
The fan catchment, incised in the upper part of Wordiekammen ridge slopes, covered approximately 0.8 km2, and was characterized by a mean terrain slope of 29o (). The catchment relief was more than 630 m, while the dominant aspect was north-west. Such large catchment allows for concentrating large amounts of water and snow; hence fluvial flows can have a considerable geomorphic effect. According to the Geological Map of Svalbard (1:250,000) provided by the Norwegian Polar Institute (http://geodata.npolar.no), the catchment developed mostly in dolomite, sandstone, gypsum (52% of the catchment area), and carbonate rocks (42% of the catchment area).
The Dynamisk Creek fan had a concave longitudinal profile with an average slope of seven degrees. The slope in the upper part was higher than that at the bottom. All four long profiles were characterized by a similar concave shape (A), despite being located on different types of surficial units (). Profile #3 over the active part of the fan had a rough topography related to the occurrence of active channels. The three remaining profiles were surveyed over inactive parts of the fan, which results in a much smoother appearance. The bulge in profile #1 (between 500 and 650 m) suggests the existence of leftovers from the older surface – potentially it can be a remnant of a rockfall, which is supported by the occurrence of boulders in this part of the fan.
Figure 5. A – longitudinal profiles over the surface of the Dynamisk Creek fan; all profiles start from the fan apex; profile location is indicated in ; B – cross profiles over the surface of the Dynamisk Creek fan; location of the profiles is indicated in ; different types of geomorphological surfaces are indicated in different colours.
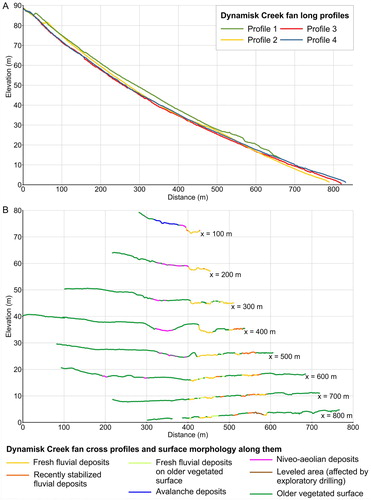
The transverse profiles were plano-convex and asymmetrical (B): the active part was in the SW side of the fan, with larger inactive areas toward the north. There was a sharp separation between the active and inactive part of the fan. The active part showed a typical braided planform. Lowering related to the present active channel was clearly visible, especially in the profiles located at 100, 200, 300, and 400 m from the apex. Some traces of older vegetated channels were still visible (profiles at 400 and 500 m from the apex) and had the same depth as the modern channels. The characteristic escarpment visible in the profiles at 400 m represented remnants of raised marine terraces. The fan was eroded at its toe by the fiord water.
5. Conclusions
5.1. UAV as a suitable tool for alluvial fan mapping
The paper presented a map at a scale of 1:850, depicting detailed geomorphology of the Dynamisk Creek fan’s surface in 2014. The primary challenge facing the study was how to map the surface of the fan with a sufficient level of details. Alluvial fans in the Arctic often have an area of less than 1 km2 (Citationde Haas et al., 2015; CitationTomczyk & Ewertowski, 2017), and their surface morphology can change on a year-to-year basis. These changes can be small but nonetheless significant from a geomorphological standpoint. We decided to use a small UAV, for when considering other alternative surveying methods, we found that traditional remote sensing data such as aerial photographs or high-resolution satellite imagery are too expensive to purchase on a regular basis and they do not offer enough spatial and temporal resolution. Moreover, topographic surveys using dGPS do not provide enough spatial coverage. Finally, laser scanning, which can offer a similar or even better level of detail than UAV surveys, is much more time-consuming and expensive (in terms of buying hardware as well as paying for such surveys).
Small, budget, and ‘ready-to-fly’ UAVs are a suitable and helpful tool for studying alluvial fans in the Arctic settings. The main advantages of using UAVs over alternative survey methods are: (1) low investments in equipment – a budget UAV with accessories costs less than 2000 USD; (2) flexibility in terms of spatial and temporal coverage. Spatial resolution depends on camera and lens characteristics as well as flying altitude; therefore ground sampling distance can vary from several mm to tens of cm. Moreover, temporal coverage can be limited only by weather conditions; (3) the quality of data is generally uniform over the whole study area; (4) orthomosaics are useful for interpretation of the geomorphological character of surfaces, whereas DEMs make it possible to quantify the geomorphological features of the fan’s surface; (5) moreover, the repetition of UAV flight campaign can enable monitoring, direct observation, and quantification of changes on the fan’s surface.
On the other hand, there are several difficulties when using UAVs to study fans in the Arctic regions, and these are mostly related to: (1) weather conditions, as windy, rainy and very cold weather is unfavorable for flying. As a result, it is recommended to plan several extra field-days in case of bad weather conditions; (2) significant differences in elevation between a fan’s apex and toe, which require changes in flight altitude and can complicate data processing; (3) local aviation regulations as they can limit or restrict UAV activities in some areas; (4) the impossibility of taking images in winter due to polar night; (5) the fact that some of the budget UAVs experience problems with magnetometer due to the high-latitude settings; (6) efficiency of batteries which can be limited by the cold temperature; (7) cloudy conditions being common which makes lighting conditions unfavorable for taking pictures (camera stabilization is required); (8) some of the Arctic sites offering only hiking-access (sometimes several hours), such that multiple batteries are necessary from a logistics standpoint.
5.2. Characteristic of the alluvial fan surface in a periglacial environment and its development over time
Fluvial activity was the most important geomorphological process responsible for aggradation of the Dynamisk Creek fan surface. Compared to debris-flow-dominated and colluvial fans, fluvial-flow-dominated fans are much fewer in numbers on Svalbard (Citationde Haas et al., 2015; CitationTomczyk & Ewertowski, 2017). It can be driven by topography, as they require enough space for developing large catchments that could feed them.
Mapping of the fan surface geomorphology from very-high resolution UAV-born images enabled us to distinguish the finer features, which provided very useful information about the geomorphological processes and their spatial patterns. The modern fan area comprised of active and inactive parts. Three-quarters of the fan surface were stabilized by vegetation. These inactive fragments of the fan were slightly influenced by secondary processes of weathering/reworking such as niveo-aeolian (6% of the fan surface) processes and snow/slush avalanches (1.5% of the fan surface). Moreover, the longest-term inactive parts were modified by the formation of small thermal-contraction-cracks. Nevertheless, the primary relief was partly preserved as some traces of the deep channels were still visible. Some of them have also been recently reactivated. A relatively small area of the fan surface (0.6%) was modified by human activities related to mining (drilling). Traces of which are still clearly visible in the landscape even if the exploration works were carried out in the 1980s. The fan surface occupied by fresh fluvial deposits covered only about 13%, including patches of the surficial units where fluvial activity expanded recently (2% of the fan surface). On the other hand, in about 5% of the fan area, fluvial processes had stopped in recent times, and these parts started being colonized by mosses and plants.
To have an insight into historical changes in the surface morphology of the fan, we used archival aerial photographs (). Time-series of images showed that in the 1960s the surficial unit, modified by active fluvial flow processes, was mainly located in the SE fragment of the fan while its spatial pattern has changed over the last fifty years. Fresh fluvial deposits moved southeast to northwest, which was probably related to a change in slope as the new material was deposited. Therefore, it illustrated how a fan surface responds to the continuous delivery of new material from a catchment. However, the total area of fluvial deposits only slightly increased (about 1.5%) during that time (). Greater variations in areal coverage were noticed in the case of snow-avalanche deposits.
Figure 6. Spatial distribution of the Dynamisk Creek fan surface morphology units in 1961, 1990, and 2014. The maps from 1961 and 1990 are based on aerial photographs provided by © Norwegian Polar Institute (http://geodata.npolar.no).
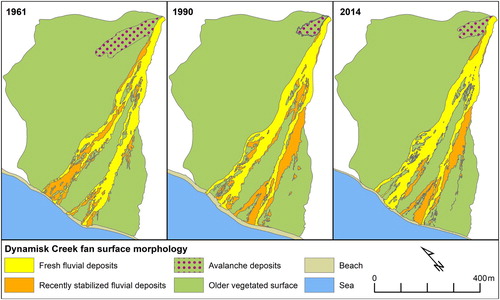
Table 2. Quantification of the Dynamisk Creek alluvial fan surface morphology from 1961, 1990, and 2014.
Surfaces of fans in periglacial environments are modified in the melting seasons (spring and summer), as there is a continuous discharge of snowmelt, permafrost thaw and rainfall from the catchment (Citationde Haas et al., 2015). Initial shape of fans was developed since the decay of last Pleistocene glaciation, following the accessibility of fresh sediments and paraglacial intensity of slope processes (CitationChurch & Ryder, 1972; CitationLønne & Nemec, 2004). Contemporarily, as the snow cover duration and its extent decrease while precipitation slightly increases in the Arctic (CitationAMAP, 2017), it might be expected that the processes operating over the fan surface will increase in the foreseeable future, which will have further implications for the safety and activity of those living nearby. Therefore, further studies on monitoring changes in the fan’s surface are necessary.
Software
UAV-born images were processed in Agisoft Photoscan Professional 1.4.2, where orthomosaic and digital elevation model was produced. ArcMap 10.6 was used to generate DEM derivatives (slope, aspect, curvature, hillshade) and analyze the morphometry of the fan and its catchment. The geomorphology of the Dynamisk Creek fan was mapped on-screen, and the final map layout was produced in ArcMap 10.6. Figures were prepared in ArcMap 10.6 and CorelDraw x7.
Main Map 1. Fluvial-flow dominated fan in central Spitsbergen, Svalbard: detailed surface morphology based on unmanned airborne vehicle (UAV) surveys
Download PDF (41 MB)Acknowledgements
Fieldwork would not have been possible without logistic support provided by AMUPS (Adam Mickiewicz University Polar Station) and members of Poznań Polar Expeditions in 2007 and 2011–2016. We are grateful to Margaret O’Donnell for proofreading the manuscript. We would like to thank the reviewers whose comments and suggestions helped to improve and clarify the manuscript and the map.
Disclosure statement
No potential conflict of interest was reported by the authors.
ORCID
Aleksandra M. Tomczyk http://orcid.org/0000-0002-5400-7131
Marek W. Ewertowski http://orcid.org/0000-0002-0422-2327
Grzegorz Rachlewicz http://orcid.org/0000-0003-1826-2079
Additional information
Funding
References
- Åkerman, H. J. (1984). Notes on talus morphology and processes in Spitsbergen. Geografiska Annaler: Series A, Physical Geography , 66 (4), 267–284. doi:10.2307/520850 doi: 10.1080/04353676.1984.11880115
- Allen, S. K. , Schneider, D. , & Owens, I. F. (2009). First approaches towards modelling glacial hazards in the mount cook region of New Zealand's Southern Alps. Natural Hazards and Earth System Sciences , 9 (2), 481–499. doi: 10.5194/nhess-9-481-2009
- AMAP . (2017). Snow, Water, Ice and Permafrost in the Arctic (SWIPA) 2017. Arctic Monitoring and Assessment Programme (AMAP). Retrieved from Oslo, Norway:.
- André, M.-F. (1995). Holocene climate fluctuations and geomorphic impact of extreme events in Svalbard. Geografiska Annaler: Series A, Physical Geography , 77 (4), 241–250. doi:10.2307/521333 doi: 10.1080/04353676.1995.11880444
- Arbellay, E. , Stoffel, M. , & Bollschweiler, M. (2010). Dendrogeomorphic reconstruction of past debris-flow activity using injured broad-leaved trees. Earth Surface Processes and Landforms , 35 (4), 399–406.
- Arfstrom, J. , & Hartmann, W. K. (2005). Martian flow features, moraine-like ridges, and gullies: Terrestrial analogs and interrelationships. Icarus , 174 (2), 321–335. doi: 10.1016/j.icarus.2004.05.026
- Ballantyne, C. K. (2002). Paraglacial geomorphology. Quaternary Science Reviews , 21 (18–19), 1935–2017. doi: 10.1016/S0277-3791(02)00005-7
- Ballantyne, C. K. , & Whittington, G. (1999). Late Holocene floodplain incision and alluvial fan formation in the central Grampian Highlands, Scotland: Chronology, environment and implications. Journal of Quaternary Science , 14 (7), 651–671. doi:10.1002/(SICI)1099-1417(199912)14:7<651::AID-JQS469>3.0.CO;2-1
- Banks, M. E. , McEwen, A. S. , Kargel, J. S. , Baker, V. R. , Strom, R. G. , Mellon, M. T. , … Jaeger, W. L. (2008). High resolution imaging science experiment (HiRISE) observations of glacial and periglacial morphologies in the circum-argyre Planitia highlands, Mars. Journal of Geophysical Research: Planets , 113 (E12), doi: 10.1029/2007JE002994
- Bernhardt, H. , Reiss, D. , Hiesinger, H. , Hauber, E. , & Johnsson, A. (2017). Debris flow recurrence periods and multi-temporal observations of colluvial fan evolution in central Spitsbergen (Svalbard). Geomorphology , 296 , 132–141. doi: 10.1016/j.geomorph.2017.08.049
- Blair, T. C. , & McPherson, J. G. (2009). Processes and forms of alluvial fans. In A.J. Parsons & A. D. Abrahams (Eds.), Geomorphology of desert environments (pp. 413–467). Dordrecht: Springer.
- Blikra, L. H. , & Nemec, W. (1998). Postglacial colluvium in western Norway: Depositional processes, facies and palaeoclimatic record. Sedimentology , 45 (5), 909–959. doi: 10.1046/j.1365-3091.1998.00200.x
- Bollschweiler, M. , & Stoffel, M. (2010). Tree rings and debris flows: Recent developments, future directions. Progress in Physical Geography: Earth and Environment , 34 (5), 625–645. doi: 10.1177/0309133310370283
- Carbonneau, P. E. , & Dietrich, J. T. (2017). Cost-effective non-metric photogrammetry from consumer-grade sUAS: Implications for direct georeferencing of structure from motion photogrammetry. Earth Surface Processes and Landforms , 42 (3), 473–486. doi: 10.1002/esp.4012
- Church, M. , & Ryder, J. M. (1972). Paraglacial sedimentation: A consideration of fluvial processes conditioned by glaciation. Geological Society of America Bulletin , 83 (10), 3059–3072. doi: 10.1130/0016-7606(1972)83[3059:PSACOF]2.0.CO;2
- Decaulne, A. , & Saemundsson, T. (2006). Geomorphic evidence for present-day snow-avalanche and debris-flow impact in the Icelandic Westfjords. Geomorphology , 80 (1), 80–93. doi: 10.1016/j.geomorph.2005.09.007
- Decaulne, A. , & Sæmundsson, Þ . (2010). Distribution and frequency of snow-avalanche debris transfer in the distal part of colluvial cones in central north Iceland. Geografiska Annaler: Series A, Physical Geography , 92 (2), 177–187. doi: 10.1111/j.1468-0459.2010.00388.x
- Decaulne, A. , Sæmundsson, Þ , & Jónsson, H. P. (2009). An overview of postglacial sediment records from colluvial accumulations in northwestern and North Iceland. Arctic, Antarctic, and Alpine Research , 41 (1), 37–47. doi: 10.1657/1523-0430-41.1.37
- Decaulne, A. , Sæmundsson, Þ , Jónsson, H. P. , & Sandberg, O. (2007). Changes in deposition on a colluvial fan during the upper Holocene in the Tindastóll mountain, Skagafjörður district, North Iceland: Preliminary results. Geografiska Annaler: Series A, Physical Geography , 89 (1), 51–63. doi: 10.1111/j.1468-0459.2007.00307.x
- de Haas, T. , Kleinhans, M. G. , Carbonneau, P. E. , Rubensdotter, L. , & Hauber, E. (2015). Surface morphology of fans in the high-arctic periglacial environment of Svalbard: Controls and processes. Earth-Science Reviews , 146 (0), 163–182. doi: 10.1016/j.earscirev.2015.04.004
- de Haas, T. , Ventra, D. , Carbonneau, P. E. , & Kleinhans, M. G. (2014). Debris-flow dominance of alluvial fans masked by runoff reworking and weathering. Geomorphology , 217 , 165–181. doi: 10.1016/j.geomorph.2014.04.028
- de Scally, F. A. , Owens, I. F. , & Louis, J. (2010). Controls on fan depositional processes in the schist ranges of the Southern Alps, New Zealand, and implications for debris-flow hazard assessment. Geomorphology , 122 (1–2), 99–116. doi: 10.1016/j.geomorph.2010.06.002
- Dühnforth, M. , Densmore, A. L. , Ivy-Ochs, S. , & Allen, P. A. (2008). Controls on sediment evacuation from glacially modified and unmodified catchments in the eastern Sierra Nevada, California. Earth Surface Processes and Landforms , 33 (10), 1602–1613. doi: 10.1002/esp.1694
- Dühnforth, M. , Densmore, A. L. , Ivy-Ochs, S. , Allen, P. A. , & Kubik, P. W. (2007). Timing and patterns of debris flow deposition on Shepherd and Symmes creek fans, Owens Valley, California, deduced from cosmogenic 10Be. Journal of Geophysical Research: Earth Surface , 112 (F3), doi: 10.1029/2006JF000562
- Engel, Z. , Česák, J. , & Escobar, V. R. (2011). Rainfall-related debris flows in Carhuacocha Valley, Cordillera Huayhuash, Peru. Landslides , 8 (3), 269–278. doi: 10.1007/s10346-011-0259-7
- Evans, D. J. A. , Ewertowski, M. W. , & Orton, C. (2016). Fláajökull (north lobe), Iceland: Active temperate piedmont lobe glacial landsystem. Journal of Maps , 12 (5), 777–789. doi: 10.1080/17445647.2015.1073185
- Evans, D. J. A. , Strzelecki, M. , Milledge, D. G. , & Orton, C. (2012). Hørbyebreen polythermal glacial landsystem, Svalbard. Journal of Maps , 8 (2), 146–156. doi: 10.1080/17445647.2012.680776
- Ewertowski, M. W. (2014). Recent transformations in the high-Arctic glacier landsystem, Ragnarbreen, Svalbard. Geografiska Annaler: Series A, Physical Geography , 96 (3), 265–285. doi: 10.1111/geoa.12049
- Ewertowski, M. W. , Evans, D. J. A. , Roberts, D. H. , & Tomczyk, A. M. (2016). Glacial geomorphology of the terrestrial margins of the tidewater glacier, Nordenskiöldbreen, Svalbard. Journal of Maps , 12 (sup1), 476–487. doi: 10.1080/17445647.2016.1192329
- Ewertowski, M. W. , Evans, D. J. , Roberts, D. H. , Tomczyk, A. M. , Ewertowski, W. , & Pleksot, K. (2019). Quantification of historical landscape change on the foreland of a receding polythermal glacier, Hørbyebreen, Svalbard. Geomorphology , 325 , 40–54. doi: 10.1016/j.geomorph.2018.09.027
- Ewertowski, M. W. , Kasprzak, L. , Szuman, I. , & Tomczyk, A. M. (2012). Controlled, ice-cored moraines: Sediments and geomorphology. An example from Ragnarbreen, Svalbard. Zeitschrift fur Geomorphologie , 56 (1), 53–74. doi: 10.1127/0372-8854/2011/0049
- Ewertowski, M. W. , Tomczyk, A. , Evans, D. , Roberts, D. , & Ewertowski, W. (2019). Operational framework for rapid, very-high resolution mapping of glacial geomorphology using low-cost unmanned aerial vehicles and structure-from-motion approach. Remote Sensing , 11 (1), 65. doi: 10.3390/rs11010065
- Ewertowski, M. W. , & Tomczyk, A. M. (2015). Quantification of the ice-cored moraines’ short-term dynamics in the high-arctic glaciers Ebbabreen and Ragnarbreen, Petuniabukta, Svalbard. Geomorphology , 234 , 211–227. doi: 10.1016/j.geomorph.2015.01.023
- Gibas, J. , Rachlewicz, G. , & Szczuciński, W. (2005). Application of DC resistivity soundings and geomorphological surveys in studies of modern Arctic glacier marginal zones, Petuniabukta, Spitsbergen. Polish Polar Research , 26 (4), 239–258.
- Glade, T. (2005). Linking debris-flow hazard assessments with geomorphology. Geomorphology , 66 (1–4), 189–213. doi: 10.1016/j.geomorph.2004.09.023
- Gonera, P. , & Kasprzak, L. (1989). The main stages of development of glacier margin morphology in the region between Billefjorden and Austfjorden, central Spitsbergen. Polish Polar Research , 10 (3), 419–427.
- Gómez-Villar, A. , & Garcı´a-Ruiz, J. M. (2000). Surface sediment characteristics and present dynamics in alluvial fans of the central Spanish Pyrenees. Geomorphology , 34 (3), 127–144. doi: 10.1016/S0169-555X(99)00116-6
- Hanáček, M. , Flašar, J. , & Nývlt, D. (2011). Sedimentary petrological characteristics of lateral and frontal moraine and proglacial glaciofluvial sediments of Bertilbreen, Central Svalbard .
- Harland, W. B. , Cutbill, J. , Friend, P. F. , Gobbett, D. J. , Holliday, D. , Maton, P. , … Wallis, R. H. (1974). The Billefjorden fault zone, Spitsbergen: The long history of a major tectonic lineament (Vol. 161) . Oslo : Norsk Polar Institute.
- Hauber, E. , Preusker, F. , Trauthan, F. , Reiss, D. , Carlsson, A. , Hiesinger, H. , … Johnsson, A. (2009). Morphometry of alluvial fans in a polar desert (Svalbard, Norway): implications for Interpreting Martian Fans. Lunar and Planetary Science XL, 1468, Abstract1658.
- Hauber, E. , Reiss, D. , Ulrich, M. , Preusker, F. , Trauthan, F. , Zanetti, M. , … Johnsson, A. (2011). Landscape evolution in Martian mid-latitude regions: Insights from analogous periglacial landforms in Svalbard. Geological Society, London, Special Publications , 356 (1), 111–131. doi: 10.1144/SP356.7
- Jahn, A. (1960). Some remarks on evolution of slopes on Spitsbergen. Zeitschrift für Geomorphologie Supplement , 1, 49–58.
- Jahn, A. (1967). Some features of mass movement on Spitsbergen slopes. Geografiska Annaler. Series A, Physical Geography , 49 (2/4), 213–225. doi: 10.2307/520889
- Jahn, A. (1976). Contemporaneous geomorphological processes in Longyeardalen, Vest-Spitsbergen (Svalbard). Biuletyn Peryglacjalny , 26 , 253–268.
- Karczewski, A. (1989). The development of the marginal zone of the Hørbyebreen, Petuniabukta, central Spitsbergen. Polish Polar Research , 10 (3), 371–377.
- Karczewski, A. , Borówka, M. , Gonera, P. , Kasprzak, L. , Kłysz, P. , Kostrzewski, A. , … Wysokiński, L. (1990). Geomorphology – Petuniabukta, Billefjorden, Spitsbergen 1:40 000. Poznań: Adam Mickiewicz University Press.
- Karczewski, A. , & Kłysz, P. (1994). Lithofacies and structural analysis of crevasse filling deposits of the Svenbreen foreland (Petuniabukta, Spitsbergen). Paper presented at the XXI Polar Symposium, Warszawa.
- Kłysz, P. (1985). Glacial forms and deposits of Ebba Glacier and its foreland (Petuniabukta region, Spitsbergen). Polish Polar Research , 6 , 283–299.
- Kłysz, P. , Lindner, L. , Marks, L. , & Wysokiński, L. (1989). Late Pleistocene and Holocene relief remodeling in the Ebbadalen-Nordenkioldbreen region in Olav V Land, central Spitsbergen. Polish Polar Research , 10 , 277–301.
- Kostrzewski, A. , Kaniecki, A. , Kapuściński, J. , Klimczak, R. , Stach, A. , & Zwoliński, Z. (1989). The dynamics and rate of denudation of glaciated and non-glaciated catchments, central Spitsbergen. Polish Polar Research , 10 (3), 317–367.
- Lamar, D. , & Douglass, D. (1995). Geology of an area astride the Billefjorden fault zone, Northern Dicksonland, Spitsbergen, Svalbard (Vol. 17) . Oslo : Norsk Polar Institute.
- Larsson, S. (1982). Geomorphological effects on the slopes of Longyear Valley, Spitsbergen, after a Heavy Rainstorm in July 1972. Geografiska Annaler. Series A, Physical Geography , 64 (3/4), 105–125. doi: 10.2307/520639
- Láska, K. , Witoszová, D. , & Prošek, P. (2012). Weather patterns of the coastal zone of Petuniabukta, central Spitsbergen in the period 2008–2010. 33 (4), 297. doi: 10.2478/v10183-012-0025-0
- Lønne, I. , & Nemec, W. (2004). High-Arctic fan delta recording deglaciation and environment disequilibrium. Sedimentology , 51 (3), 553–589. doi: 10.1111/j.1365-3091.2004.00636.x
- Małecki, J. (2016). Accelerating retreat and high-elevation thinning of glaciers in central Spitsbergen. The Cryosphere , 10 (3), 1317–1329. doi: 10.5194/tc-10-1317-2016
- Owen, G. , Hiemstra, J. F. , Matthews, J. A. , & McEwen, L. J. (2010). Landslide-glacier interaction in a neoparaglacial setting at tverrbytnede, jotunheimen, southern Norway. Geografiska Annaler: Series A, Physical Geography , 92 (4), 421–436. doi: 10.1111/j.1468-0459.2010.00405.x
- Petrakov, D. A. , Krylenko, I. V. , Chernomorets, S. S. , Tutubalina, O. V. , Krylenko, I. N. , & Shakhmina, M. S. (2007). Debris flow hazard of glacial lakes in the central Caucasus. In D. Rickenmann , & C. Chen (Eds.), Debris-flow hazards mitigation: Mechanics, prediction, and assessment (pp. 703–714). The Netherlands : Millpress.
- Przybylak, R. , & Araźny, A. (2006). Climatic conditions of the north − western part of Oscar II Land (Spitsbergen) in the period between 1975 and 2000. Polish Polar Research , 27 (2), 133–152.
- Rachlewicz, G. (2003). Meteorological conditions in Petunia Bay (central Spitsbergen) in 2000 and 2001 summer seasons. Problemy Klimatologii Polarnej , 13 , 127–138.
- Rachlewicz, G. (2009). Contemporary sediment fluxes and relief changes in high Artic Glacierized valley systems (Billefjorden, central Spitsbergen) . Poznań : Adam Mickiewicz University.
- Rachlewicz, G. (2010). Paraglacial modifications of glacial sediments over millennial to decadal time-scales in the high Arctic (Billefjorden, central Spitsbergen, Svalbard). Quaestiones Geographicae , 29 (3), 59–67. doi: 10.2478/v10117-010-0023-4
- Rachlewicz, G. , & Szczuciński, W. (2008). Changes in thermal structure of permafrost active layer in a dry polar climate, Petuniabukta, Svalbard. Polish Polar Research , 29 (3), 261–278.
- Rachlewicz, G. , Szczuciński, W. , & Ewertowski, M. W. (2007). Post−‘little ice age’ retreat rates of glaciers around Billefjorden in central Spitsbergen, Svalbard. Polish Polar Research , 28 (3), 159–186.
- Raczkowska, Z. (1990). Observations on nivation and its geomorphological effects in mountains at high latitude (with Mt. Njulla Massif in northern Sweden as example). Pirineos , 136 , 19–32. doi: 10.3989/pirineos.1990.v136.201
- Rapp, A. , & Strömquist, L. (1976). Slope erosion due to extreme rainfall in the Scandinavian Mountains. Geografiska Annaler: Series A, Physical Geography , 58 (3), 193–200. doi: 10.1080/04353676.1976.11879938
- Reiss, D. , Hauber, E. , Hiesinger, H. , Jaumann, R. , Trauthan, F. , Preusker, F. , … Johansson, L. (2011). Terrestrial gullies and debris-flow tracks on Svalbard as planetary analogs for Mars. Geological Society of America Special Papers , 483 , 165–175. doi: 10.1130/2011.2483(11)
- Rønning, O. I. (1996). The flora of Svalbard . Oslo : Norsk Polarinstitutt.
- Ryder, J. M. (1971). Some aspects of the morphometry of paraglacial alluvial fans in south-central British Columbia. Canadian Journal of Earth Sciences , 8 (10), 1252–1264. doi: 10.1139/e71-114
- Saito, K. , & Oguchi, T. (2005). Slope of alluvial fans in humid regions of Japan, Taiwan and the Philippines. Geomorphology , 70 (1), 147–162. doi: 10.1016/j.geomorph.2005.04.006
- Senderak, K. , Kondracka, M. , & Gądek, B. (2017). Talus slope evolution under the influence of glaciers with the example of slopes near the Hans Glacier, SW Spitsbergen, Norway. Geomorphology , 285 , 225–234. doi: 10.1016/j.geomorph.2017.02.023
- Stankowski, W. , Kasprzak, L. , Kostrzewski, A. , & Rygielski, W. (1989). An outline of morpho-genesis of the region between Horbyedalen and Ebbadalen, Petuniabukta, Billefjorden, central Spitsbergen. Polish Polar Research , 10 , 749–753.
- Stoffel, M. , Conus, D. , Grichting, M. A. , Lièvre, I. , & Maître, G. (2008). Unraveling the patterns of late Holocene debris-flow activity on a cone in the Swiss Alps: Chronology, environment and implications for the future. Global and Planetary Change , 60 (3–4), 222–234. doi: 10.1016/j.gloplacha.2007.03.001
- Stolz, A. , & Huggel, C. (2008). Debris flows in the Swiss National Park: The influence of different flow models and varying DEM grid size on modeling results. Landslides , 5 (3), 311–319. doi: 10.1007/s10346-008-0125-4
- Strzelecki, M. (2011). Schmidt hammer tests across a recently deglacierized rocky coastal zone in Spitsbergen – is there a “coastal amplification” of rock weathering in polar climates? 32 (3), 239. doi: 10.2478/v10183-011-0017-5
- Szuman, I. , & Kasprzak, L. (2010). Glacier ice structures influence on moraines developement (Hørbye glacier, Central Spitsbergen). 29 (1), 65. doi: 10.2478/v10117-010-0007-4
- Tomczyk, A. M. , & Ewertowski, M. W. (2017). Surface morphological types and spatial distribution of fan-shaped landforms in the periglacial high-arctic environment of central Spitsbergen, Svalbard. Journal of Maps , 13 (2), 239–251. doi: 10.1080/17445647.2017.1294543
- Westoby, M. J. , Brasington, J. , Glasser, N. F. , Hambrey, M. J. , & Reynolds, J. M. (2012). ‘Structure-from-motion’ photogrammetry: A low-cost, effective tool for geoscience applications. Geomorphology , 179 , 300–314. doi: 10.1016/j.geomorph.2012.08.021