ABSTRACT
This paper presents a new geomorphological map for the lower Hushe Valley (below 3400 m asl), located to the SE of the Central Karakoram in Baltistan (North Pakistan). Fieldwork and remote sensing were combined to improve understanding of the most recent surface landforms to produce a 1:50,000 scale map. Thirteen landform types associated with glacial, fluvial, gravitational and mass wasting processes were identified and mapped. Particular emphasis was made on currently dynamic processes that could pose a threat to the population. The distribution of the landforms on the valley (reworked tills, alluvial fans, rockfalls, among others) differs between the eastern and the western hillslopes, and from north to south, mainly due to bedrock types, location of geological structures and distribution of lateral tributaries. This map is the first and necessary step towards a deep assessment on geological risk related to external processes in the area.
1. Introduction
The Central Karakoram is one of the most rapidly rising areas on Earth (rates of uplift are estimated to be between 2 and 6 mm y−1), with complex topography and extreme relief, providing one of the best natural laboratories to study complex landscape evolution (CitationLeland et al., 1998; CitationRex et al., 1988). Feedback mechanisms between climate, surface processes and tectonics have been proposed to explain landforms in the Central Karakoram (CitationAhmed & Rogers, 2014). However, according to some studies, surface processes are the dominant events that govern landscape evolution during interglacial times (CitationShroder et al., 2011). Debuttressing of slopes due to glacier retreat may have prepared the slopes for failure (CitationBallantyne, 2002; CitationHewitt et al., 2011). As a result, ubiquitous mass movements have transported and transport material from steep slopes to valley bottoms while glaciofluvial meltwater redistributes sediment down the valley (CitationSeong et al., 2009).
The present study is part of a development cooperation project to increase the resilience of the Hushe Valley inhabitants (Central Karakoram) to the hydro-geomorphological processes that pose a threat. With this in mind, our research focused on identifying and mapping the landforms of the lower Hushe Valley (). The complex geological setting makes it essential to combine the preliminary analysis of satellite and aerial imagery with thorough fieldwork in order to map the most recent surface landforms. The map presented here provides a basis for future geochronological assessment that would complement those carried out in neighbouring valleys (CitationHewitt, 1999; CitationOwen, 1989; CitationSeong et al., 2009) and for a refined reconstruction of past processes that are being conducted using sedimentological analysis. As far as we know, this is the first detailed geomorphological map for the lower Hushe Valley including the main landforms.
Figure 1. (a) Location of Hushe valley. (b) Elevation map of the Hushe River basin, showing the river and its main tributaries and sub-basins, villages, glaciers and highest peaks. (c) Longitudinal profile of the Hushe River (A-A’ in b) with the location of the villages. (d) Simplified geological map of the basin with the main geological structures and lithological complexes (modified from CitationPalin et al., 2012).
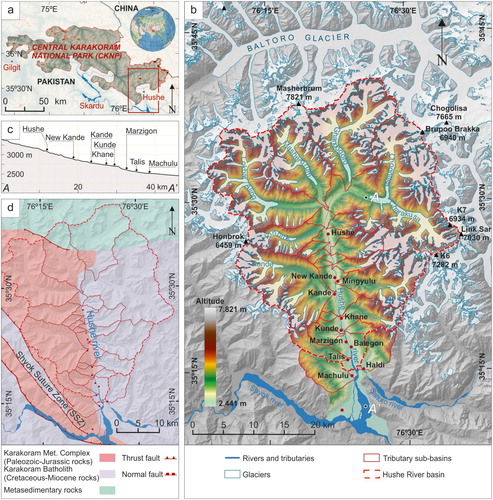
2. Study area
The Hushe River basin is located to the SE of the Central Karakoram National Park (CKNP) in Pakistan ((a)), south of the Baltoro glacier and high mountains such as Masherbrum (7821 m). It is a north–south oriented valley with an area of 1230 km2 and elevations between 2441 and 7821 m ((b)). The area exhibits a complex topography and a complicated interaction among erosion, transport and sedimentation processes involving glaciers, slopes and rivers, which produce a wide variety of landforms.
The thickening of the crust, the rise of the continent and its erosion have produced in the area, beyond an extreme topography, abundant exposures of the deep crust of the Asian plate (CitationSearle et al., 1989). Geologically, the valley is located between the Karakoram Batholith to the north-east and the Shyok Suture Zone (SSZ) or the Main Karakoram Thrust (MKT) to the south-west (CitationPalin et al., 2012). The Hushe River course is mainly located at the contact between materials of different nature: in the east mainly plutonic materials integrated within the Karakoram Batholith (Cretaceous-Miocene rocks) and in the west rocks from the Karakoram Metamorphic Complex, (Paleozoic-Jurassic rocks) that mainly consist of gneisses, metapelites and marbles (CitationRolland et al., 2006) ((d)), which increase in metamorphic degree towards the north.
The effect of the high elevation together with the precipitation's altitudinal variation results in zoning of geomorphological processes in the region (CitationHewitt, 2005): the lower zone, below 3000 m asl, dominated by fluvial processes comprises deep gorges and valley bottoms where most of the areas are covered by glaciofluvial and gravitational hillside sediments (CitationSeong et al., 2009); the middle zone, between 3000 and 6000 m asl, dominated by glacial surfaces and/or sediments and mountain ridges; and, the upper zone extending above 6000 m asl, covered by perennial ice and snow, and comprising the highest peaks and ridges in the region. The Main Map produced focuses on the lower Hushe Valley (below 3400 m asl), an area where the human population, civil infrastructure and main cultivation areas are located. A significant part of the external geological processes concentrates in this area, although their origin is mostly triggered in the upper-middle zone.
The Hushe River, with a length of 42.4 km, runs from east to west at the head of the valley, where it receives the meltwaters from the Chogolisa-Charakusa and Ghondogoro glaciers ((b)). Then, after receiving the tributaries of Masherbrum and Lokpar/Aling glaciers, it runs north to south towards its confluence with the Saltoro River, near Machulu, and the Shyok River (Indus basin) near Saling (Main Map), both coming from the east side. At this point where the three rivers converge, an extensive funnel-shaped deposition area is formed, accumulating a large part of the materials eroded and transported by the Hushe River (mainly silt, sand and gravel). Here, the river shows a braided morphology, with a well-developed floodplain that extends towards the margins from Kande to Saling, with notable narrowings around Khane and Kunde. In the northern part, around Hushe village, the river shows a similar morphology.
The tributaries of the Hushe flow transversely to the main river ((b)). The streams coming from the east show, in general, narrower valleys than those from the west, most probably due to geological causes. Kande is one of the tributaries with the largest catchment area (122.4 km2) and shows a significant glacier area at its headwater. Most of the lateral tributaries show glaciers at their headwaters, except Kunde, with a relatively small catchment area, and Machulu. These differences, among others, have a great control over the hydrological dynamics and processes of these lateral valleys which imply a certain danger for the inhabitants located downstream. Traditionally, the inhabitants of the valley have settled on flat areas near the river, so most of the 12 villages in the Hushe Valley, including Haldi, were built on the alluvial fans at the exit of the lateral valleys, such as Khane, Talis and Kande ((b)).
3. Methodology
Landform mapping was achieved through satellite image interpretation and field mapping. The map is presented at 1:50,000 scale using the WGS 1984 UTM-Zone 43N coordinate system. Landforms were digitised in QGIS (http://qgis.org) using a combination of 10 m resolution Sentinel-2 images, 3–5 m resolution PlanetScope/RapidEye (CitationPlanet Team, 2017), and ∼1 m resolution GoogleSatellite and DigitalGlobe/GeoEye-1 images available through the QGIS ‘QuickMapServices’ plugin (). Areas of poor image quality were examined in GoogleEarth™ software (v7.3) which also offers high-resolution SPOT 6/7 and DigitalGlobe images for the study area. Both relief-shaded (315° and 45° azimuth) and slope gradient-shaded models were constructed using a mosaic of Radiometric Terrain-Corrected (RTC) ALOS PALSAR Global Radar Imagery (12.5 m resolution) from the VERTEX (https://vertex.daac.asf.alaska.edu/) repository, primarily to provide topographic context. Additionally, the composite mosaic of the 8 m resolution HMA (High Mountain Asia) DEM (Digital Elevation Model) derived from high-resolution optical imagery (CitationShean, 2017) has been used to improve the mapping quality and landform identification. Contemporary glaciers were identified using the most current version 6.0 of the Randolph Glacier Inventory (CitationRGI Consortium, 2017), which was manually corrected to update the outlines to the 2019 extensions.
Figure 2. Illustrative image of the joint process of interpretation and mapping of the landforms of the lower Hushe valley from satellite images and fieldwork. (a) PlanetScope satellite image mosaic from July 12, 2018. (b) Relief-hillshade composite of ALOS PALSAR and HMA Digital Elevation Models (DEMs). (c) Notes, schemes and illustrations made during fieldwork. (d) Interpretation and manual drawing of landforms on GoogleSatellite imagery. (e) Extract of the final map in the Kunde area.
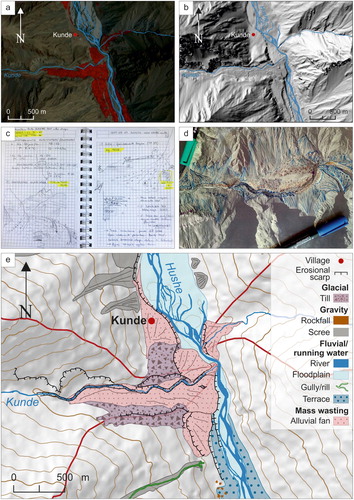
Field work was conducted in two campaigns allowing a cross-check of features mapped from remote imagery. During October 2018, a first geomorphological description of the entire lower Hushe Valley was made, from the south (Saling, 2490 m asl) to the north (near Ghondogoro glacier, 3410 m asl). During September 2019, previously recognised landforms were characterised by their internal structure and classified according to the geological processes, with particular emphasis on currently dynamic ones. The field description of lithofacies has been made using notations of genetic facies models to establish the link between the deposit and the physics of the depositional process. We constructed stratigraphic columns, established the lateral variations of facies and applied the principles of vertical superposition as a base for reconstructing depositional environments and build the map. This work was fundamental for a correct mapping of landforms because the concatenation of different deposition and erosion processes in such an active area modify the original shapes of deposits making the remote interpretation of forms very difficult.
4. Landforms and geomorphological processes
Thirteen main landform types were recorded on the geomorphological map of the lower Hushe Valley (Main Map), which are summarised in . In this section, we briefly describe the erosion and deposition landforms grouped by their genesis.
Table 1. Summary of landforms mapped in this study classified according to the geomorphological process type. Erosional scarps are related to the other mentioned processes.
4.1. Associated with glacial processes
Geomorphological evidence indicates that the Karakoram region has undergone at least four major glaciations including, at least, six glacial advances throughout the Late Quaternary (CitationSeong et al., 2009). However, in areas such as this, where there is active tectonics and seasonal changes are strong, the records of glacial and paraglacial formations are often eroded and/or hidden by more recent processes (CitationSeong et al., 2009). In the lower Hushe Valley, erosive glacial landforms are rarely observed. However, in the southern half of the valley, the original U-shaped cross-sectional valley-form is preserved although modified by fluvial incision ((a)), which causes the erosive forms of the former glacial valley to be hanging. In the Machulu area, the possible base of the glacial depression is located about 200 m above the main riverbed and triangular-shaped cliffs can be observed in some areas above a shoulder located around 700–1000 m from the main channel (at an altitude of 3200–3400 m asl) ((b)). The hanging lateral valleys that could have been generated by the action of glaciers, have been eroded by the river forming narrow gorges at the present day (e.g. the Mingyulu and Khane Valleys) ((c)).
Figure 3. (a) View looking north-west from south of Haldi showing U-shaped of the previous glacier valley eroded by the Saltoro River. (b) Shoulders (partially marked by the yellow line) related to the previous glacial erosion forms, view looking east from Kande (Main Map). (c) View looking east of Mingyulu looking hanging lateral valley generated by the glacial dynamic and later eroded by the river. (d) Detail of a till-type deposit located south of Hushe village (Main Map). (e) Kande tributary sub-basin and the location of the glacial lake (Main Map) on a Sentinel-2 satellite image, 20 September 2019. (f) Close-up view of the glacial lake on a PlanetScope satellite image, 12 July 2018 (CitationPlanet Team, 2017). View for the location of the villages mentioned.
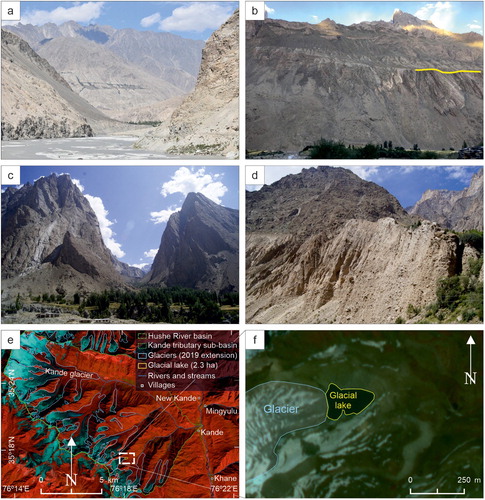
No glacial depositional landforms (e.g. moraines) could be distinguished in the lower elevations of the valley. Some large glacial boulders emerge between the river terrace deposits around Talis and Machulu and towards the north from Kande, however they are located in secondary positions, related to fluvial incision processes or to subsequently eroded alluvial fans. For that reason, in order not to cause erroneous interpretations they have not been mapped. The deposits that most resemble ancient moraines, are the tills that appear to the south of the Hushe village and also in the areas of Kunde and Talis (Main Map) at 700–1000 m above the bottom of the main valley (from 3200 to 3500 m asl and around 4000 m asl). Most of those tills ((d)) are reworked and eroded by gravity processes and incorporated within alluvial fans (described in subsection 4.4) or scree deposits (described in subsection 4.3). Therefore, it is difficult to determine their former extent, and the resulting morphology is subtle and discontinuous. They are more frequent on the western hillside, while on the eastern side the erosive forms, as shoulders ((b)), predominate at similar heights (Main Map).
In the Hushe River basin, there are 154 contemporary glaciers covering a total area of ∼401.7 km2 in 2019, approximately one third (32.7%) of the entire valley area. This area represents ∼11% of the glacier surface of the entire CKNP (total area in 2010 from CitationSenese et al., 2018). The compound basin of Chogolisa-Charakusa hosts the largest glacier in the valley with a surface area of 75.2 km2 and a length of 16.7 km. It is followed by Ghondogoro (68 km2), Lokpar/Aling (49.8 km2), and Masherbrum (32.3 km2) glaciers, all of them located in the headwater of the Hushe River. The mean glacier terminus elevation is found to be about 4470 m asl., although the debris-covered ablation tongues of the main glaciers reach down to 3500 m asl. Some of these glaciers have shown surging events during the last decades, such as Ghondogoro between 1990–2000 and Lokpar/Aling between 1989–1997 (CitationBhambri et al., 2017). Additionally, several small meltwater ponds are distributed on top of these debris-covered ablation tongues, which could be taken as an initial stage for a supraglacial lake expansion process (CitationSakai, 2012), and eventually lead to a smaller magnitude outburst flood due to the sudden release of glacier meltwater (CitationWatson et al., 2017). In relation to that, although glacial lakes are frequent in the CKNP (CitationSenese et al., 2018), only few small-size lateral moraine-dammed lakes have been mapped and one proglacial lake of significant size (2.3 ha) has been identified in the Hushe River basin today. This is a lake impounded by the terminal moraine derived from glacial retreat of a second order glacier (64 ha in area and 1.4 km in length) in the Kande sub-basin ((e,f)).
4.2. Associated with fluvial or running water processes
The fluvial regime is mainly influenced by glacier meltwater showing large diurnal and seasonal variations and contributing a significant amount of water during summer. In its 42.4 km length, the Hushe River shows an average slope of 0.2%. Along its profile, in its middle section, there are at least 3 well-marked knickpoints: between Hushe and New Kande, after Kunde and just before Talis ((c)). In most of its profile, the current main course of the river incises into previous deposits, a surprising fact in such active orogen (CitationHewitt et al., 2011; CitationMiller, 1984). However, in the southern part, near Machulu, some incision into bedrock has been observed, where a higher rate of incision can be attributed to the proximity of the Shyok Suture Zone (CitationSeong et al., 2008) ((d)).
To the north of Hushe village, the main river shows nested fill terraces ((a)) within previously deposited fluvial sediments. Downstream the river terraces are erosional in origin, cut-in-fill terraces ((b)), downcutting in previously deposited sediments of a different nature (CitationEasterbrook, 1993; El CitationOshebi et al., 2017). From the current floodplain of the Hushe River ((d)), it is possible to identify at least three levels of cut-in-fill terraces along almost the entire valley profile. In the southern half of the valley (downstream from Khane) the river terraces are not so well preserved due, in part, to anthropogenic actions (terracing of the land for cultivation, roads, etc.) or to reworking by geological processes. The deposits of these terraces are made up of gravel and subrounded boulders and blocks with a medium to low grain size sorting ((c)). The sandy-loam fraction is scarce since it has been washed out by water.
Figure 4. (a) The Hushe River inciding in nested fill river terrace deposits, north of Hushe village. (b) Cut-in-fill terraces in the up-western part of the valley, south of Hushe village; three levels of terraces are indicated by the yellow lines. (c) Detail of Hushe River terrace deposits in the west side of the road from New Kande to Hushe. (d) View looking north of the current floodplain of the Hushe River around Marzigon (indicated by the white arrow).
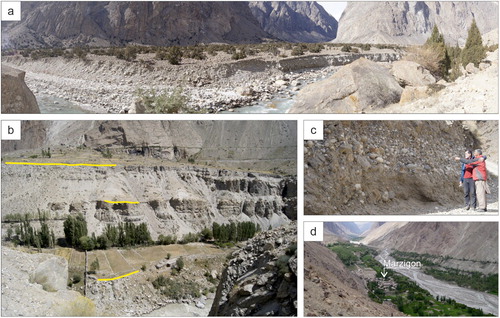
The Hushe River is fed by 19 main lateral tributary rivers, 12 from the west and 7 from the east ((b)). The streams have steep slopes and high energy showing alluvial fans dominated by cohesive or open texture sediment deposits at their outlets. Those alluvial fans (subsection 4.4) reach the Hushe River, creating a certain degree of narrowing or the complete closure of the main channel and interrupting its fluvial landforms, causing: (a) the expansion by agradation of the fluvial load deposition upstream the narrowing of the river (Main Map); or (b) the creation of a lake upstream of the closure of the main channel.
This river damming has happened at several points in the valley causing the formation of paleolakes, as in some other Karakoram valleys (CitationAhmed et al., 2018; CitationHewitt, 1998; CitationOwen, 1988). The most significant sedimentary record of this type of lacustrine deposits in the valley can be found around Hushe village ((a,b); Main Map) and is characterised by an absolute predominance of fine facies (sands and silts), with good sorting and parallel or cross lamination. The mapping of those sediments outlines a paleolake of at least 4 km in length, 1.5 km in width and around 100–140 m thick. Other smaller deposits of lake sediments are located on the eastern hillside of the valley, in front of the alluvial fan of Kunde, near Kande, and between Talis and Balegon ((c)) covered by other types of sediments.
Figure 5. (a) Map of the paleolake deposits in the Hushe village area. (b) View looking south-east from Hushe showing paleolake sediments outcrops; deposit thickness is indicated by the white arrow. (c) Paleolake deposits between Talis and Balegon; the orange dashed line indicates the limit between the alluvial fan deposits and the lacustrine deposits. (d) Gully erosion near Saling. (e) Rills and gullies on scree deposits in the upper part of Talis tributary, northward view. c and d show detail of scree deposits.
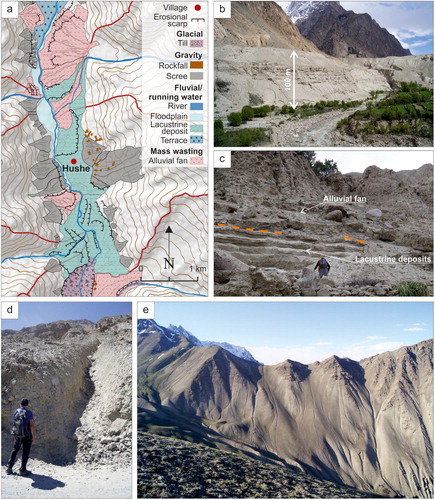
On the hillslopes, gullies (> 30 cm width and > 60 cm deep) and rills (< 30 cm width and < 60 cm deep) ((d,e)) mainly developed on scree gravitational deposits (subsection 4.3) can be observed. They follow the line of maximum slope (around 30°, although it varies between 10° and 54°) along a vertical gradient that goes from 300 to 1600 metres with channel lengths from 300 to 3000 metres. They are more frequent and of greater longitudinal development to the south of the valley (Main Map), on the west side, around the villages of Saling and Machulu, where the flow can reach a high energy due to the magnitude (slope and length) of the channel and its path. In general, these rills and gullies reach the Hushe River and do not leave any sedimentary record due to the high redistribution capacity of the latter.
4.3. Associated with gravitational processes
Rockfalls mainly occur on the western hillside, in some cases related to the detachment of rocks directly from a rocky erosional scarp but in most cases the rocks come from previous deposits such as tills, alluvial fans or terraces. In New Kande ((a)) an accumulation of angular megaclasts up to 10 m in diameter covers the surface of alluvial deposits and river terraces located 110 metres above river level. The rupture scar (∼500 m wide) stands 800–1300 m above the current valley and the piles of blocks indicate multiple rockfall events.
Figure 6. (a) Accumulation of megaclasts in New Kande. (b) Rocks of different sizes in the road slope, close to Marzigon. (c) Material extraction from the bottom of the scree deposit on the west side of the road between New Kande and Hushe. (d) Detail of a scree deposit.
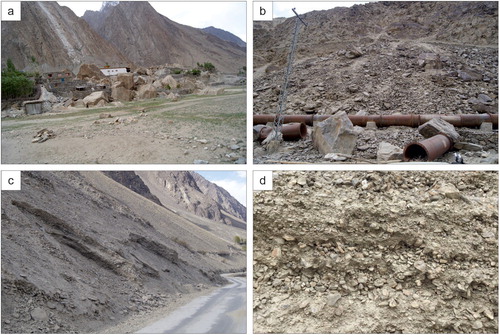
Figure 7. (a) View looking north from Marzigon showing the alluvial fan in Kunde (located by the white arrow), where the river is eventually dammed. (b) View looking south of the alluvial fan in the outlet of Kande tributary (located by the white arrow), taken from New Kande village; in the background Kunde (indicated by the black arrow) can be distinguished. (c) Debris deposits coming from diamictites in the upper part of Kunde tributary valley. (d) Rock avalanche type non-cohesive deposits in the Kande tributary.
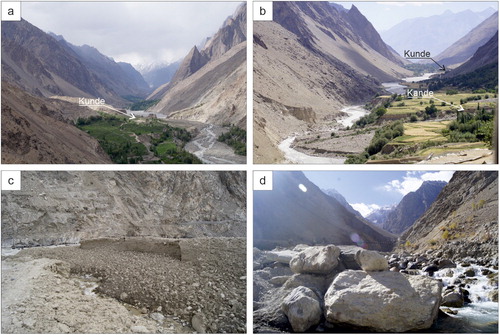
Many of the rockfalls are associated with the construction of the road that connects the Hushe Valley from north to south ((b,c)). Those works result in road slopes with different characteristics: (a) nearly vertical cut slopes with highly fractured rocks, (b) slopes in sediments with low cohesion and a great diversity of block sizes, or (c) slopes in gravel sediments without any matrix.
Scree deposits have been mapped all along the valley and on both hillsides (Main Map). They are formed by accumulations of centimetre-sized rock fragments at the foot of erosional scarps due to periodic falling. These accumulations show stratified alternating coarser and finer layers. The layers do not exceed 30° of dip, varying between 23-28°. Coarse layers show an openwork (clast supported) structure, with a heterogeneous clast size distribution ((d)). The fine layers are composed of clasts with a matrix-supported texture. Different processes might be responsible for this type of stratified deposits (CitationGarcía-Ruiz et al., 2001; CitationSass & Krautblatter, 2007; Citationvan Steijn et al., 1995). In this case, they are interpreted as the consequence of the fragmentation of rocks due to freeze–thaw cycles and accumulation by gravity, however, the influence of other processes in the final distribution of sediments, as the contribution of debris flow, cannot be discarded at this point. The associated forms are mostly fan-shaped, with slightly concave longitudinal profiles and with the larger clasts in the distal areas ((c,d)). Cartographically three different categories can be distinguished in the valley: (a) well-defined conical forms, with widths of more than 0.5 km; (b) well-defined conical forms, but of reduced dimensions (< 0.5 km); and (c) as a sheet, covering the erosional scarps (Main Map). The thickness of these deposits is very variable both within the same unit and between different units, but can exceed 10 metres. As they have an open texture, the accumulations are very unstable. In the valley, this type of deposit is used as road construction material. The material is extracted from the bottom of the deposit causing instabilities and small landslides mostly on the road slopes ((c)).
4.4. Mass wasting sediments and deposits
The steep and unstable slopes of the lateral valleys, with crumbly bedrock and tills, provide large volumes of debris that are reworked by streams to form large active alluvial fans at the outlet of the tributaries ((a,b)). There is some controversy about the appropriateness of the term alluvial fan (CitationJarman et al., 2011), however, in this case the broader definition has been chosen (CitationHarvey, 2018) assuming that diverse processes or sets of processes may have been involved in its formation.
The distribution of the alluvial fans shows asymmetry in the valley: in the northern half, the fans located in the eastern hillside prevail, while in the southern half, the largest fans are found in the outlet of west tributaries (Main Map). Many of the fans on the western side, such as Kunde, Talis or Machulu, are dominated by diamictites, since the sediments in these fans come directly from tills located in those tributary valleys (subsection 4.1), they are therefore reworked tills (Main Map). These alluvial fans are mainly formed by up to 2–3 m thick, massive sedimentary bodies of diamictites forming low-slope (5-10°) deposits dominated by angular and subangular rocks centimeters to metres in size ((c)). However, in the Kande tributary and others, the fans show gravels and blocks of very different size with an open matrix structure alternating with the diamictite type sedimentary bodies. This alternating structure suggests that in these areas, part of the sediment may have come from rock falls or rock avalanches in the tributary valley. These fans located to the west are symmetrical, with a ‘telescopic’ type progradational evolution (CitationColombo, 2005). Meanwhile, the morphology of fans located to the east are ‘L-shaped,’ with an active braided channel located to the south of the fan. Sediment availability in origin, transport capacity of the tributaries and main channels, and, specially, fan accommodation space have been proved to have effects on fan shape (CitationSorriso-Valvo et al., 1998), however, in such an active area, tectonic factors should also be considered as a cause of this fan planform asymmetry (CitationGiles et al., 2016; CitationLeeder, 1999).
Erosional scarps are observed all over the valley (Main Map), related to most of the mentioned processes. These non-tectonic scarps are located on top of scree type deposits (subsection 4.3), related to tills (subsection 4.1), to lacustrine deposits or river terraces (subsection 4.2) ((a,b) and (b)), as well as to alluvial fans (subsection 4.4).
5. Summary and conclusions
This paper presents a new landforms map for the lower Hushe Valley. Thirteen landform types associated with glacial, fluvial, gravitational and mass wasting processes have been identified and mapped throughout the valley (). The distribution of those landforms on the valley differs between the eastern and the western hillslopes, and sometimes also from north to south. This asymmetry may be related, among other factors, with the different geological materials found on both sides of the Hushe River and the location of the Shyok Suture Zone in the area. In the east, materials from the Karakoram Batholith are found, and in the west and north, those conforming the Karakoram Metamorphic Complex, although they show a higher metamorphic degree in the north.
There are few ancient glacial deposits in the valley and most of them appear partially eroded or reworked. These tills are more frequent in the western hillside, while in the eastern side erosion forms prevail (shoulders), both at a height of 700–1000 metres above the valley bottom. The distribution of tills influences the type of materials transported by the lateral streams, and hence, the type of alluvial fan. Alluvial fans formed at the outlet of the tributary streams make the Hushe a fragmented valley. Those lateral alluvial fans also cause and have caused in the past the total damming of the Hushe River, which in some occasions has lasted long enough to form a lake that has left more than 300 m of stratigraphic record.
There are some differences in the alluvial fans coming from the lateral valleys regarding; (1) their morphology (‘L-shaped’ or symmetric); (2) their location (alluvial fans developed on the eastern hillside prevail in the north, and larger alluvial fans located on the western side do so in the south); (3) and the type of deposits they are related to (cohesive deposits, and open texture rock avalanches or rock falls with large rocks). The arrival of those materials poses a real threat to the inhabitants settled at the exits of these lateral valleys, and could affect the infrastructures along the lower Hushe Valley (road, electricity and water), as well as agricultural areas, located in the terraces that the Hushe River currently erodes. Furthermore, under the current climate change scenario, this type of event could become more frequent (CitationBishop et al., 2010), as the number of flooding events on the Hushe River itself could also increase.
Some of the gravitational processes currently active in the valley as rockfalls, from rocky scarps or tills, and small landslides associated to scree type deposit are linked to infrastructure construction that mainly affects the west hillslope of the valley.
This map is the first and necessary step towards a deep assessment on geological risk related to external processes in the area. Furthermore, it is also designed as a basis for future chronological studies and for a detailed reconstruction of geomorphological processes that will help understanding the actual landscape and the future threats for the Hushe Valley inhabitants.
Software
Mapping and image processing was conducted using QGIS Geographic Information System.
Geolocation information
Hushe Valley general GPS coordinates: 35.362595 N, 76.368633 E.
Zabaleta_et_al_Main_Map_reviewed.pdf
Download PDF (60.7 MB)TJOM_1822939_Main Map Review_2.pdf
Download PDF (80.1 MB)Acknowledgements
We are grateful for the support given by the people of the Felix Foundation Baltistan (Machulu) during the fieldwork campaigns of 2018 and 2019. Without their help with the logistics and companionship it would not have been possible to get to the point where we are now. Additionally, the authors would like to thank Esther Cano and the Baltistan Fundazioa in the Basque Country (Spain) for their collaboration and support. We also thank reviewers for critical reading, suggestions and discussion that undoubtedly helped substantially improving and clarifying this manuscript.
Disclosure statement
The authors declare no competing interests.
Additional information
Funding
References
- Ahmed, M. F. , & Rogers, J. D. (2014). First-approximation landslide inventory maps for northern Pakistan, using ASTER DEM data and geomorphic indicators. Journal of Environmental & Engineering Geoscience , 20 (1), 67–83. https://doi.org/10.2113/gseegeosci.20.1.67
- Ahmed, M. F. , Rogers, J. D. , & Ismail, E. H. (2018). Knickpoints along the upper Indus River, Pakistan: An exploratory survey of geomorphic processes. Swiss Journal of Geosciences , 111 (1-2), 191–204. https://doi.org/10.1007/s00015-017-0290-3
- Ballantyne, C. K. (2002). Paraglacial geomorphology. Quaternary Science Reviews , 21 (18-19), 1935–2017. https://doi.org/10.1016/S0277-3791(02)00005-7
- Bhambri, R. , Hewitt, K. , Kawishwar, P. , & Pratap, B. (2017). Surge-type and surge-modified glaciers in the Karakoram. Scientific Reports , 7 (1), 15391. https://doi.org/10.1038/s41598-017-15473-8
- Bishop, M. P. , Bush, A. B. G. , Copland, L. , Kamp, U. , Owen, L. A. , Seong, Y. B. , & Shroder, J. F. Jr (2010). Climate change and mountain topographic evolution in the Central Karakoram, Pakistan. Annals of the Association of American Geographers , 100 (4), 772–793. https://doi.org/10.1080/00045608.2010.500521
- Colombo, F. (2005). Quaternary telescopic-like alluvial fans, Andean Ranges, Argentina. In A. M. Harvey , A. E. Matter , & M. Stokes (Eds.), Alluvial fans,: Geomorphology, Sedimentology, dynamics (pp. 69–84). Geological Society. Special Publications. https://doi.org/10.1144/GSL.SP.2005.251.01.06
- Easterbrook, D. J. (1993). Surface processes and land forms . Macmillan.
- García-Ruiz, J. M. , Valero-Garcés, B. , González-Sampériz, P. , Lorente, A. , Martí-Bono, C. , Beguería, S. , & Edwards, L. (2001). Stratified scree in the Central Spanish Pyrenees: Palaeoenvironmental implications. Permafrost and Periglacial Processes , 12 (3), 233–242. https://doi.org/10.1002/ppp.388
- Giles, P. T. , Whitehouse, B. M. , & Karymbalis, E. (2016). Interactions between alluvial fans and axial rivers in Yukon, Canada and Alaska, USA. Geological Society, London, Special Publications , 440 (1), 23–43. https://doi.org/10.1144/SP440.3
- Harvey, A. (2018). Alluvial fans. Reference module in earth systems and environmental sciences. Elsevier , https://doi.org/10.1016/B978-0-12-409548-9.11066-8
- Hewitt, K. (1998). Catastrophic landslides and their effects on the upper Indus streams, Karakoram Himalaya, northern Pakistan. Geomorphology , 26 (1–3), 47–80. https://doi.org/10.1016/S0169-555X(98)00051-8
- Hewitt, K. (1999). Quaternary moraines vs catastrophic avalanches in the Karakoram Himalaya, northern Pakistan. Quaternary Research , 51 (3), 220–237. https://doi.org/10.1006/qres.1999.2033
- Hewitt, K. (2005). The Karakoram anomaly? Glacier expansion and the ‘elevation effect,’ Karakoram Himalaya. Mountain Research and Development , 25 (4), 332–340. https://doi.org/10.1659/0276-4741
- Hewitt, K. , Gosse, J. , & Clague, J. J. (2011). Rock avalanches and the pace of late Quaternary development of river valleys in the Karakoram Himalaya. Geological Society of America Bulletin , 123 (9-10), 1836–1850. https://doi.org/10.1130/B30341.1
- Jarman, D. , Agliardi, F. , & Crosta, G. B. (2011). Megafans and outsize fans from catastrophic slope failures in Alpine glacial troughs: The Malser Haide and the Val Venosta cluster, Italy . Special Publications.
- Leeder, M. (1999). Sedimentology and sedimentary basins: From Turbulence to tectonics . Blackwell Science.
- Leland, J. , Reid, M. R. , Burbank, D. W. , Finkel, R. , & Caffee, M. (1998). Incision and differential bedrock uplift along the Indus River near Nanga Parbat, Pakistan Himalaya, from 10Be and 26Al exposure age dating of straths. Earth and Planetary Science Letters , 154 (1-4), 93–107. https://doi.org/10.1016/S0012-821X(97)00171-4
- Miller, K. J. (1984). The International Karakoram project , 2. Cambridge University Press.
- Oshebi, E. , Badi, F. M. , Shaltami, A. M. , & Fares, O. S. , & F, F. (2017). Alluvial terraces as a measure of vertical movements and neotectonics: Evidences from Wadi Zazah, Al Jabal Al Akhdar, NE Libya. Libyan Journal of Science and Technology , 6 (1), 19–24.
- Owen, L. A. , (1988). Terraces , uplift and climate in the Karakoram Mountains, Northern Pakistan . [Unpublished doctoral Thesis]. University of Leicester, 399 pp.
- Owen, L. A. (1989). Terraces, uplift and climate in the Karakoram mountains, northern Pakistan: Karakoram intermontane basin evolution. Zeitschrift für Geomorphologie, N.F., Suppl.-Bd , 76 , 117–146.
- Palin, R. M. , Searle, M. P. , Waters, D. J. , Horstwood, M. S. A. , & Parrish, R. (2012). Combined thermobarometry and geochronology of peraluminous metapelites from the Karakoram metamorphic complex, north Pakistan; New insight into the tectonothermal evolution of the Baltoro and Hunza valley regions. Journal of Metamorphic Geology , 30 (8), 793–820. https://doi.org/10.1111/j.1525-1314.2012.00999.x
- Planet Team . (2017). Planet application program interface: In space for life on earth. https://api.planet.com
- Rex, A. J. , Searle, M. P. , Tirrul, R. , Crawford, M. B. , Prior, D. J. , Rex, D. C. , Barnicoat, A. , & Bertrand, B. M. (1988). The Geochemical and tectonic evolution of the Central Karakoram, north Pakistan. Philosophical Transactions of the Royal Society of London. Series A, Mathematical and Physical Sciences , 326 (1589), 229–255. https://doi.org/10.1098/rsta.1988.0086
- RGI Consortium . (2017). Randolph glacier inventory – A dataset of global glacier outlines: Version 6.0: Technical Report Global land Ice Measurements from space, Colorado, USA . Digital Media. http://www.glims.org/RGI/rgi60_dl.html
- Rolland, Y. , Carrio-Schaffhauser, E. , Sheppard, S. M. F. , Pêcher, A. , & Esclauze, L. (2006). Metamorphic zoning and geodynamic evolution of an inverted crustal section (Karakorum margin, N Pakistan), evidence for two metamorphic events. International Journal of Earth Sciences , 95 (2), 288–305. https://doi.org/10.1007/s00531-005-0026-x
- Sakai, A. (2012). Glacial lakes in the Himalayas: A review on formation and expansion processes. Global Environmental Research , 16 , 23–30. https://jglobal.jst.go.jp/en/detail?JGLOBAL_ID=201202230253094241
- Sass, O. , & Krautblatter, M. (2007). Debris flow-dominated and rockfall-dominated talus slopes: Genetic models derived from GPR measurements. Geomorphology , 86 (1-2), 176–192. https://doi.org/10.1016/j.geomorph.2006.08.012
- Searle, M. P. , Rex, A. J. , Tirrul, R. , Rex, D. C. , Barnicoat, A. , & Windley, B. F. (1989). Metamorphic, magmatic, and tectonic evolution of the central Karakoram in the Biafo-Baltoro-Hushe regions of northern Pakistan. In: Malinconico, L. L. Jr . & Lillie, R. J. (Eds.), Tectonics of the western Himalayas . Geological Society of America, Special Paper 232, 47–73.
- Senese, A. , Maragno, D. , Fugazza, D. , Soncini, A. , D’Agata, C. , Azzoni, R. S. , Minora U. , Ul-Hassan R. , Vuillermoz E. , Asif Khan M. , Shafiq Rana A. , Rasul G. , Smiraglia C. , & Diolaiuti, G. A. (2018). Inventory of glaciers and glacial lakes of the central Karakoram national park (CKNP – Pakistan). Journal of Maps , 14 (2), 189–198. https://doi.org/10.1080/17445647.2018.1445561
- Seong, Y. B. , Bishop, M. P. , Bush, A. , Clendon, P. , Copland, L. , Finkel, R. C. , Kamp, U. , Owen, L. A. , & Shroder, J. F. (2009). Landforms and landscape evolution in the Skardu, Shigar and Braldu valleys, Central Karakoram. Geomorphology , 103 (2), 251–267. https://doi.org/10.1016/j.geomorph.2008.04.026
- Seong, Y. B. , Owen, L. A. , Bishop, M. P. , Bush, A. , Clendon, P. , Copland, L. , Finkel R. C. , Kamp U. , & Shroder, J. F. (2008). Rates of fluvial bedrock incision within an actively uplifting orogen: Central Karakoram mountains, northern Pakistan. Geomorphology , 97 (3-4), 274–286. https://doi.org/10.1016/j.geomorph.2007.08.011
- Shean, D. (2017). High mountain Asia 8-meter DEM Mosaics derived from optical imagery, version 1 [tile-370] . NASA National Snow and Ice Data Center Distributed Active Archive Center.
- Shroder, J. F. , Owen, L. A. , Seong, Y. B. , Bishop, M. P. , Bush, A. , Caffee, M. W. , Copland L. , Finkel R. C. , & Kamp, U. (2011). The role of mass movements on landscape evolution in the Central Karakoram: Discussion and speculation. Quaternary International , 236 (1–2), 34–47. https://doi.org/10.1016/j.quaint.2010.05.024
- Sorriso-Valvo, M. , Antronico, L. , & Le Pera, E. (1998). Controls on modern fan morphology in Calabria. Geomorphology , 24 (2–3), 169–187. https://doi.org/10.1016/S0169-555X(97)00079-2
- van Steijn, H. , Bertan, P. , Francou, B. , Hétu, B. , & Texier, J. P. (1995). Models for the genetic and environmental interpretation of stratified slope deposits: Review. Permafrost and Periglacial Processes , 6 (2), 125–146. https://doi.org/10.1002/ppp.3430060210
- Watson, C. S. , Quincey, D. J. , Carrivick, J. L. , Smith, M. W. , Rowan, A. V. , & Richardson, R. (2017). Heterogeneous water storage and thermal regime of supraglacial ponds on debris-covered glaciers. Earth Surface Processes and Landforms , 43 (1), 229–241. https://doi.org/10.1002/esp.4236