ABSTRACT
The city of Antananarivo is located in the central highlands of Madagascar, and is the largest urban center of the island. Considering the frequent heavy cyclonic rains frequently affecting the area, its geomorphological context is particularly prone to geo-hydrological hazards, such as landslides and flash floods, as recently proved during the disastrous events of the winters of 2015 and 2018. Field data and high-resolution remote sensing data interpretation (DEMs and satellite orthophotos) were combined in order to produce detailed hydrographic and geomorphological maps. The aim was to understand the processes acting in the Analamanga hill area, with special regards to the effect of human activity in modeling the natural landforms and exacerbating the geo-hydrological hazards. The obtained maps will provide management-planning tools to be used as a first step towards a risk reduction strategy in the Antananarivo historical urban center.
1. Introduction
Antananarivo is the capital city of Madagascar and is located in the central highlands region (18.55′ South; 47.32′ East), about 160 km from the east coast and 330 km from the west coast, at approximately 1200 m a.s.l. above sea level (). Antananarivo was called Analamanga (the ‘blue forest’), until 1610, when the merina King Andrianjaka built his first wooden royal palace complex (the so-called Rova) and a fortified village on the flat hilltop of the highest relief of the area (Analamanga hill); its privileged position assured an ideal defense and observation post on the lowermost Ikopa river plane (CitationCiampalini, Frodella, Margottini, & Casagli, 2019) (). The first core of the city (the High City) further developed from the hilltop on the hillslopes (Middle town), by the late nineteenth century reaching the river valley during the colonial period (Low town). During the latter decades, the city has continued to evolve to nearly 1.7 million inhabitants today, covering an urban area of approximately 86.4 km2 (namely ‘The Great Antananarivo’, (b)).
Figure 2. Lithography and Historical pictures showing the urban development of Antananarivo (courtesy of Musee de la Photo, Antananarivo): (a) The High city in the end of the 16th century; (b) vegetation cover and rock outcrops along the Analamanga hill slopes in the end of the nineteenth century. Pictures of the modern city configuration: south-eastern slope (c) and southwestern slope (d).
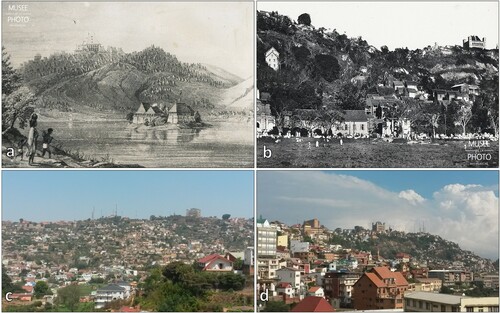
Currently, the High City represents an important cultural heritage site of Madagascar, encompassing the new stone brick-built Rova and Chapel, the high dignitaries’ buildings, such as the baroque-style architecture Andafiavaratra palace, the Cathedrals of Andohalo and Ambohipotsy, built by the first missionaries in the second half of nineteenth century (). For these reasons, the High City is currently included in the UNESCO World Heritage tentative list. Detailed thematic maps are of basic importance for the study of landslide processes (Calista et al., Citation2016; CitationFrodella, Morelli, Fidolini, Pazzi, & Fanti, 2014; CitationSmith & Ellison, 1999) and for analysing the landform evolution during the Quaternary (CitationDe Muro, Ibba, Simeone, Buosi, & Brambilla, 2017; CitationKarymbalis, Papanastassiou, Gaki-Papanastassiou, Tsanakas, & Maroukian, 2013; CitationPucci et al., 2015), with special regard to the interactions with human activity (CitationPaliaga, Luino, Turconi, & Faccini, 2018; CitationRoccati, Faccini, Luino, Ciampalini, & Turconi, 2019; CitationVisser, 2014). In this framework, the integration with remote sensing technologies can overcome the limitations of a field approach, by allowing a complete coverage of the analyzed phenomena over wide and inaccessible areas reducing costs and ensuring the safety of the field operators (CitationBardi et al., 2017; CitationCiampalini et al., 2019; CitationDel Soldato et al., 2018; CitationFrodella, Gigli, Morelli, Lombardi, & Casagli, 2017a; CitationGigli et al., 2014). The protection of Cultural Heritage from instability phenomena requires a specific inter-disciplinary approach, which should be planned considering the geological and geomorphological characteristics of the site, as well as the typology of the related hazard (CitationMargottini, Fidolini, Iadanza, Trigila, & Ubelmann, 2015a, Citation2015b; CitationNolesini, Frodella, Bianchini, & Casagli, 2016; CitationPastonchi et al., 2018). The rapid urban development of Antananarivo, together with the lack of a proper urban planning, due to political and governance issues, have caused several environmental problems, such as intense deforestation and quarrying, unauthorized slope cutting and terracing for the construction of illegal hovels, moreover, there is a general lack of proper drainage and sewer systems. This context makes the Analamanga hill area particularly prone to soil erosion, landslide and flash flooding phenomena (CitationCiampalini et al., 2019), especially during the frequent heavy cyclonic rain events affecting the central highlands (). In this context, in March 2015 Antananarivo was hit by the tropical storm Chedza, resulting in severe flooding in the river plain area of the Low city, and in widespread shallow landslides along the hillslopes of the Middle Town. This event caused diffuse structural damage affecting several quarters, also in the Upper town’s historical buildings, including 20 casualties and an estimate of 36,000 evacuees. Rock falls also occurred in the winter of 2018, affecting the buildings of the Middle town’s depressed neighborhoods, unfortunately causing several casualties. Following these events, a detailed field mapping campaign was carried out (October 2017 and May–June 2019), in order to understand the geological, geomorphological and hydrographic features of the Analamanga hill area. The surveys also enabled the identification of possible new UNESCO Core and Buffer zones. The outcomes of this activity are presented in this work by showing detailed geological and hydrographical maps, which constitute an update of the work of CitationCiampalini et al. (2019). The final purpose was to improve the geological and morphological knowledge of an area characterized by limited scientific data, in order to understand the interaction between the natural landforms, the urban evolution and the acting geomorphological processes, as the first step towards risk management and a conservation strategy of the Antananarivo historical center.
Figure 3. Antananarivo historical center quarter areas, including the possible new UNESCO Core and Buffer zones. The High City cultural heritage: (a) The Rova Palace; (b) Andafiavaratra Palace; (c) The Royal Chapel; (d) Ambohipotsy Cathedral; (e) Andohalo Cathedral.
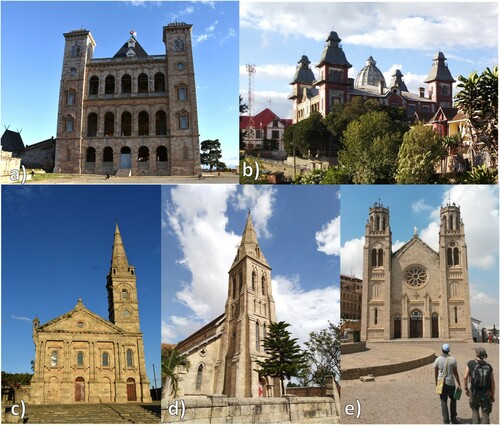
Table 1. List of tropical storms and cyclones affecting the area of Antananarivo since 1972.
1.1. Geomorphological – geological setting
The Antananarivo plateau presents a widespread surface of low relief, lying at about 1300 m.a.s.l., within the central sector of Madagascar ((b)). Its urban area develops on several low rocky hill domes and ridges dotting the alluvial plain of the Ikopa river ((b)). The landscape of the central highlands consists of high and moderate-sized hills, where the forest, which formerly covered a much greater area of Madagascar, has almost completely disappeared. From a geological point of view, the area of Antananarivo preserves a neo-Archean geological history: the Antananarivo Craton forms the basement of much of Central Madagascar and is dominated by a thick sequence of migmatites, orthogneisses, and granites that crop out in a broad north–south band across the island (CitationCollins, 2006; CitationKroener et al., 2000). Granitization occurred at various epochs (2500 million years, 1100 million years, 550 million years), producing granite intrusions in the form of batholites or dikes or, by means of granitization of some beds of the pre-Cambrian series, forming stratoid granites of variable thicknesses (from a few tens of centimeters up to 500 m or more; CitationNédélec, Ralison, Bouchez, & Grégoire, 2000). The Madagascar central basement is mantled by lateritic clays produced by chemical weathering of the crystalline bedrock; this laterisation has probably been going on since the Pliocene (CitationCox, Zentner, Rakotondrazafy, & Rasoazanamparany, 2010). Covered with a sparse vegetation of grasses, the thick, loose lateritic soils overlying the bedrock are exposed to the accelerated weathering and erosion, which produce spectacular effects on the relief: the ‘Lavakas’ (the Malagasy word for ‘hole’ with steep sides usually on the side of a hill), a typical gully erosional feature in the Central Highlands (CitationRamifehiarivo et al., 2017).
2. Methodology
The field surveys were performed on a 5.2 square kilometer by integrating traditional geological and geomorphological field surveying data with differential Real Time Kynematic GPS. The maps were prepared using a base topographic contour map 1:5000 (1:5000 scale), a post-2015 cyclone event aerial LiDAR data (1 m resolution) and a Pleiades-1A satellite orthophoto (0.5 m resolution, acquired on May 2015). The available data were homogeneized in an ESRI ArcGIS 10 environment (https://www.esri.com/en-us/home), allowing for contour, photo and digital relief visual interpretation, in order to detect morphological features related to instability phenomena (large gullies, scarps, counterscarps, hummocky topography, slope scars and bare sectors) (e.g. CitationBrardinoni, Slaymaker, & Hassan, 2003; CitationBrunsden, 1993; CitationGuzzetti et al., 2012). The complexity of the urbanization of the study area did not allow for an accurate map of all the hydrographic flow-paths; therefore, the drainage network was automatically mapped on the LiDAR data using the ArcMap 10.4.1 Hydrology Tools package (Flow Direction, Accumulation and Strem Order functions) (e.g. CitationFrodella, Morelli, & Pazzi, 2017b; CitationMargottini et al., 2015a; CitationStrahler, 1957). The geological map legend was arranged based on the granite weathering products classification of CitationLee and De Freitas (1989) and CitationDewandel, Lachassagne, Wyns, Maréchal, and Krishnamurthy (2006).
3. Results
The enclosed map shows the main features of the area and incorporates the hydrographic features (Main map on the left) and the geomorphology of the area (Main map on the right) that will be described in the following paragraphs. The adopted scale was chosen to focus on the UNESCO Buffer zone (an area that must guarantee an additional level of protection to the assets recognized as world heritage, CitationUNESCO, 1977) bounding the Analamanga hill area. The boundary of the chosen UNESCO Core zone, encompassing the High City and its cultural heritage is also shown. Other smaller maps show large and regional scale geographic information, as well as altitude at a local scale (maps a, b, c in the upper right and central section). Urban features such as the High City quarters and roads are also reported (map d in the lowermost section).
3.1. Study area geomorphological setting
The Analamanga hill is a N–S oriented rocky ridge, 3.3 km in length, rising up to 200 m above the Ikopa river alluvial plain (). From a morphologic point of view, the hilltop is represented by a flat area (1436 m.a.s.l. at its highest point), rising gradually from north and alternating southward with three saddles; two lower saddles link the Analamanga hill footslopes on the south-western and central-eastern sides to two adjacent hilltops that dot the Ikopa river plain area. The Analamanga hill southern sector is characterized by 40° slopes, where diffuse and intense quarrying activity has carved several large niches ( and ). The western hillside shows a high slope angle in its uppermost sector, due to the widespread outcropping of the granite bedrock along a sub-vertical rock wall, while the slope toe is represented by low energy surfaces gently linking with the plain area, severely affected by lavaka-like gully erosional features. On the contrary, the eastern hillside has a general minor slope angle (mean values around 30°) (CitationCiampalini et al., 2019). This slope being more exposed to the cyclones coming from the Indian Ocean, it is affected by sheet-overland flow erosion, as testified by slope scars exposing eluvial–colluvial deposits overlying the granite bedrock. Residual lateritic soils (up to a few tens of meters of maximum thickness) widely crop out at the hill footslopes, bounding the lower sector of the whole Analamanga hill, particularly in the western sector.
3.2. Hydrography
Within the study area, the complexity of the hydrographic network is due to the strong influence of urbanization (roads, bridges, buildings, anthropic channels), which modifies the natural flow-paths of the hydrographic network. Channeling and narrowing often affect the creeks, especially in their terminal stretch, where at times they experience the first culverting and then swamping (). Swamps were extensively reclaimed during the colonial period, creating artificial ponds and rice-paddies. The latter are drained by means of channels, the larger of which are located in the northern Great Antananarivo urban area, which converge into the Ikopa river main course (). According to a first field surveying approach, the general hydrographic pattern of the Analamanga hill shows radial-like features, with small creeks, deeply cutting into both the hill slopes. The performed GIS analysis allowed to map this ‘anthropic’ hydrographic network and to identify 10 small watersheds, characterized by streams up to the fifth order; their lowermost sector is delimited by the boundary of the UNESCO Buffer Zone (). The western slope is characterized by a general lower stream order of the creeks, which often form deep and narrow small valleys trending WNW-ESE, due to the linear erosion of the thick lateritic soil cover (=large gullies, or lavakas; Basins 6–7). On the contrary, the eastern slope is characterized by a more developed hydrographic network (sub-dendritic pattern-like), with higher stream order and wider basins trending NE-SW (Basins 1–4). In these catchments, sheet erosion phenomena are more frequent, as testified by frequent bare soil exposures. Generally, the creeks are present also in the dry season, thanks to the presence of springs and watercress (cressonieres), testifying the presence of perched water tables in the upper slope area. The catchments flow direction is influenced by a combination of anthropic-natural factors: (i) the two morphological lower saddles ( and ) force the network of Basins 1 and 7–10 to flow North, while the network of Basins 2–5 flows South into the large rice fields area; (ii) In the northern sector the hill morphology and the streets, the Antananarivo Stadium and ponds deeply influence the flow path direction of Basins 8-10, while on the southern sector the frequent quarry slope cuts heavily affect Basin 5.
Table 2. Features of the identified watershed.
3.3. Study area geological features
From a geological point the view the Analamanga hill is composed of a migmatitic granitoid batholite, covered discontinuously by the various products of the progressive stages of weathering of the crystalline bedrock. These latter are formed by an irregular sequence reported here, from bottom to top, following the classifications of CitationLee and De Freitas (1989) and CitationDewandel et al. (2006) ((a)):
granite fresh rock (=F), in which various fracture network develop, widely outcropping in the steep rock walls at the top of the western slopes, and in the south-eastern slope toe (in correspondence with the abandoned quarries) ((b));
laminated and slightly moderately weathered granite (saprock = SW-MW), cropping out on top of the rock walls ((b));
from highly to completely weathered granite (saprolite = HW-CW), discontinuously cropping out especially in the hilltop and on the eastern hillside ((a));
completely decomposed residual soils (laterite = RS), formed by mainly loamy sands, completely encircling both the of hillslope toes ((c)). The higher thickness of these deposits (up to a few tens of meters) is located in correspondence with the low energy surfaces at the bottom of the western slope;
loose sandy eluvial/colluvial cover (=SC), formed mainly by slightly pebbly sands, irregularly outcropping especially in the hilltop and the eastern hillside ().
Figure 6. Geological features of the study area: road cut showing an example of different granite weathering products (a); fresh granite (on the bottom) and limited granite (on the top) (b); laterite terrace slope cut for housing (c); eluvial–colluvial cover mixed with rubbish on vegetated slope (d); detail of heterogeneous slope deposits (e).
Slope deposits mantle the western and eastern slopes in fan-like shapes, and are constituted by cobbles, blocks and scattered boulders, deposited by slope gravitational processes, in a coarse sandy matrix formed by the weathering of the bedrock ((e)). Furthermore, the intense anthropic activity in the last centuries has created detrital deposits composed of heterogeneousresidual building materials, from fine to coarse-grained (anthropic deposits = AD; (d)). These may cover the above-mentioned sequence in correspondence with the inhabited areas. Alluvial deposits (=AL), formed by organic-rich clays and silts, mantle the river plain areas in correspondence with the rice-paddies.
3.4. Slope Instability processes
In this geological and geomorphological context four main groups of instability phenomena were identified, following the classification of CitationCruden and Varnes (1996), according to which the above-mentioned materials are grouped as follows: (i) rock (F-SW-MW); (ii) debris (HW-CW-AD); (iii) earth (RS):
Debris rotational/translational slides occurring in HW-CW-RS-AD ((a)). These phenomena generally occur on the hilltop and the south-eastern hillside, and are characterized by small volumes and reduced mobility (usually they accumulate at the slope toe and do not reach the hydrographic network). Nevertheless, in the case of cyclonic rain, these can evolve in unchanneled debris flows moving on the slope surface.
Earth-debris rotational/translational slides involving RS and AD. These phenomena occur along the slopes of the main creek channels deeply cutting the slope toe at the foot of the western hillside (gullies; (b)), and may evolve into channeled debris flows following intense rainfall events during the cyclones. Their related level of risk is due to the interaction with man-made structures, such as buildings located directly inside the channels, which often restrict their section, and road paving often causing their culverting in the downstream sector.
Rock fall and rock slides (planar and wedge), from metric to decametric dimension, involving the granite fresh rock (F) ((c)). These instability processes affect the steep rock walls, located both in the western hillside and at the south-eastern slope toe, and are characterized by high risk, as testified by the severe building damage and casualtiesin the March 2019 event.
Complex rotational/translational slide phenomena involving various materials such as SW-MW-HW-CW and AD ((d)). One of these phenomena, from small to medium size (1 m3 < V < 15 m3), occurred in January 2019 in correspondence with the top of the western rock wall. It evolved down the rock wall as a debris fall-avalanche: a few houses located at the foot of the rock wall were destroyed causing several casualties. Therefore, this type of phenomena is characterized by a high risk.
Figure 7. Examples of the detected instability phenomena: (a) Debris rotational/translational slides; (b); Earth-debris rotational/translational slides; (c) Rock fall and rock slides; (d) Complex rotational/translational slide.
Figure 8. Natural and Urbanized features of the study area hydrographic network: Lavakas in the western footslope (Basin 7; a); narrowing by concrete buildings (Basins 6; b); Culverting causing road pavement erosion, and garage dumping filling culvert (Basin 8; c, d); Rice fields in Basin 1 (e); general view of an artificial pond (Lac Anosy, Basin 9; f).
4. Discussion and conclusions
The morphological setting of the Analamanga hill granted a strategic commanding position above the Ikopa river valley and the surrounding highlands, favoring the development of the first fortified village around the royal palace, the ‘High City’, on its flat hilltop in the sixteenth century. The further development of Antananarivo as the Capital city of Madagascar at the end of the eighteenth century caused the beginning of the urban growth along the hillslopes, creating the ‘Middle City’. During the colonial period, this process was enhanced, reaching the river plain areas and creating the ‘Low City’, while in more recent times the urban area has sprawled covering approximately 86.4 km2 (‘Great Antananarivo’). This rapid expansion was not controlled by proper urban planning and did not take into account the geological and geomorphological processes acting on the slopes and the river plain. The steep slope sectors, the widespread weathering and fracturing of the granitoid bedrock, together with the heavy cyclonic rainfall frequently hitting the highland area, represent natural predisposing/triggering factors for geo-hydrological hazards. In this context the anthropic activity is exacerbating soil erosion, slope instabilities and flooding due to overbuilding, diffuse burning of the vegetation cover, quarry excavation and slope cutting, garbage dumping, hovels lacking proper sewer and drainage systems, narrowing, culverting and swamping of the creeks. The identification of landslide processes on the field was not an easy task, due to the rapid modification of the landforms and slope cover caused by the intense hovel urbanization. Nevertheless, the combination of field surveys and the interpretation of remote sensing data has provided the detection of landslide processes and of the hydrographic network with a satisfactory resolution from the local to a large scale. Regarding landslide hazards, the most exposed areas are the depressed southern sector of the Middle City area, particularly the western hillslope (Basins 6-7-8), where hovels have been built at the foot of rock slopes, as testified by the winter 2019 rock fall events. Debris rotational/translational slides involving the saprolithe and the overlying eluvial/colluvial cover were mapped on the south-eastern hillside, while Earth-debris rotational/translational slides involving residual soil were identified along the slopes of the main creek channels deeply cutting the slope toe at the foot of the western hillside. The Analamanga hill hydrography is characterized by a radial-like network formed by 10 small watersheds, ranging in the surface from 122 to 16 hectares, and characterized by streams up to the fifth order. Intense linear erosion of the soft lateritic soil cover creates large gullies along all the western slope toe, which are rapidly expanding and damaging the road pavement and buildings. Sheet erosion phenomena are more frequent on the eastern slope. The catchments flow direction is influenced by a combination of the natural landforms and the urban structures: regarding flood hazard, the most exposed areas are again Basins 6-7-8, where often hovel and concrete buildings are located within the large creek gullies. The produced maps represent the first step for land-use planning and a general master plan of mitigation measures for the High City and surroundings; in the near future, detailed susceptibilty mapping, slope stability analysis and accurate landslide inventory are necessary for a complete hazard assessment.
Software
ESRI ArcMap 10.4.1 was used for the data integration, the automatic hydrographic network analysis and DEM derived products (Elevation map, Hillshade, Slope), for digitizing landforms recognized by means of field surveys and DEM-ortophoto interpretation and for cartographic work. Map layout and final editing were performed using Adobe Illustrator CS5.
Analamanga_hill_Map_REV2_UPDATED.pdf
Download PDF (142.2 MB)Acknowledgements
This work was carried out in the framework of the activities of the UNESCO chair on prevention and sustainable management of geo-hydrological hazards of the University of Florence, Italy (www.unesco-geohazards.unifi.it), carried out in cooperation with the Région Île-de-France. The authors would like to thank: Paris Region Expertise-Madagascar (PRX) and in particular Tamara Teisseidre-Philip, Alexandrine Wadel, Coline Mollaret for providing all of the available topographic data, and especially kindness and support during our work; Francois Cristofoli and Filippo De Dominicis (RC Heritage/RCh consultants) for the logistic support and suggestions; Helihanta Rajaonarison of Antananarivo University for her precious knowledge and guidance, Niandr Bania Ramboason and Andria Rou’hrouh for their valuable assistance and enthusiasm.
Disclosure statement
No potential conflict of interest was reported by the author(s).
Correction Statement
This article has been republished with minor changes. These changes do not impact the academic content of the article.
References
- Bardi, F. , Raspini, F. , Frodella, W. , Lombardi, L. , Nocentini, M. , Gigli, G. , … Casagli, N. (2017). Monitoring the rapid-moving reactivation of earth flows by means of GB-InSAR: The April 2013 Capriglio landslide (northern Appennines, Italy). Remote Sensing , 9 (2), 165. doi: 10.3390/rs9020165
- Brunsden, D. (1993). Mass movement; the research frontier and beyond: A geomorphological approach. Geomorphology , 7 , 85–128. doi: 10.1016/0169-555X(93)90013-R
- Brardinoni, F. , Slaymaker, O. , & Hassan, M. A. (2003). Landslide inventory in a rugged forested watershed: A comparison between air-photo and field survey data. Geomorphology , 54 (3–4), 179–196. doi: 10.1016/S0169-555X(02)00355-0
- Calista, M. , Miccadei, E. , Pasculli, A. , Piacentini, T. , Sciarra, M. , & Sciarra, N. (2016). Geomorphological features of the Montebello sul Sangro large landslide (Abruzzo, Central Italy). Journal of Maps , 12 (5), 882–891. doi: 10.1080/17445647.2015.1095134
- Ciampalini, A. , Frodella, W. , Margottini, C. , & Casagli, N. (2019). Rapid assessment of geo-hydrological hazards in Antananarivo (Madagascar) historical centre for damage prevention. Geomatics, Natural Hazards and Risk , 10 (1), 1102–1124. doi: 10.1080/19475705.2018.1564375
- Cox, R. , Zentner, D. B. , Rakotondrazafy, A. F. M. , & Rasoazanamparany, C. F. (2010). Shakedown in Madagascar: Occurrence of lavakas (erosional gullies) associated with seismic activity. Geology , 38 (2), 179–182. doi: 10.1130/G30670.1
- Cruden, D. M. , & Varnes, D. J. (1996). Landslides: Investigation and mitigation. Chapter 3-Landslide types and processes. Transportation research board special report (247).
- Collins, A. S. (2006). Madagascar and the amalgamation of Central Gondwana. Gondwana Research , 9 (1), 3–16. doi: 10.1016/j.gr.2005.10.001
- Del Soldato, M. , Riquelme, A. , Bianchini, S. , Tomás, R. , Di Martire, D. , De Vita, P. , … Calcaterra, D. (2018). Multisource data integration to investigate one century of evolution for the Agnone landslide (Molise, Southern Italy). Landslides , 15 (11), 2113–2128. doi: 10.1007/s10346-018-1015-z
- De Muro, S. , Ibba, A. , Simeone, S. , Buosi, C. , & Brambilla, W. (2017). An integrated sea-land approach for mapping geomorphological and sedimentological features in an urban microtidal wave-dominated beach: A case study from S Sardinia, western Mediterranean. Journal of Maps , 13 (2), 822–835. doi: 10.1080/17445647.2017.1389309
- Dewandel, B. , Lachassagne, P. , Wyns, R. , Maréchal, J. C. , & Krishnamurthy, N. S. (2006). A generalized 3-D geological and hydrogeological conceptual model of granite aquifers controlled by single or multiphase weathering. Journal of Hydrology , 330 (1–2), 260–284. doi: 10.1016/j.jhydrol.2006.03.026
- Frodella, W. , Morelli, S. , Fidolini, F. , Pazzi, V. , & Fanti, R. (2014). Geomorphology of the Rotolon landslide (Veneto Region, Italy). Journal of Maps , 10 (3), 394–401. doi: 10.1080/17445647.2013.869666
- Frodella, W. , Gigli, G. , Morelli, S. , Lombardi, L. , & Casagli, N. (2017a). Landslide mapping and characterization through infrared thermography (IRT): Suggestions for a methodological approach from some case studies. Remote Sensing , 9 (12), 77–84. doi: 10.3390/rs9121281
- Frodella, W. , Morelli, S. , & Pazzi, V. (2017b). Infrared thermographic surveys for landslide mapping and characterization: The Rotolon DSGSD (Northern Italy) case study. Italian Journal of Engineering Geology and Environment , 1 , 77–84. Special Issue.
- Gigli, G. , Intrieri, E. , Lombardi, L. , Nocentini, M. , Frodella, W. , Balducci, M. , … Casagli, N. (2014). Event scenario analysis for the design of rockslide countermeasures. Journal of Mountain Science , 11 (6), 1521–1530. doi: 10.1007/s11629-014-3164-4
- Guzzetti, F. , Mondini, A. C. , Cardinali, M. , Fiorucci, F. , Santangelo, M. , & Chang, K. T. (2012). Landslide inventory maps: New tools for an old problem. Earth-Science Reviews , 112 , 42–66. doi: 10.1016/j.earscirev.2012.02.001
- Lee, S. G. , & De Freitas, M. H. (1989). A revision of the description and classification of weathered granite and its application to granites in Korea. Quarterly Journal of Engineering Geology and Hydrogeology , 22 (1), 31–48. doi: 10.1144/GSL.QJEG.1989.022.01.03
- Karymbalis, E. , Papanastassiou, D. , Gaki-Papanastassiou, K. , Tsanakas, K. , & Maroukian, H. (2013). Geomorphological study of Cephalonia Island, Ionian Sea, Western Greece. Journal of Maps , 9 (1), 121–134. doi: 10.1080/17445647.2012.758423
- Kroener, A. , Hegner, E. , Collins, A. S. , Windley, B. F. , Brewer, T. S. , Razakamanana, T. , & Pidgeon, R. T. (2000). Age and magmatic history of the Antananarivo Block, central Madagascar, as derived from zircon geochronology and Nd isotopic systematics. American Journal of Science , 300 (4), 251–288. doi: 10.2475/ajs.300.4.251
- Margottini, C. , Fidolini, F. , Iadanza, C. , Trigila, A. , & Ubelmann, Y. (2015a). The conservation of the Shar-e-Zohak archeological site (Central Afghanistan): Geomorphological processes and ecosystem-based mitigation. Geomorphology , 239 , 73–90. doi: 10.1016/j.geomorph.2014.12.047
- Margottini, C. , Antidze, N. , Corominas, J. , Crosta, G. B. , Frattini, P. , Gigli, G. , … Vacheishvili, N. (2015b). Landslide hazard, monitoring and conservation strategy for the safeguard of Vardzia Byzantine monastery complex, Georgia. Landslides , 12 (1), 193–204. doi: 10.1007/s10346-014-0548-z
- Nédélec, A. , Ralison, B. , Bouchez, J. L. , & Grégoire, V. (2000). Structure and metamorphism of the granitic basement around Antananarivo: A key to the Pan-African history of central Madagascar and its Gondwana connections. Tectonics , 19 (5), 997–1020. doi: 10.1029/2000TC900001
- Nolesini, T. , Frodella, W. , Bianchini, S. , & Casagli, N. (2016). Detecting slope and urban potential unstable areas by means of multi-platform remote sensing techniques: The Volterra (Italy) case study. Remote Sensing , 8 (9), 746. doi: 10.3390/rs8090746
- Paliaga, G. , Luino, F. , Turconi, L. , & Faccini, F. (2019). Inventory of geo-hydrological phenomena in Genova municipality (NW Italy). Journal of Maps , 15 (2), 28–37. doi: 10.1080/17445647.2018.1535454
- Pastonchi, L. , Barra, A. , Monserrat, O. , Luzi, G. , Solari, L. , & Tofani, V. (2018). Satellite data to improve the knowledge of geohazards in world heritage sites. Remote Sensing , 10 (7), 992. doi: 10.3390/rs10070992
- Pucci, S. , Villani, F. , Civico, R. , Pantosti, D. , Del Carlo, P. , Smedile, A. , … Gueli, A. (2015). Quaternary geology of the middle Aterno valley, 2009 L'Aquila earthquake area (Abruzzi Apennines, Italy). Journal of Maps , 11 (5), 689–697. doi: 10.1080/17445647.2014.927128
- Ramifehiarivo, N. , Brossard, M. , Grinand, C. , Andriamananjara, A. , Razafimbelo, T. , Rasolohery, A. , & Albrecht, A. (2017). Mapping soil organic carbon on a national scale: Towards an improved and updated map of Madagascar. Geoderma Regional , 9 , 29–38. doi: 10.1016/j.geodrs.2016.12.002
- Roccati, A. , Faccini, F. , Luino, F. , Ciampalini, A. , & Turconi, L. (2019). Heavy rainfall triggering shallow landslides: A susceptibility assessment by a GIS-approach in a Ligurian Apennine Catchment (Italy). Water , 11 (3), 605. doi: 10.3390/w11030605
- Smith, A. , & Ellison, R. A. (1999). Applied geological maps for planning and development: A review of examples from England and Wales, 1983 to 1996. Quarterly Journal of Engineering Geology and Hydrogeology , 32 (Supplement), S1–S44. doi: 10.1144/GSL.QJEG.1999.032.00S.01
- Strahler, A. N. (1957). Quantitative analysis of watershed geomorphology. Transactions, American Geophysical Union , 38 , 913–920. doi: 10.1029/TR038i006p00913
- UNESCO , 1977. Operational guidelines for the implementation of the world heritage convention. Intergovernamental committee for the protection of the world cultural heritage and natural heritage. First Session, Unesco, Paris, 27 June-1July 1977.
- Visser, F. (2014). Rapid mapping of urban development from historic Ordnance Survey maps: An application for pluvial flood risk in Worcester. Journal of Maps , 10 (2), 276–288. doi: 10.1080/17445647.2014.893847