ABSTRACT
We present a 1:50 000 scale geomorphological map of the upper Mkhomazi River basin, located in the foothills of the Drakensberg mountains in KwaZulu-Natal Province, South Africa. The sub-horizontal strata of the Permo-Triassic Beaufort Group forms plateau interfluves with a concave valley slope morphology. Locally, thick sequences of late Pleistocene colluvial deposits and associated buried paleosols (Masotcheni Formation) infill first-order tributary stream valleys and extend across the adjacent lower slopes. Surface runoff processes preferentially incise into the poorly consolidated, highly erodible sediments causing severe gully erosion that is responsible for widespread land degradation and desertification phenomena. The main purpose of this work is to derive a geomorphological map of the study area focussing on the erosional landforms to understand their spatial distribution and their relation to the colluvial deposits. Finally, a local and regional stratigraphic correlation of colluvial deposits and associated buried palaeosol profiles is proposed.
1. Introduction
In this work, we present a 1:50,000 scale geomorphological map of the upper Mkhomazi River basin in the central Drakensberg Mountains, Kwazulu-Natal, South Africa (). The area is characterized by a complex terrain morphology and is deeply incised by runoff-related soil erosion phenomena which dissect large areas of agricultural land.
The bedrock geology is composed of Permian sedimentary rocks of the Beaufort Group (Adelaide and Tarkastad Subgroups), which are sub-horizontally bedded, structurally influencing the typical deeply incised valley and high plateau terrain morphology. Other significant morphological controls are the Jurassic dolerite sills and dykes which crosscut the above-mentioned rock sequences. It was in this region that CitationKing and Fair (1944) and CitationKing (1949) associated sheet flow sediment transport and gully formation with long-term erosion, parallel slope retreat and pediment formation. However, they did not describe the detritus or transported waste that commonly overlies the cut bedrock pediment surface in this region. The association of episodic accumulation of poorly sorted sediment through the episodic accumulation of colluvial deposits on hillslopes in the Middleveld region of Swaziland was described by CitationPrice Williams et al. (1982) who noted the association of archaeological artefacts that was deposited during colluviation and the subsequent phases of soil development during hillslope stabilization. Colluvium is defined as poorly sorted sediment comprising an admixture of clay-, silt-, sand- and gravel sized particles that is eroded from upper slopes and deposited on lower hillslopes or pediments by sheet-wash processes (CitationWatson et al., 1984). These hillslope cover deposits occur in a wide range of terrain morphological and bedrock contexts across parts of Southern Africa.
In the KwaZulu-Natal study area of South Africa these colluvial deposits are grouped as the Masotcheni Formation, a lithostratigraphic grouping of sheetwash and gully channel colluvial and buried palaeosols that represent several cycles of colluvium deposition, pedogenesis and erosion during the Quaternary (CitationBotha, 1996; CitationBotha et al., 1990, Citation1994; CitationTemme et al., 2008). The geological map of the Drakensberg foothills study area (CitationGeological Survey, 1981) depicts only a simplistic, incomplete distribution of the colluvial deposits that delineates the sediments exposed by deep erosion gullies (dongas) rather than the broader deposits associated with infilled bedrock depressions that extend over wide areas between interfluves. In this study we present a more accurate and detailed assessment of the spatial distribution of the Masotcheni Formation in the upper Mkhomazi River basin based on field measurements, remote sensing, and geophysical surveys. The topographical range and lithological characteristics of the dispersive and highly erodible colluvium/palaeosol pediment cover deposits (i.e. fine clay and clay loam deposits on gentle slope (∼5°)), support rill-interrill erosion ((a)) and gully erosion phenomena ((b)). Gully erosion is the removal of soil by concentrated turbulent water flow through enlargement of rills and channels mainly due to intermittent stream flow after heavy rainfall events (e.g. CitationPoesen, 1996; CitationSidorchuk et al., 2003). Gully erosion represents a geo-environmental problem and a serious land degradation issue in many parts of the world causing soil loss and sediment mobilization (e.g. CitationConforti et al., 2011, Citation2014; CitationKropáček et al., 2016; CitationPelacani et al., 2009; CitationSidorchuk, 1999; CitationSidorchuk et al., 2003; CitationZakerinejad et al., 2018). In their study of the physio-chemical properties of the colluvial sediments and buried palaeosols typical of the Masotcheni Formation, CitationCama et al., 2020, CitationRienks et al. (2000) found that the vulnerability of the colluvium/palaeosols to gully erosion was related to the variable dispersivity and erodibility properties of the material, subsurface piping and turbulent surface runoff hydrology.
Figure 2. Main lithotypes and principal soil erosion processes in the study area. (a) Rill-interrill erosion. (b) Masotcheni Formation in a gully outcrop. (c) Adelaide Subgroup interbedded mudstone and fine sandstone. (d) Lithological boundary between Tarkastad Sandstone (beneath) and intrusive dolerite sill (above) displaying a spheroidal weathering profile. (e) Top of the Masotcheni colluvial profile showing a typical Solonetz polygonal structure. (f) Shallow landslide.
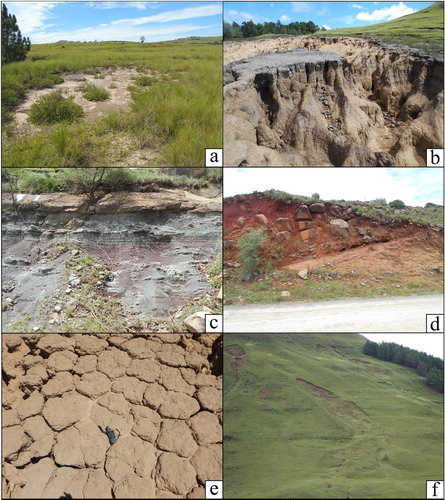
The geomorphological mapdepicts four principal landforms; (i) fluvial and runoff associated landforms, (ii) slope landforms and deposits, (iii) litho-structural landforms, and (iv) anthropogenic structures. The landforms were mapped through field survey, orthophotos and Google Earth image analyses. Furthermore, gully forms and features have been characterized from a geological point of view using 20 stratigraphic columns of 14 well exposed and accessible gullies. A detailed terrain analysis based on a 12 m DEM allowed for a quantitative morphometric description of the gully systems. The map covers a regional area of about 500 km2 and yields insights into the spatial distribution and lithostratigraphic nature of the colluvial deposits, highlighting the vulnerability of the area in terms of gully and rill-interrill erosion phenomena.
Moreover, the colluvial deposits of southern Africa are also relevant since the time elapsed between phases of colluviation permitted archaeological material to accumulate both during colluviation and the subsequent phases of stabilization and soil formation (CitationPrice Williams et al., 1982). The archaeological value of the Middle Stone Age (MSA) artefacts in the Masotcheni Formation is due to the occurrences in ‘open sites' rather than the confines of cave deposits where MSA are usually excavated. The luminescence dated colluvial succession where Early- and Middle Stone Age stone artefacts were described by CitationBotha (1996) also represents a much longer late Pleistocene temporal framework (∼130,000 years) than that preserved in most cave deposits. At the Sibudu Shelter near the coast in KwaZulu-Natal, CitationJacobs et al. (2008) correlated three periods of late Pleistocene hillslope colluviation in the region with periods when MSA inhabitants abandoned the shelter whereas the intervening periods bracketing palaeosol formation (CitationBotha & Partridge, 2000) correlate with periods of occupation at Sibudu.
Finally, a detailed knowledge of the geomorphological processes and related forms allows for better land use management especially in the context of subsistence agriculture as practiced in the area today.
2. Geological and geomorphological setting
2.1. Geological setting
The study area () covers about 500 km2 and is dominated by a mountainous landscape. The elevation ranges between 2267 m a.s.l. at the peak of the Drakensberg Mountains to ca. 1055 m a.s.l. at the Mkhomazi River. The region is characterized by a Subtropical Highland climate (Cwb), following the Köppen climate classification (CitationKottek et al., 2006), with significant precipitation of about 920 mm/year based on the precipitation time series from 1970 to 2005 (CitationNel & Sumner, 2008). From the base, the upper part of the Karoo Supergroup sedimentary succession exposed in the study area is represented by the Permo-Triassic Beaufort Group (CitationRutherford et al., 2015; CitationTurner, 1981), specifically by the Adelaide Subgroup ((c)) and the upper Tarkastad Subgroup ((d)) (CitationCatuneanu & Elango, 2001; CitationNorman & Whitfield, 2006; CitationSmith et al., 1993). The Karoo Supergroup succession is intruded by dolerite sills and dykes (CitationLyons et al., 2013) associated with the igneous event that produced the Drakensberg basaltic lavas. A long period of erosion and valley incision followed Gondwana breakup during the late Cretaceous and the study area is now covered by widespread, thin veneer of late Pleistocene colluvial deposits represented by the Masotcheni Formation. Pleistocene colluvial mantles occur in northern and central KwaZulu-Natal, the eastern Free State and some areas in central Swaziland, Botswana and Zimbabwe (CitationBotha, 1996; CitationBotha et al., 2016; CitationWatson et al., 1984). Although similar colluvial palaeosol successions are widespread north and west of the Maluti mountains in the eastern Free State province and Lesotho, these occurrences are in a different ‘geomorphic province' context (CitationPartridge et al., 2010) to the Masotcheni Formation type area which extends across the Ladysmith Basin, South-eastern Coastal Hinterland and Queenstown Basin geomorphic provinces of central KwaZulu-Natal and the Eastern Cape province.
The Masotcheni Formation colluvial deposits are commonly concentrated where the hillslope morphology forces overland flow and sheetwash transported sediments to accumulate in bedrock depressions or colluvial hollows (e.g. CitationDietrich & Dorn, 1984; CitationMills, 1987; CitationReneau et al., 1989) and multiple episodes of gully cut-and-fill have been documented in this region (CitationBotha, 1996).
The Masotcheni Formation includes fine clay, silty and sandy, poorly sorted, stratified colluvial sediments (CitationBotha & Fedoroff, 1995; CitationLyons et al., 2013; CitationTemme et al., 2008; CitationWatson et al., 1984) ((b)) generated by the erosion of weathered regolith and soils from upslope on the Drakensberg foothill interfluve ridges and deposited along their lower slopes (CitationBotha et al., 2016). In many areas, the Masotcheni Formation is eroded by recently forming gullies, locally named ‘dongas' (CitationLyons et al., 2013). Following the FAO soil Group Classification (CitationIUSS Working Group WRB, 2014) the paleosols are classified as Solonetz and are characterized by silty-clay soils with a high-concentration of sodic clay in a Btn horizon. Moreover, some these paleosols are characterized by typical columnar, prismatic shaped peds with a polygonal structure on top of the Btn-horizon ((b, e)). The colluvial deposits were deposited under semi-arid conditions (CitationWatson et al., 1984), followed by long periods of hillslope stability represented by paleosol profiles (CitationBotha, 1996). Concentration of runoff towards the colluviums-filled bedrock depressions on middle and lower hillslopes results in preferential erosion of the colluvium/paleosol succession which incorporates some dispersive and highly erodible sediment (CitationRienks et al., 2000).
2.2. Geomorphological setting
The steep, high relief topography is structurally controlled by the sub-horizontal strata of the Tarkastad and Adelaide Subgroups, and intrusive dolerite sills, leading to a classic plateau morphology (). The dolerite intrusions mainly occur as sills and dykes that dominate the landscape due to their relative resistance to weathering and the related selective erosion processes. Severe weathering processes cause a typical ‘onion skin’ spheroidal weathering of dolerite deposits and a characteristic oxidation of soils giving a red colour to the latter ((d)). The grassland and patches of sparse shrubby vegetation, combined with the lithological and topographic characteristics of the area as well as intense overland flow runoff processes, lead to widespread rill-interrill and gully processes and their related forms.
In the study area, the formation of gullies is also influenced by the presence of man-made contour terraces, where gullies can develop due to breaching of the contour banks by the concentration of surface runoff. Gully erosion exposes thick sequences of interbedded colluvial sediments and associated paleosols that define the stratigraphy of the Masotcheni Formation.
The Mkhomazi and Mkhomazana rivers represent the most important rivers of the area investigated. Long term erosion of the river system has preserved fluvial terraces at levels of up to 60 m above the current riverbed. These river terraces form flat surfaces that represent paleo-floodplains and valley bottoms. River terrace deposits are constituted by heterogeneous pebbles scattered in a fine red matrix. Shallow rotational and translational landslides and rockfall deposits occur on the steep slopes below the plateau margins ((f)).
3. Material and methods
The geomorphological forms and features were delineated, and the geomorphological map was generated following the methodology reported in .
3.1. Geomorphological mapping and map assemblage
The geomorphological map was generated following several working steps: (i) detailed field survey in 2017/2018/2019, (ii) analysis of available orthophotos (2009–1: 10 000 scale) and Digital Elevation Model, and (iii) GIS based map compilation.
The field survey was conducted based on a 1:50,000 topographic map 2929DA Himeville (CitationNational Geo-Spatial Information, 2016). Once the principal landforms were mapped and features were checked, we validated the identified forms and features using a set of orthophotos provided by the Chief Directorate: CitationNational Geo-Spatial Information, 2009 as well as Google Earth images (© 2016 Google). The field mapping supported by aerial photo interpretation (API) was enhanced by a detailed terrain analysis using a DEM with 12 m resolution, from the TanDEM-X satellite platform provided by Deutsches Zentrum für Luft- und Raumfahrt (DLR). The elevation model is an innovative spaceborne radar interferometer that is based on two TerraSAR-X radar satellites flying in close formation (CitationKrieger et al., 2007). The terrain analysis was performed using SAGA GIS version 7.6.2 (CitationConrad et al., 2015). A set of different topographic indices () were calculated following CitationWilson and Gallant (2000); CitationZakerinejad and Maerker (2014); and CitationBachofer et al. (2015). The topographic indices facilitate the geomorphological interpretation of landforms and features (CitationBosino et al., 2019; CitationMaerker et al., 2019). Initially, the DEM was corrected to remove sinks using the algorithm proposed by CitationWang and Liu (2006) and subsequently we derived the indices represented in .
Table 1. Topographic indices used in this study.
Furthermore, we integrated our field investigation with the existing gully inventory map provided by the Department of Environmental Affairs, Republic of South Africa, available at http://carbon-atlas.dirisa.org/download-data. The assembled database includes 279 gullies and 133 forms of sheet erosion that were digitized from Google Earth images (2016) and orthophotos (2009).
Each landform mapped in the field was digitized using QGIS (version 3.2) with a unique symbol following the Italian guidelines for geomorphological cartography (after CitationCampobasso et al., 2018). The symbols representing the processes, forms and features were differentiated into tectonic (brown), litho-structural (ochre), slope landforms and deposits (red), fluvial and runoff associated forms (green), and anthropogenic (black). The geological base map was derived from the lithostratigraphic subdivision of the existing 1:250 000 geological series map, 2928 Drakensberg (CitationGeological Survey, 1981). The geological boundaries were digitized using QGIS and the colluvial deposits of the Masotcheni Formation partially mapped with DGPS and field surveys. The lithotypes present in the map were grouped on the basis of geomorphological characteristics and on their attitude in relation to degradationfeatures (CitationCampobasso et al., 2018). The principal lithotypes are: (i) Sandstone and Quaternary deposits (yellow colour) represented by fluvial deposits (FVL) and by the Masotcheni Formation (Qm), (ii) Intrusive rocks (bordeaux colour) represented by the dolerite dykes and sills (Jd) that intrude the bedrock formations, (iii) the Elliot Formation (grey colour) is characterized by red mudstone (Tre), and (iv) interstratified rocks consisting of alternating sandstone, shale and mudstone (light blue colour) representing the Tarkastad Subgroup (TRt) where sandstone and maroon coloured mudstone are the dominant lithotype. Finally, in blue colour the Adelaide Subgroup (Pa) in which the mudstone is the dominant lithotype. Additionally, three geological sections are depicted on the map to highlight the general stratigraphy and the main terrain morphology of the area.
3.2. Description of landforms and features
In the upper Mkhomazi River basin the principal geomorphological processes are related to the fluvial channel, as well as surface and subsurface erosion processes. Runoff from upslope and the sides of colluvial depressions is concentrated, causing severe erosion on the weakly consolidated colluvial deposits/paleosols that mantle the footslopes. Consequently, rill-interrill and gully erosion landforms and features are widespread in the area (see Main map). During the field investigation we conducted a sedimentological, pedological and stratigraphic description of the colluvial deposits/paleosols exposed by the gully erosion. In order to correlate the thickness and the stratigraphic distribution of the colluvial deposits and associated paleosols at a local and regional scale, we mapped and described profiles of 14 well exposed and accessible gully systems (see Main map). In each a detailed stratigraphic section was measured and the thickness, lithology, colour, and structure of the paleosols were described (see section 4.1). Furthermore, five morphometric indices derived from the DEM (elevation, slope, aspect, general curvature, and vertical distance to channel network) were statistically assessed and plotted using the software R (CitationR Development Core Team, 2008). The indices were classified as follows; (i) the slopes ranging between 0° and 90° were split into six classes, (ii) aspect ranging between 0° to 360° was divided into eight classes, (iii) the general curvature which ranges between −0.5 and +0.5 was divided into three classes (−0.5 to −0.002 concave slopes, −0.002 to 0.0002 plan slopes, and 0.0002–0.5 convex slopes), (iv) the elevation ranging between 1000 m and 1600 m was split into six classes, and (v) the vertical distance to channel network which was divided into fourteen classes from 0 to 600 m.
The other fluvial and runoff associated features occurring in fluvial channels as well as raised fluvial terrace remnants were mapped during the field survey with the support of Google Earth imagery and orthophoto maps.
River terrace deposits were identified and mapped on the field from 2017 to 2019 in the Mkhomazi and Mkhomazana basins ((a)). A vertical distance to channel network was applied to extract the river terrace surfaces using a 5th order stream network. The QGIS plugin, QProf, was used to draw several river terrace profiles in order to correlate the river terrace deposits at the regional scale.
Figure 5. Field evidence. (a) River terrace deposits; level 4 overlying Tarkastad Subgroup bedrock. (b) Man-made contour terraces in abandoned ploughed agricultural fields where gullies have developed.
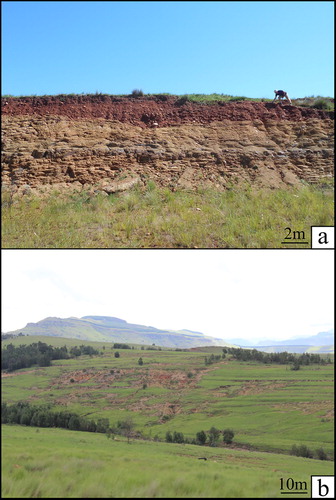
The study area is also characterized by dryland agricultural fields where contour terraces have been created to control and divert runoff from the ploughed lands. Poor maintenance of the contour banks often results in the development of rill-interrill and gully erosion ((b)). Fluvial and runoff landforms and features were mapped using a green colour. The gravitational mass movement landforms were symbolized in red polygons. The principal river systems, drainages and lakes were mapped from the orthophotos as polygonal shapes and are shown in blue.
The anthropogenic terrain forms are depicted using black lines. Quarries, landfill sites and terraced slopes were identified with vector polygons and the villages with a point symbol. Roads were mapped with white lines. Archeological areas and the locations of the stratigraphic columns were also reported in the map, with black point dots.
4. Geomorphological map
The landforms were identified, grouped, and described as follows: (i) Fluvial and runoff associated landforms, (ii) Slope landforms and deposit, (iii) Litho-structural landforms, and (iv) Anthropogenic forms.
4.1. Fluvial and runoff associated landforms
The fluvial landforms include river terraces, abandoned meanders (oxbow lakes), fluvial saddles, V-shaped valleys and crevasse channels.
The Mkhomazi and Mkhomazana rivers are characterized by incised meandering channels. During the field survey several raised river terrace deposits were identified. In order to extract the paleo-river terrace surfaces preserved in the Mkhomazi River basin we applied the ‘Vertical Distance to Channel Network’ module on a 12 m DEM. The identification of five terrace levels confirms the long-term incision by the river. The ‘level 0’ is the oldest terrace at a height of about 40 m above the present channel, the ‘level 1’ terrace is elevated 35-38 m, the ‘level 2’ terrace at 22-25 m, the ‘level 3’ terrace occurs at a height of about 18 m, and the ‘level 4’ terrace is the most recently incised, situated at a height of 5 m above the present day river bed. The profiles were extracted with QProf, where the elevation scale was exaggerated in order to better visualize the height variations.
Along the rivers the riverbanks are stable and vegetated. Furthermore, along the slopes, several V-shaped valleys were mapped. From the orthophoto analysis, flood crevasse channels and abandoned meanders were also identified in the area.
Finally, the resulting map depicts the rill-interrill and gully erosion features that were observed on both data sources (2016 Google Earth and 2009 orthophotos).
In the study area, gullies develop preferentially in the fine colluvial sediments and buried palaeosols of the Masotcheni Formation. The gully incision is up to 9 m deep and the bedrock and the overlying colluvial deposits can be readily distinguished. Gullies cover an area of 3.9 km2 while rill-interrill erosion forms and sheet erosion features cover an area of just 1.3 km2, for a total area of 5.2 km2. Even if the area covered by the erosional features results small respect to the extent of the study area, these landforms manifest on the gentle slopes which are often used for agriculture purposes causing severe loss in agricultural and grazing land.
(a) shows a general and complete vertical section of a gully system (KwaThunzi gully) located in the Mkhomazi basin. In general, the Btn horizon is characterized by clay-loam soil and the A horizon is depicted by clay soil ((b)). The burial of a sequence of paleosols is the result of successive periods of landscape stability (pedogenesis), followed by instability (erosion) and sediment accretion (gully infill) ((c)). Btn 5 and A5 horizon were dated using Optically Stimulated Luminescence (OSL), a method used to estimate the burial ages of sediments (CitationAdamiec et al., 2008). Preliminary OSL dating results ((c)) indicate a the accumulation of this colluvium occurred at 66.62 ± 7.68 ka and 39.42 ± 5.27 ka which brackets the earliest colluvial deposition in the Okhombe valley which is in a similar geological and terrain morphological context towards the northwest (CitationTemme et al., 2008). The Mkhomazi area dates do not include the earliest colluvial accretion and erosion events dated by CitationBotha et al. (1994) and CitationBotha (1996) in the Thukela basin to the northeast. Interestingly, these dates overlap a period during Oxygen Isotope Stage 3 when cooler and drier conditions prevailed at Sibudu shelter (CitationJacobs et al., 2008) when widespread erosion in the catchments made occupation of the region inhospitable to Middle Stone Age culture.
Figure 6. (a) General vertical section of the Masotcheni Formation. (b) Grain size analysis following the CitationSoil Classification Working Group (1991). (c) Optically Stimulated Luminescence (OSL) dated units (ka) shown relative to the cyclical erosion/colluviation/pedogenesis schematic model that represents the sequence of colluvial sediments and associated paleosols (after CitationBotha, 1996).
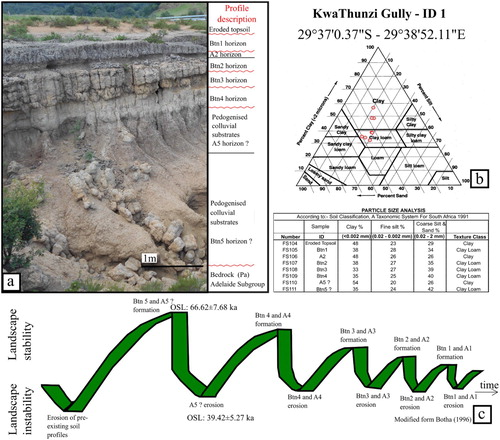
Btn5 horizon is clay-rich with thin beds of angular sandstone pebbles and does not show the typical columnar/prismatic structures of a solonetz soil. According to the Munsell soil chart the colour of this layer can be described as 10YR7/6. A5 horizon (2.5Y5/2) is rich in clayey components and includes sub-angular granule-sized siltstone clasts.
The following Btn4 is dominated by greenish clay (5YR8/2), silt and rounded sand grains. Btn3 horizon is dominated by clays (10YR8/1) and is characterized by fine, black material, which might be traces of organic matter. Btn 2 horizon and A1 horizon consist of brown clay and clay-loam, which includes spherical brown oxide particles. According to the Munsell colour charts, Btn 2 has a colour of 10YR7/2, A1 has a colour of 10YR6/1.
The highest part of the succession is the current soil profile in which Btn1 horizon is characterized by a columnar structure and colour 7.5YR6/1. The topsoil is not present, having been eroded by surface runoff.
Twenty stratigraphic profiles were measured in 14 gully sidewalls in order to spatially correlate the Masotcheni paleosol horizons in the study area (). As highlighted in the most consolidated Btn5 horizon is always preserved and its thickness varies between 40 and 300 cm. Btn4 horizon ranges from brown or reddish colour depending on the influence of weathered dolerite components in the colluvial sediments. Other Btn and A layers are widely eroded in the area and are characterized by a range of thickness. This is due to the footslope inclination that leads to a differential erosion of the colluvial layers.
Figure 7. Spatial correlation of colluvial deposits. Stratigraphic columns in the Mkhomazi and Mkhomazana River basins: Pa: Adelaide Subgroup. FVL: fluvial deposits. Btn5, Btn4, Btn3, Btn2, Btn1: Bt horizon of Solonez soils. A5, A3, A2: A horizon of Solonez soils. Act: Actual soil, Solonetz.
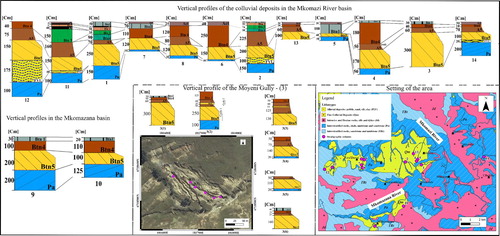
In order to characterize the gullies of the study area from a morphometric point of view we took into consideration the five morphometric indices derived from the 12 m DEM. The mean value from the point cloud of each gully was calculated and plotted in R where 88% are in an elevation range between 1200 m and 1400 m altitude ((a)).
Figure 8. Morphometric indices calculated as mean value from the point cloud of each gully. (a) Elevation. (b) Slope. (c) Aspect. (d) General Curvature. (e) Vertical Distance to Channel Network.
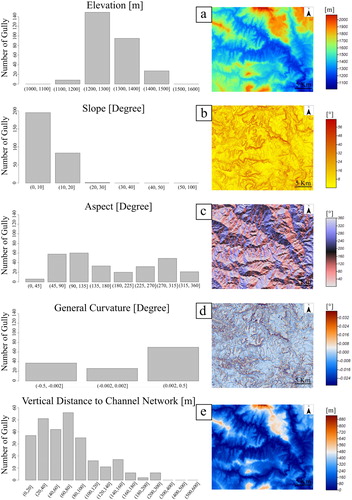
Furthermore, 69% of gullies occur within the slope classes between 0o and 10° and the remaining 31% between 10o and 30° ((b)). Gullies are mainly exposed on slopes with an easterly and northwesterly aspect (0–135° and 270° - 315° respectively) ((c)) and are predominantly convex slopes (from 0.0002 to 0.5) ((d)). Gullies occur mainly at elevations between 0 m and 100 m above the river channel network ((e)).
4.2. Slope landforms and deposits
The area steep upper hillslopes are presently stable and not affected by large landslides. However, localized, shallow landslides were identified in the field ((f)), caused by detachment of the upper soil cover, creating shallow depressions that concentrate runoff. The sub-horizontal attitude of the well-bedded Tarkastad Subgroup bedrock associated with steep slopes and high relief leads also to widespread rockfall debris below steep slope segments in the study area. Small rockfalls and topples occur in parts of the gully systems ((a)).
4.3. Litho-structural landforms
The litho-structural forms mapped include dykes, plateaus, structural scarps, ridges and saddles. The principal litho-structural landforms are directly related to the characteristics of the weathered Adelaide and Tarkastad Subgroups bedrock strata, i.e. alternating sandstone/mudrock beds, thickness of resistant strata as well as the sub-horizontal attitude of the bedding. Selective erosion acts on the friable clayey strata, resulting in undermining of the low scarps associated with the overlying, more resistant sandstone beds, forming the typical plateau topography.
The bedrock sedimentary succession is traversed by sub-vertical dolerite dykes which can be easily recognized in the field and from orthophoto analysis due to their positive weathering. The dolerite dykes define a preferential NW-SE aligned trend and are usually not covered by colluvial deposits.
4.4. Anthropogenic forms
In the study area the rural community is located in widespread homestead clusters and a few small villages. Dryland crop agriculture and cattle breeding are the principal sources of income in the area. Consequently, the principal evidence of human-induced landscape modifications is represented by agricultural contour bank terrace systems ((b)). These terraces in ploughed lands follow the contour and were established in the late 1950s (CitationBotha, 1996) as a means of reducing rill erosion from crop lands. These structures are seldom adequately maintained and thus encourage soil erosion which starts with rill-interrill erosion and can develop into gully erosion features where contour banks are breached. Gully systems are often used as landfill or dumping area in the region. Finally, in the upper Mkhomazi basin there are small dams that have been constructed for irrigation purposes.
5. Conclusions
We present a 1:50 000 scale geomorphological map of the upper Mkhomazi basin, KwaZulu-Natal, South Africa. Thick sequences of fine colluvial deposits on gentle slopes have been preferentially eroded by surface runoff phenomena, yielding rill-interrill and gully erosion landforms and features. The colluvial deposits, previously only partly mapped and inadequately represented on geological maps in the study area (CitationGeological Survey, 1981), were characterized and analysed in detail through an extensive field survey. A consistent sequence of buried, partially truncated paleosol horizons was identified. The Btn5-horizon is always preserved in the area, whereas the younger Btn and A horizons are more or less eroded. The high silt and clay component of the paleosols often includes sodic smectitic clay that imparts dispersive soil characteristics that enhance the highly erodible nature of the pedogenically altered colluvial hillslope cover deposits. High erosion rates and formation of rill and gully erosion reduce soil fertility and strip soil cover from agricultural fields. Soil erosion leads to the loss of agricultural productivity, environmental degradation and structural damage to buildings and road/bridge infrastructure. The geomorphological map represents an accurate inventory of erosional landforms, especially including gully and rill-interrill features and constitutes the basis for a quantitative assessment of erosion susceptibility in the upper Mkhomazi basin and can be used to gauge expansion of erosion features in the future.
Software
The maps were generated and realized using open source GIS (QGIS 3.0). The DEM was derived with SAGA GIS version 7.6.1. The stratigraphic columns were compiled with the free software Inkscape.
Main Map.pdf
Download PDF (61.3 MB)Acknowledgements
The authors are grateful to the Council for Geoscience office in Pietermaritzburg for logistical support. We thank the DLR and the TDX Science Team for providing Tandem-X data set of the study area.
Disclosure statement
No potential conflict of interest was reported by the authors.
Additional information
Funding
References
- Adamiec, G., Bailey, R. M., Wang, X. L., & Wintle, A. G. (2008). The mechanism of thermally transferred optically stimulated luminescence in quartz. Journal of Physics D-Applied Physics, 41(13), 135503. https://doi.org/10.1088/0022-3727/41/13/135503
- Bachofer, F., Quénéhervé, G., Hochschild, V., & Märker, M. (2015). Multisensorial topsoil mapping in the Semiarid Lake Manyara Region, Northern Tanzania. Remote Sensing, 7(8), 9563–9586. https://doi.org/10.3390/rs70809563
- Bosino, A., Omran, A., & Maerker, M. (2019). Identification, characterisation and analysis of the Oltrepo Pavese calanchi in the Northern Apennines (Italy). Geomorphology, 340, 53–66. https://doi.org/10.1016/j.geomorph.2019.05.003
- Botha, G. A. (1996). The geology and paleopedology of late Quaternary colluvial sediments in northern KwaZulu-Natal. Memoir, 83, Geological Survey of South Africa. 165 pp.
- Botha, G. A., De Villiers, J. M., & Vogel, J. C. (1990). Cyclicity of erosion, colluvial sedimentation and paleosol formation in Quaternary hillslope deposition from northern Natal, South Africa. Palaeoecology Africa, 21, 195–210.
- Botha, G. A., & Fedoroff, N. (1995). Palaeosols in late Quaternary colluvium, northern KwaZulu-Natal, South Africa. Journal of African Earth Science, and the Middle East, 21(2), 291–311. https://doi.org/10.1016/0899-5362(95)00072-2
- Botha, G. A., & Partridge, T. C. (2000). Colluvial deposits. In T. C. Partridge, & R. R. Maud (Eds.), The Cenozoic of Southern Africa (pp. 88–99). Oxford University Press.
- Botha, G. A., Temme, A. J. A. M., & Singh, R. G. (2016). Colluvial deposits and slope instability. In J. Knight & S. Grab (Eds.), Quaternary environmental change in Southern Africa: Physical and human dimensions (pp. 137–152). Cambridge University Press. doi:10.1017/CBO9781107295483.009.
- Botha, G. A., Wintle, A. G., & Vogel, J. C. (1994). Episodic Late Quarternary palaeogully erosion in northern Kwazulu/Natal, South Africa. Catena, 23(3-4), 327–340. https://doi.org/10.1016/0341-8162(94)90076-0
- Cama, M., Schillaci, C., Kropáček, J., Hochschild, V., Bosino, A., & Maerker M. (2020). A probabilistic assessment of soil erosion susceptibility in a head catchment of the Jemma Basin, Ethiopian Highlands. Geosciences 10(7), 248. http://doi.org/10.3390/geosciences10070248
- Campobasso, C., Carton, A., Chelli, A., D’orefice, M., Dramis, F., Graciotti, R., Guida, D., Pambianchi, G., Peduto, F., & Pellegrini, L. (2018). Carta Geomorfologica d’Italia –1:50.000. Aggiornamento ed integrazioni delle linee guida della Carta Geomorfologica d’Italia alla scala 1:50.000. Roma. Quaderni serie III Volume 13 Fascicolo I. Servizio Geologico d’Italia – ISPRA; Associazione Italiana Di Geografia Fisica E Geomorfologia – AIGEO, Consiglio Nazionale Dei Geologi – CNG.
- Catuneanu, O., & Elango, H. N. (2001). Tectonic control on fluvial styles: The Balfour Formation of the Karoo Basin, South Africa. Sedimentary Geology, 140(3-4), 291–313. https://doi.org/10.1016/S0037-0738(00)00190-1
- Conforti, M., Aucelli, P. P., Robustelli, G., & Scarciglia, F. (2011). Geomorphology and GIS analysis for mapping gully erosion susceptibility in the Turbolo stream catchment (Northern Calabria, Italy). Natural Hazard, 56(3), 881–898. https://doi.org/10.1007/s11069-010-9598-2
- Conoscenti, C., Angileri, S., Cappadonia, C., Rotigliano, E., Agnesi, V., & Maerker, M. (2014). Gully erosion susceptibility assessment by means of GIS-based logistic regression: A case of Sicily (Italy). Geomorphology, 204, 399–411. https://doi.org/10.1016/j.geomorph.2013.08.021
- Conrad, O., Bechtel, B., Bock, M., Dietrich, H., Fischer, E., Gerlitz, L., & Böhner, J. (2015). System for automated geoscientific analyses (SAGA) v. 2.1.4. Geoscientific Model Development Discussions, 8(2), 2271–2312. https://doi.org/10.5194/gmdd-8-2271-2015
- Dietrich, W. E., & Dorn, R. (1984). Significance of thick deposits of colluvium on hillslope: A case study involving the use of pol lin analysis in the coastal mountains of northern California. Journal of Geology, 92(2), 147–158. https://doi.org/10.1086/628845
- Geological Survey. (1981). 1:250.000 geological series map. 2928 Drakensberg. Department of Minerals and Energy.
- IUSS Working Group WRB. (2014). World reference base for soil resources 2014, update 2015 International soil classification system for naming soils and creating legends for soil maps. World Soil Resources Reports No. 106. Rome: FAO.
- Jacobs, Z., Wintle, A. G., Duller, G. A. T., Roberts, R. G., & Wadley, L. (2008). New ages for the post-Howiesons Poort, late and final Middle Stone Age at Sibudu, South Africa. Journal of Archaeological Science, 35(7), 1790–1807. https://doi.org/10.1016/j.jas.2007.11.028
- King, L. (1949). The pediment landform: Some current problems. Geological Magazine, 86(4), 245–250. https://doi.org/10.1017/S0016756800074665
- King, L. C., & Fair, T. J. D. (1944). Hillslopes and dongas. Transactions of the Geological Society of South Africa, XLVII, 2–4.
- Kottek, M., Grieser, F., Beck, C., Rudolf, B., & Rubel, F. (2006). World Map of the Köppen-Geiger climate classification updated. Meteorologische Zeitschrift, 15(3), 259–263. https://doi.org/10.1127/0941-2948/2006/0130
- Krieger, G., Moreira, A., Fiedler, H., Hanjnesk, I., Werner, M., Younis, M., & Zink, M. (2007). TanDEM-X: A satellite formation for high-resolution SAR interferometry. IEEE Transactions on Geoscience and Remote Sensing, 45(11), 3317–3341. https://doi.org/10.1109/TGRS.2007.900693
- Kropáček, J., Schillaci, C., Salvini, R., & Märker, M. (2016). Assessment of gully erosion in the Upper Awash, Central Ethiopian highlands based on a comparison of archived aerial photographs and very high resolution satellite images. Geograria Fisica e Dinamamica Quaternaria, 39, 161–170. http://doi.org/10.4461/GFDQ.2016.39.15
- Lyons, R., Tooth, S., & Duller, G. A. T. (2013). Chronology and controls of donga (gully) formation in the upper Blood River catchment, KwaZulu-Natal, South Africa: Evidence for a climatic driver of erosion. The Holocene, 23(12), 1875–1887. https://doi.org/10.1177/0959683613508157
- Maerker, M., Schillaci, C., Melis, R. T., Kropáček, J., Bosino, A., Vilímek, V., Hochschild, V., Sommer, C., Altamura, F., & Mussi, M. (2019). Geomorphological processes, forms and features in the surroundings of the Melka Kunture Palaeolithic site, Ethiopia. Journal of Maps, 15(2), 797–806. https://doi.org/10.1080/17445647.2019.1669497
- Mills, H. H. (1987). Variation in sedimentary properties of colluvium as a function of topographic setting, valley and ridge province. Virginia. Zeitschrift fur Geomorphologie, 31(3), 277–292.
- National Geo-Spatial Information. (2016). 2929DA Himeville. 1:50000 topocadastral map.
- Nel, W., & Sumner, P. (2008). Rainfall and temperature attributes on the Lesotho-Drakensberg escarpment edge, Southern Africa. Geografiska Annaler, 90(1), 97–108. https://doi.org/10.1111/j.1468-0459.2008.00337.x
- Norman, N., & Whitfield, G. (2006). Geological journeys. A traveller’s guide to South Africa’s rocks and landforms. Struik Nature.
- Partridge, T. C., Dollar, E. S. J., Moolman, J., & Dollar, L. H. (2010). The geomorphic provinces of South Africa, Lesotho and Swaziland: A physiographic subdivision for earth and environmental scientists. Transactions of the Royal Society of South Africa, 65(1), 1–47. https://doi.org/10.1080/00359191003652033
- Pelacani, S., Maerker, M., & Rodolfi, G. (2009). Modelling the potential impact of groundwater dynamics on gully erosion and drainage basin evolution. In D. M. Ferrari, & A. R. Guiseppi (Eds.), Geomorphology and plate tectonics (pp. 1–18). Nova Science Publishers.
- Poesen, J. W. (1996). Contribution of gully erosion to sediment production on cultivated lands and rangelands. IAHS, 236, 251–266.
- Price Williams, D., Watson, A., & Goudie, A. S. (1982). Quarternary colluvial stratigraphy. Archaeological sequences and palaeonvironmental in Swaziland, southern KwaZulu-Natal, South Africa. Catena, 39, 11–31.
- R Development Core Team. (2008). R: A language and environment for statistical computing. R Foundation for Statistical Computing. ISBN 3-900051-07-0, URL http://www.R-project.org
- Reneau, S. L., Dietrich, W. E., Rubin, M., Donahue, D. J., & Jull, A. J. T. (1989). Analysis of hillslope erosion rate using dated colluvial deposits. Journal of Geology, 97(1), 45–63. https://doi.org/10.1086/629280
- Rienks, S. M., Botha, G. A., & Hughes, J. C. (2000). Some physical and chemical properties of sediments exposed in a gully (donga) in northern KwaZulu-Natal, South Africa and their relationship to the erodibility of the colluvial layers. Catena, 39(1), 11–31. https://doi.org/10.1016/S0341-8162(99)00082-X
- Rutherford, B. S., Rubidge, B. S., & Hancox, P. J. (2015). Sedimentology and palaeontology of the Beufort Group in the Free State Province support a reciprocal foreland basin model for the Karoo Supergroup, South Africa. South African Journal of Geology, 118(4), 355–372. https://doi.org/10.2113/gssajg.118.4.355
- Sidorchuk, A. (1999). Dynamic and static models of gully erosion. Catena, 37(3-4), 401–414. https://doi.org/10.1016/S0341-8162(99)00029-6
- Sidorchuk, A., Maerker, M., Moretti, S., & Rodolfi, G. (2003). Gully erosion modelling and landscape response in the Mbuluzi River catchment of Swaziland. Catena, 50(2-4), 507–525. https://doi.org/10.1016/S0341-8162(02)00123-6
- Smith, R. M. H., Erickson, P. A., & Botha, W. J. (1993). A review of the stratigraphy and sedimentary environments of the Karoo-aged basins of Southern Africa. Journal of African Earth Sciences, 16(1), 143–169. https://doi.org/10.1016/0899-5362(93)90164-L
- Soil Classification Working Group. (1991). Soil classification – A taxonomic system for South Africa. Department of Agricultural Development.
- Temme, A. J. A. M., Baartman, J. E. M., Botha, G. A., Veldkamp, A., Jongmans, A. G., & Wallinga, J. (2008). Climate control on late Pleistocene landscape evolution of the Okhombe valley, KwaZulu-natal, South Africa. Geomorphology, 99(1-4), 280–295. https://doi.org/10.1016/j.geomorph.2007.11.006
- Turner, B. R. (1981). Revised stratigraphy of the Beaufort Group in the Southern Karoo Basin. Paleontologia Africana, 24, 87–98.
- Wang, L., & Liu, H. (2006). An efficient method for identifying and filling surface depressions in digital elevation models for hydrologic analysis and modelling. International Journal of Geographical Information Science, 20(2), 193–213. https://doi.org/10.1080/13658810500433453
- Watson, A., Price Williams, D., & Goudie, A. S. (1984). The paleoenvironmental interpretation of colluvial sediments and paleosols of the late Pleistocene hypotermal in South Africa. Paleogeography, Paleoclimatology, Paleoecology, 45(3-4), 225–249. https://doi.org/10.1016/0031-0182(84)90008-7
- Wilson, J. P., & Gallant, J. C. (2000). Digital terrain analysis. In J. P. Wilson, & J. C. Gallant (Eds.), Terrain analysis: Principles and applications (pp. 1–27). J. Wiley.
- Zakerinejad, R., & Maerker, M. (2014). Prediction of gully erosion susceptibilities using detailed terrain analysis and maximum entropy modeling: A case study in the Mazayejan plain, southwest Iran. Geografia Fisica e Dinamica Quaternaria, 37(1), 67–76.
- Zakerinejad, R., Omran, A., Hochschild, V., & Maerker, M. (2018). Assessment of gully erosion in relation to lithology in the southwestern Zagros mountains, Iran using aster data, gis and stochastic modeling. Geografia Fisica e Dinamica Quaternaria, 41, 95–104. http://doi.org/10.4461/GFDQ.2018.41.15
- Zevenbergen, L. W., & Thorne, C. R. (1987). Quantitative analysis of land surface topography. Earth Surface Processes and Landforms, 12(1), 47–56. https://doi.org/10.1002/esp.3290120107