ABSTRACT
Geomorphological mapping in mountain regions is key for a better understanding of past and present environmental dynamics. Here, we present a 1:25000 scale geomorphological map covering 553 km2 of the Aran Valley, Upper Garonne Basin (Central Pyrenees). The map identifies 44 different geomorphological units classified under glacial, periglacial, nival, karst, slope, alluvial, and fluvial categories. The area includes geomorphic evidence of past Quaternary glaciations reconstructed based on the distribution of moraines from the valley floor to the highest cirques. Following deglaciation of the valley, the landscape was mainly reshaped by periglacial, slope, alluvial and fluvial processes. In addition to paleoenvironmental reconstruction, the map can also be used to promote geoheritage and geoconservation, as well as for planning. As such, it is of relevance for areas exposed to natural hazards, since present-day slope failures and debris flows frequently impact the villages and infrastructures across the valley floor.
1. Introduction
Geomorphological mapping in mountains has significantly advanced over the last decades in parallel to the development of survey techniques and availability of aerial and satellite images (CitationChandler et al., 2018), as well as of airborne LiDAR surveys (CitationOguchi et al., 2012). A better understanding of present-day geomorphological processes allows improved territorial management in areas exposed to natural hazards (CitationParon & Claessens, 2011). Accurate geomorphological maps of alpine regions can be used to reconstruct their past environmental histories and to promote the inventorying and classification of geoheritage (CitationCoratza & de Waele, 2012). As such, geomorphological maps are important tools, leading to the implementation of geoconservation measures that may be applied to promote regional development policies.
Geomorphological maps have been used as a scientific tool since the 1960s, developing into more complex maps on glaciated landscapes of mountains, piedmonts, and glacial forefields at the beginning of the twenty-first century (CitationKnight et al., 2011). Several geomorphological maps have been recently published for mid-latitude mountains, such as the Iberian mountains (CitationGonzález-Gutiérrez et al., 2017; CitationPellitero, 2014) and the Alps (CitationCoratza et al., 2019; CitationLambiel et al., 2016), or for areas such as the Tibetan Plateau (CitationLindholm & Heyman, 2016; CitationSchneider et al., 2021). In the Pyrenees, besides the geomorphological maps by the ‘Instituto Geológico y Minero de España’ at 1:50000, detailed geomorphological mapping has mostly focused on (i) National Parks, such as Ordesa-Monte Perdido (CitationGarcía-Ruiz & Martí-Bono, 2001) and Aigüestortes-Estany de Sant Maurici (CitationMartínez-Rius, 2010), (ii) some large valleys, such as Benasque (CitationGarcía-Ruiz et al., 1992) or Andorra (CitationCopons, 2005), (iii) singular landscape features for all valleys of the southern part of the range, such as glacial landforms (CitationMartí-Bono & García-Ruiz, 1994), or (iv) in small valleys and glacial cirques, where geomorphological sketches and maps supported various research topics, mainly on glacial, periglacial or slope dynamics (e.g. CitationAndrés et al., 2018; CitationFernandes et al., 2017; CitationJarman et al., 2014; CitationSerrano et al., 2020). To date, a detailed map including the wide range of geomorphological features existing in the Aran Valley is still missing.
This work focuses on the Aran Valley, one of the longest glacial valleys in the Pyrenees, which showed a ca. 80 km long valley glacier at the maximum ice extent of the last glacial cycle (CitationFernandes et al., 2017). The geomorphological and paleogeographical richness of the area has resulted in several publications (CitationFernandes et al., 2021b, Citation2021a, Citation2018, Citation2017; CitationLopes et al., 2018; CitationOliva et al., 2021). The valley has ca. 10,000 inhabitants distributed in 33 settlements and infrastructures are widespread both in the valley floor and mountain slopes. Hence, an accurate identification and analysis of the geomorphological features become important for a better spatial planning, for the mitigation of natural hazards and for the implementation of geoconservation measures and promoting geoconservation. To present, the existing geomorphological coverage of the Aran Valley is incomplete and not systematic, consisting of: (i) a low-resolution map from the early 1990s focussing on the main glacial features (CitationSerrat et al., 1994), (ii) a geomorphological map of the northern part of the Aran Valley including only the Toran, Varradòs and Unhòla tributaries (CitationMartí-Soler, 1988), (iii) a map of glacial and postglacial features of the SW area of Aran, namely the Joèu and Nère valleys (CitationBordonau, 1985), and (iv) the geomorphological map of the Ruda Valley (CitationFernandes et al., 2017).
The objectives of this work are to: (i) improve the existing geomorphological maps, which covered only 200 km2 and expand the survey to the entire 553 km2 of the Aran Valley, and (ii) offer the local authorities a high-resolution geomorphological map, that may be used as a tool for spatial planning purposes.
2. Study area
The Aran Valley is the northwesternmost district of Catalonia within Spanish administrative territory (). The headwaters are on the central axis of the Pyrenees, with elevations above 3000 m a.s.l. (Molières Peak, 3009 m asl; Besiberri Nord Peak, 3007 m), whereas the valley floor, near the French border, is at ca. 600 m. The Aran Valley constitutes the headwaters of the Upper Garonne Basin at its easternmost flank ().
Figure 1. Location of the Aran Valley within the Pyrenean range, including the main lithological units of the study area.
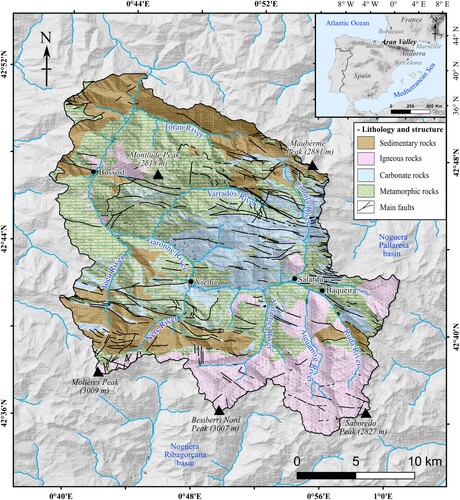
The current climate in the Aran Valley is dominated by wet and cool Atlantic air masses. Annual precipitation in the valley floor is ca. 900 mm at Vielha village (980 m), and increases to 1232 mm at the Port de la Bonaigua water divide (2266 m). Annual air temperatures (MAAT) at these two sites are 9.6°C and 2.7°C, respectively. The regional 0°C isotherm is at 2950 m asl in the Central Pyrenees (CitationLópez-Moreno et al., 2016), with only the highest summits recording negative MAATs. The Aran Valley shows typical Euro-Siberian vegetation, with flat-leaf, deciduous forests in the lower elevations and conifers up to ca. 2200–2300 m asl, where they are replaced by alpine meadows (CitationBolòs & Vigo, 1984).
The Aran Valley is part of the Paleozoic basement of the Central Pyrenees that was uplifted during the Alpine orogeny (CitationGarcía-Sansegundo et al., 2013; CitationQuesada & Oliveira, 2019). The rise of the Pyrenees formed several thrusts organized in two structural domains: the first is mainly located in the northern part of the Aran Valley with E-W direction subhorizontal structures, and the second constitutes the central part of the valley and mainly includes E-W subvertical folds (CitationSitter & Zwart, 1962; CitationZwart, 1979). Both were intruded by granitoids, such as the Maladeta Batholith and the Dome of Bossòst (CitationGarcía-Sansegundo, 2004). Bedrock in the north and central valley slopes is composed of metamorphic (slates, schists and marbles) and sedimentary rocks (limestones, lutites, conglomerates and sandstones) from the Cambrian to the Devonian periods (CitationGeològic de Catalunya, 2017). The highest areas are mostly composed of igneous rocks (granites) that intruded during the Carboniferous period (CitationMartínez et al., 2016; CitationMezger & Gerdes, 2016). Quaternary deposits are located along the narrow valley bottoms, floors and footslopes of the glacial cirques and in parts of the slopes.
Similar to other areas of the Pyrenees, the Aran Valley was extensively glaciated during Quaternary glacial cycles (CitationOliva et al., 2019). A ca. 80-km long glacier descended along the main Garonne Valley until the glacial basin near Montrejeau at 400–500 m asl, where it formed a series of frontal moraine ridges, both at ca. 130 ka and prior to 24–21 ka (CitationFernandes et al., 2021b). At those periods, the equilibrium line altitude (ELA) was located at ca. 1500 m (CitationFernandes et al., 2017).
The Aran Valley presents a U-shaped cross-section and a prevailing S-N direction, turning E-W between the villages of Baquèira and Vielha (). The relief may be divided into three major units: (i) interfluves (peaks, ridges and summit plateaus) and glacial cirques, (ii) mountain slopes, and (iii) gently sloping floors of the Garonne Valley and its tributaries (Aiguamòg, Valarties, Nère, etc.). The landscape was extensively shaped by Quaternary glaciers, although the chronological framework of glacial oscillations in the northern slope of the Central Pyrenees is yet to be established (CitationFernandes et al., 2021a; CitationOliva et al., 2019). Postglacial dynamics has been mainly driven by periglacial and nival processes in the highest areas, with the occurrence of frequent permafrost-related features, such as rock glaciers and protalus lobes (CitationFernandes et al., 2018), as well as by hillslope and alluvial processes across the slopes and valley floor (CitationFernandes et al., 2020). Warmer temperatures during the Holocene promoted soil development, slope stabilization and forest expansion (CitationGonzález-Sampériz et al., 2017; CitationTejedor-Rodríguez et al., 2021). Since the Neolithic/Chalcolithic periods, and particularly since 4 ka cal BP, humans have significantly modified the landscape. Initially the impacts were associated with forest clearing to expand transhumance practices, that triggered hydrologic and erosion changes (CitationGarcía-Ruiz et al., 2020; CitationTejedor-Rodríguez et al., 2021). Over the last millennium, particularly since 1.1 ka cal BP, human settlements grew and agriculture and grazing expanded across the valley floor (CitationGarcés-Pastor et al., 2017). Since the latest twentieth century, a tourism-driven economy has dramatically changed the landscape, with the construction of infrastructures and the expansion of settlements, mainly across the valley floor.
3. Methodology
The elaboration of the geomorphological map of the Aran Valley at 1:25000 scale was based on:
extensive bibliographical and cartographic review, including georeferencing of existing geomorphological maps by CitationBordonau (1985), CitationMartí-Soler (1988) and CitationSerrat et al. (1994),
remote sensing data interpretation, including the analysis of LiDAR-derived terrain models (digital elevation model, hillshade and slope), digital orthophotomaps and very high-resolution Google Earth imagery (),
systematic field surveys during the summers of 2015, 2019, 2020 and 2021 for reconnaissance, landform identification and validation ((C)), and
editing in GIS environment (ArcGIS) and the final map layout (Illustrator).
Figure 2. Steps followed during the elaboration of the geomorphological map; (A) combination of hillshade (40% transparent) with orthophotomaps; (B) first draft of the geomorphological map; (C) field validation of the first geomorphological draft with pictures, such as till cover (C1), superficial deformation of till cover at the plateau (C2), peat area (C3), and alluvial fan (C4). (D) final geomorphological map respecting the Main map legend.
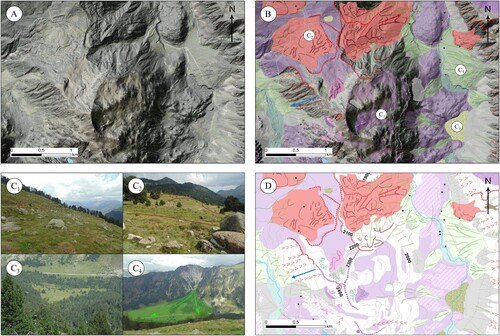
Table 1. Attributes of the remote sensing products used for the geomorphological map.
The geomorphological map main focus is on landforms and processes, with synthesizing the criteria used for their mapping. They were outlined and symbolized according to CitationJoly (1997) and CitationLambiel et al. (2013) and grouped following criteria on glacial, periglacial, slope, alluvial, fluvial, nival and karst features. The symbolization uses lines for the linear landforms (e.g. moraine crests), points for the isolated landforms or deposits (e.g. erratics), and polygons for larger non-linear features (e.g. fluvial plain). Additional information includes the main faults (CitationGeològic de Catalunya, 2017), pre-Quaternary geology (1:50000; CitationGeològic de Catalunya, 2017), rivers and lakes (Institut Cartogràfic i Geològic de Catalunya ICGC), 100 m contours (derived from the Advanced Spaceborne Thermal Emission and Reflection Radiometer – ASTER DEM), main peaks (Instituto Geográfico Nacional de España; IGN), and main infrastructures (ICGC).
Table 2. Location and morphological characteristics of the landforms and processes drew in the Main map.
All landforms were validated during the summer when the snow-free landscape allowed for the identification of geomorphological features. Most landforms were identified in LiDAR-derived models, online anaglyph map viewer (https://www.ign.es/iberpix2/visor/), satellite imagery, and orthophotomaps, with only the small-size features having been identified in the field.
4. Results
4.1 Glacial landforms
Abundant erosional and depositional glacial landforms occur from the highest areas to the Aran Valley floor. The highest areas show glacial cirques ((D)), where ice accumulated before filling the valleys with a maximum thickness of ca. 800 m (CitationFernandes et al., 2017). The main valley as well as its tributaries display well-preserved U-shaped sections. Glacial erosion shaped overdeepened basins along the valley bottoms (e.g. Valarties Valley), that are occupied by lakes, peatlands (e.g. Ruda Valley; (C)) and fluvial plains (e.g. main Garonne Valley). Intense glacial erosion is also shown in smaller landforms, such as roches moutonneés and glacially polished surfaces – including striations – along the valleys (e.g. next to the Maubèrme Peak). A significant part of the glacially eroded material was transported downvalley and accumulated as terminal and lateral moraines (e.g. Nère Valley; (A)), till or scattered erratics (e.g. main Garonne Valley; (B)). Moraines accumulated during different periods of advance or standstills during glacial retreat and are distributed in a wide elevation range: from the highest cirques at 2400 to 2600 m asl (e.g. Aiguamòg Valley) to the valley floors at 1300 m asl (e.g. Nère Valley), or even on the marginal and elevated plateaus including deposits located 500 m above the riverbed (e.g. between Ruda and Aiguamòg valleys).
Figure 3. Examples of the different glacial landforms identified in the area: (A) lateral moraine around 1600 m at the Nère Valley; (B) E-W perspective of the main Garonne Valley with an erratic boulder of granite distributed on a slate outcrop above Salardú village; (C) glacial lake distributed in an overdeepened basin at the Unhòla Valley; (D) glacial cirque of the Montardo Peak at the Valarties Valley.
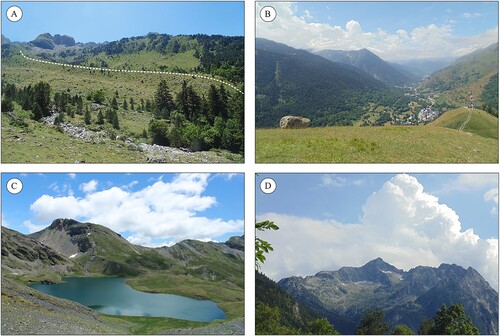
4.2 Periglacial landforms
The frozen ground has promoted the development of numerous landforms, some of which are still active under current climate conditions. Rock glaciers ((A)) and protalus lobes ((B)) provide evidence for the past occurrence of permafrost (CitationFernandes et al., 2018), with only one active rock glacier having been identified in the area (CitationSerrano et al., 2011). Above ca. 2300 m asl, smaller landforms associated with seasonal frost show traces of activity. In relatively flat summit surfaces, there are block fields (e.g. between Joèu and Nère valleys) and patterned ground features (e.g. next to the Maubèrme Peak; (D)), while in gentle slopes, there are block streams (e.g. Ruda Valley; (C)) and solifluction lobes (e.g. Salient Valley).
Figure 4. Examples of the different periglacial landforms identified in the area: (A) relict rock glacier distributed across the glacial cirque floor of Locampo, Ruda Valley; (B) active talus slope with protalus lobes at the Nère Valley; (C) relict block stream at 2200 m in the Ruda Valley; (D) inactive sorted cirques at 2480 m in the Mauberme cirque, Unhòla Valley.
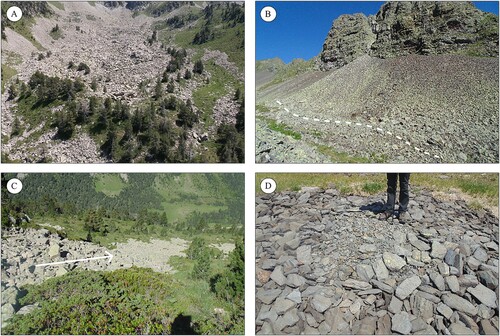
4.3 Nival landforms
Under current climate conditions, snow remains for 6–8 months/year above 2000–2200 m asl with nival dynamics affecting upper slopes. Snow avalanches are relevant on steep slopes (e.g. Valarties Valley) and in shady areas of the cirques and high valleys, snow-patches can persist during most of the year ((A)), triggering the formation of protalus ramparts (e.g. Nère Valley).
Figure 5. Examples of other geomorphological landforms identified in the area: (A) long-lying snow patch at 2350 m, in the north face of the Armèros Peak; Unhòla Valley (B) dolines located in the Sascorjada glacial cirque, Toran Valley; (C) Rock slope deformation at the Varradòs Valley; (D) alluvial fan connected with the fluvial plain at the Joèu Valley.
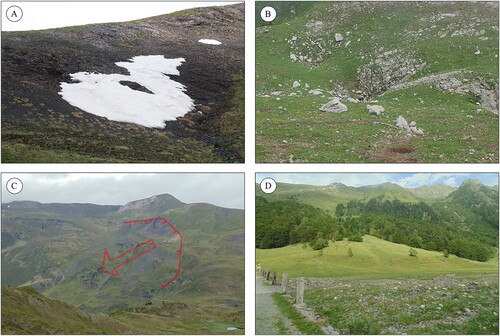
4.4 Karst landforms
Karst morphologies occur in limestone terrain, especially at the Unhòla valley, between the Armeròs and Maubèrme peaks. In these sectors, glaciers and dissolution have originated a glacio-karst landscape ((B)) with dolines, uvalas and karst windows.
4.5 Slope landforms and processes
The elevation difference of ca. 2500 m from the highest peaks to the lowest valley floors in the Aran results in very active slope processes, particularly in slopes between 25 and 35°. Past slope dynamics occurred mostly during the paraglacial stage consisted of catastrophic rock slope failures, rock slope deformations, rockfalls and landslides ((C)) (CitationFernandes et al., 2020). Postglacial gravitational processes generated thick debris mantles covering the slopes (e.g. main Garonne Valley). Talus cones and talus slopes, particularly those located at lower elevations (e.g. Nère Valley), have gradually become inactive, allowing for soil development and forest expansion. Some of them show traces of deep incision, following debris flows and gully erosion (e.g. Varradòs Valley). These processes also occur on fine-grained sedimentary rocks, such as lutites or shales.
4.6 Alluvial landforms and processes
Alluvial fans ((D)) are found at the contact between steep slopes and the valley floors, forming triangular-shaped features of fine sediments of variable sizes (e.g. main Garonne Valley). Depending on the prevailing lithology, alluvial fans can be also reshaped by debris flows, mudflows or rockfalls (e.g. main Garonne Valley). These are particularly intense during heavy rainfall or snow-melt episodes. Sediments can also accumulate in overdeepened basins in the valley heads (e.g. Ruda Valley), infilling ancient lakes and promoting the development of peatlands (e.g. Unhòla Valley).
4.7 Fluvial landforms
Rivers and streams flowing to the Garonne River show a high capacity to mobilize sediments, particularly during the snow-melting period. Whereas in some areas the rivers flow through gorges (e.g. Unhòla Valley), in others the valley presents a large flood plain that is used for agriculture and grazing purposes (e.g. Nère Valley). In the Aran Valley, there are more than 700 lakes of glacial origin distributed between 1566 and 2711 m asl with a 0.5 m2 ha mean size (Main map). In the Aran Valley, 80% of the lakes are located above 2000m asl, mostly in overdeepened basins in the granitic bedrock. At lower areas, the lakes are smaller and show infill of peat and proglacial sediments.
5. Discussion
The geomorphological map of the Aran Valley shows evidence that almost the entire valley was glaciated during the last glacial cycle, and only the peaks and rock ridges (<8% of the area) protruded above the ice as nunataks (Main map). Given the prevalence of glacial erosional landforms supporting basal sliding, the broad spectrum of periglacial, slope and alluvial phenomena existing in the area must have formed following deglaciation of the valley.
The chronology of the maximum ice extent of the last glacial cycle is still not well-known in the Central Pyrenees (CitationOliva et al., 2019). However, recent studies shed light on the chronological frame of the terminal basins. Glacial features associated with the Garonne paleoglacier in the Loures-Barousse-Barbazan basin at 470–680 m asl show an advance during the marine isotope stage (MIS)-6 and retreat during MIS-2 (CitationFernandes et al., 2021a). The presence of discontinuous morainic steps on the slopes above the main Garonne Valley suggests that glaciers reached an ice thickness of ca. 600–800 m during the maximum Pleistocene ice expansion (). The intensity of postglacial slope, alluvial and fluvial dynamics must have destroyed glacial evidence in the lowest areas of the Aran Valley, where no terminal moraines are preserved. Erosional (e.g. polished surfaces) and accumulation glacial landforms (e.g. moraines) are widespread in the upper parts of the valley. Indeed, exposure ages from polished surfaces showed glacier recession at 15–14 and at 12.7 ka. Moraines located in glacial cirques at 2080–2190 m and 2430–2470 m asl show ages of 13.5–13.0 and 12.9–12.6 ka, respectively. These relate to glacial advances or standstills in the Bølling-Allerød, as well as in the Bølling-Allerød to Younger Dryas transition (CitationFernandes et al., 2021b; CitationOliva et al., 2021). Such glacial landforms are a legacy from past processes and environments. The wide range of glacial landforms, its degree of preservation, as well as its singularity within southern Europe constitute key elements of the natural heritage and geodiversity of the Aran Valley.
Figure 6. Sequence of environmental phases shaping the landscape of the Aran Valley: (A) maximum ice extent of the last glacial cycle; (B) glacial tongues flowing downvalleys during a glacial advance/standstill (Oldest Dryas) within the long-term glacial retreat of the Garonne paleoglacier that favored also intense slope readjustment; (C) alpine and cirque glaciers (Younger Dryas) with very intense postglacial slopes dynamics, and the occurrence of permafrost that promoted the formation of rock glaciers; (D) deglaciated cirques with periglacial processes prevailing at higher elevations (inactive rock glaciers), and slope, alluvial and fluvial processes at lower elevations.
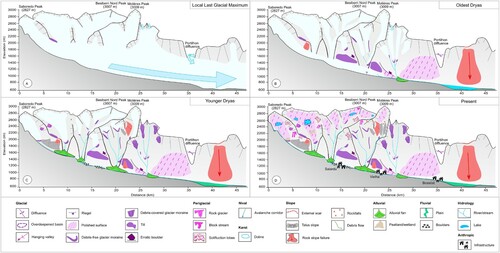
As glaciers disappeared from glacial cirques, periglacial morphogenesis became the prevailing process shaping the highest land surfaces. Indeed, rock glaciers and protalus lobes present in most cirques show evidence of permafrost in formerly glaciated cirques (CitationFernandes et al., 2018). In the Pyrenees, these periglacial features developed following glacial retreat at the end of both the Oldest and Younger Dryas cold events under paraglacial debuttressing of cirque slopes (CitationAndrés et al., 2018; CitationGarcía-Ruiz et al., 2016; CitationPalacios et al., 2017). The readjustment to new glacier-free conditions favored widespread slope failure events affecting both the bedrock and unconsolidated glacial sediments. CitationFernandes et al. (2020) show that more than 90% of the 135 large slope failures identified in the Aran Valley occurred within the glaciated domain of the last glacial cycle ((C) and ).
Since the onset of the Holocene, climate variability ranged within ± 2°C compared to present conditions in mid-latitude regions (CitationMayewski et al., 2004; CitationWanner et al., 2015). The current periglacial zone in the Aran Valley is located above ca. 2300 m asl (CitationFernandes et al., 2017) and thus seasonal frost processes should have affected elevations between ca. 2000 and 2500 m asl, with permafrost existing at the summit levels only during the coldest phases (CitationOliva et al., 2018). Holocene periglacial processes have reshaped slopes with widespread development of talus cones and slopes, as well as solifluction landforms, whereas patterned ground and block fields formed in flat or low gradient hillsides (e.g. Nère and Unhòla valleys – Main map). Climatic changes during the Holocene together with transhumance practices during the Mid-Late Holocene promoted vegetation shifts in the periglacial environment that enhanced or diminished hydrological and geomorphic dynamics (CitationGarcía-Ruiz et al., 2020).
Frequent high-intensity events, such as snow avalanches (e.g. Valarties Valley), debris flows (e.g. Varradòs Valley) or mudflows (e.g. Bargadera Valley), dominate the glacial landscape from the cirques and valley heads to the valley floors, where they contributed to the development of several generations of alluvial fans (e.g. Unhòla; CitationVictoriano et al., 2016). These are the major natural hazards currently affecting the Aran Valley, particularly debris flows that cause annual infrastructure damage, such as in the road network. An example occurred in the Valarties Valley, where a debris flow in May 2018 mobilized 50,000 m3 of sediments, destroying the local road (CitationPerdices Cos, 2019). Intense rainfall and snow-melting episodes lead to frequent flood events that trigger intense fluvial erosion in the highest sectors and accumulation of sediments in the flood plains of the lowest areas, such as in June 2013 where a flash flood in the Upper Garonne Basin caused damage to infrastructure exceeding 100 million euros (CitationBalasch et al., 2019; CitationVictoriano et al., 2016). Therefore, this high-resolution geomorphological map identifying landforms associated with natural hazards can be used by local authorities to better predict the location of hazards and their impacts.
6. Conclusions
Accurate mapping of geomorphological processes and landforms of the Aran Valley at 1:25000 scale allows a better understanding of the processes that have shaped the alpine landscape of this valley. The glacial landforms are diverse and widespread from the summits to the Garonne riverbed, and reveal that glacial, periglacial, nival, karst, slope, alluvial and fluvial processes have played a role in shaping the landscape of the entire valley. Once glaciers started retreating and thinning following the Last Glacial Maximum, periglacial processes dominated in the exposed ice-free terrain, accompanied by intense slope, alluvial and fluvial dynamics. In specific areas, nival and karstic processes also contributed to reshape the formerly glaciated environment.
Today, the steep slopes surrounding the main valley floor favor the occurrence of slope failures and frequent snow avalanches. In addition, sudden snow-melting episodes and torrential rains trigger also floods. Mapping these processes at a high-resolution improves the precision of the available tools and consequently enhances spatial planning, such as by validating susceptibility maps of geohazards or by promote geoheritage protection and assess geosite management.
Software
The geomorphological map was developed using ArcGIS 10.7.1 (ESRI) software. The final map was improved with Illustrator 16.3.
TJOM_A_2035266_Supplementary material
Download PDF (21.5 MB)Disclosure statement
No potential conflict of interest was reported by the author(s).
Data availability statement
The data described in this article are openly available at the ZENODO data repository (https://doi.org/10.5281/zenodo.4644265).
Additional information
Funding
References
- Andrés, N., Gómez-Ortiz, A., Fernández-Fernández, J. M., Tanarro García, L. M., Salvador-Franch, F., Oliva, M., & Palacios, D. (2018). Timing of deglaciation and rock glacier origin in the southeastern Pyrenees: A review and new data. Boreas, 47(4), 1050–1071. https://doi.org/10.1111/bor.12324
- Balasch, J. C., Tuset, J., Furdada, G., Porras, L., Castelltort, X., Barriendos, M., & Pino, D. (2019). The Upper Garonne flood series from discharge gauging data (Bossost, Val d’Aran, Spain) and reconstruction of historical floods (Saint-Béat, France), in: EGU General Assembly 2019.
- Benn, D. I., & Evans, D. J. A. (2010). Glaciers and glaciation. Hodder Education.
- Bolòs, O. d., & Vigo, J. (1984). Flora dels països Catalans. Editorial Barcino.
- Bordonau, J. (1985). Estudi geomorfològic del sector sudoccidental de la Vall d’Aran. L’evolució Quaternària de les valls dels rius Joeu i Nere. Tesis de Licenciatura.
- Chandler, B. M. P., Lovell, H., Boston, C. M., Lukas, S., Barr, I. D., Benediktsson, Í. Ö., Benn, D. I., Clark, C. D., Darvill, C. M., Evans, D. J. A., Ewertowski, M. W., Loibl, D., Margold, M., Otto, J.-C., Roberts, D. H., Stokes, C. R., Storrar, R. D., & Stroeven, A. P. (2018). Glacial geomorphological mapping: A review of approaches and frameworks for best practice. Earth-Science Reviews, 185, 806–846. https://doi.org/10.1016/j.earscirev.2018.07.015
- Copons, R. (2005). Mapa geomorfològic d’Andorra 1:50.000. Centre de Recerca en Ciències de la Terra. Govern d’Andorra.
- Coratza, P., & de Waele, J. (2012). Geomorphosites and natural hazards: Teaching the importance of geomorphology in society. Geoheritage, 4(3), 195–203. https://doi.org/10.1007/s12371-012-0058-0
- Coratza, P., Ghinoi, A., Marchetti, M., & Soldati, M. (2019). Geomorphology of the Rio Cisles basin (Odle Group, Dolomites, Italy). Journal of Maps, 15(2), 546–554. https://doi.org/10.1080/17445647.2019.1633426
- Evans, D. J. A. (2005). Glacial landsystems, glacial landsystems. Hodder Arnold.
- Evans, D. J. A. (2013). Glacial landsystems. Encyclopedia of quaternary sciences. Elsevier.
- Fernandes, M., Oliva, M., Palma, P., Ruiz-Fernández, J., & Lopes, L. (2017). Glacial stages and post-glacial environmental evolution in the Upper Garonne valley, Central Pyrenees. Science of The Total Environment, 584–585, 1282–1299. https://doi.org/10.1016/j.scitotenv.2017.01.209
- Fernandes, M., Oliva, M., & Vieira, G. (2020). Paraglacial slope failures in the Aran Valley (Central Pyrenees). Quaternary International, 566–567, 24–38. https://doi.org/10.1016/j.quaint.2020.07.045
- Fernandes, M., Oliva, M., Vieira, G., Palacios, D., Fernández-Fernández, J. M., Delmas, M., García-Oteyza, J., Schimmelpfennig, I., Ventura, J., & Team, A. (2021a). Maximum glacier extent of the penultimate glacial cycle in the Upper Garonne Basin (Pyrenees): new chronological evidence. Environmental Earth Sciences, https://doi.org/10.21203/rs.3.rs-788888/v1
- Fernandes, M., Oliva, M., Vieira, G., Palacios, D., Fernández-Fernández, J. M., García-Oteyza, J., Schimmelpfennig, I., Team, A., & Antoniades, D. (2021b). Glacial oscillations during the Bølling–Allerød Interstadial–Younger Dryas transition in the Ruda Valley. Journal of Quaternary Science, 1–17. https://doi.org/10.1002/jqs.3379
- Fernandes, M., Palma, P., Lopes, L., Ruiz-Fernández, J., Pereira, P., & Oliva, M. (2018). Spatial distribution and morphometry of permafrost-related landforms in the Central Pyrenees and associated paleoclimatic implications. Quaternary International, 470, https://doi.org/10.1016/j.quaint.2017.08.071
- French, H. (2007). The periglacial environment (Third ed). John Wiley & Sons, Ltd.
- Garcés-Pastor, S., Cañellas-Boltà, N., Pèlachs, A., Soriano, J. M., Pérez-Obiol, R., Pérez-Haase, A., Calero, M. A., Andreu, O., Escolà, N., & Vegas-Vilarrúbia, T. (2017). Environmental history and vegetation dynamics in response to climate variations and human pressure during the Holocene in Bassa Nera, Central Pyrenees. Palaeogeography, Palaeoclimatology, Palaeoecology, 479, 48–60. https://doi.org/10.1016/j.palaeo.2017.04.016
- García-Ruiz, J. M., Bordonau, J., Martínez de Pisón, E., & Vilaplana, J. M. (1992). Mapa geomorfológico de Benasque (M.T.N. 180). Geoforma Ediciones.
- García-Ruiz, J. M., & Martí-Bono, C. (2001). Mapa geomorfológico del parque nacional de ordesa y monte Perdido. Organismo Autónomo de Parques Nacionales.
- García-Ruiz, J. M., Palacios, D., González-Sampériz, P., De Andrés, N., Moreno, A., Valero-Garcés, B. L., & Gómez-Villar, A. (2016). Mountain glacier evolution in the Iberian peninsula during the younger dryas. Quaternary Science Reviews, 138, 16–30. https://doi.org/10.1016/j.quascirev.2016.02.022
- García-Ruiz, J. M., Tomás-Faci, G., Diarte-Blasco, P., Montes, L., Domingo, R., Sebastián, M., Lasanta, T., González-Sampériz, P., López-Moreno, J. I., Arnáez, J., & Beguería, S. (2020). Transhumance and long-term deforestation in the subalpine belt of the central Spanish Pyrenees: An interdisciplinary approach. Catena, 195, 104744. https://doi.org/10.1016/j.catena.2020.104744
- García-Sansegundo, J. (2004). Estructura varisca de los Pirineos. Geol. España. 254–258
- García-Sansegundo, J., Merino, J. R., Santisteban, R. R., & Leyva, F. (2013). Canejan-Vielha Mapa geologico 1:50 000. Instituto Geologico y Minero de España.
- Geològic de Catalunya. (2017). Base de dades geològiques de Catalunya 1:50.000 v1.0.
- Giles, D. P., Griffiths, J. S., Evans, D. J. A., & Murton, J. B. (2017). Chapter 3 geomorphological framework: Glacial and periglacial sediments, structures and landforms. Geological Society, London, Engineering Geology Special Publications, 28(1), 59–368. https://doi.org/10.1144/EGSP28.3
- González-Gutiérrez, R. B., Santos-González, J., Gómez-Villar, A., Redondo-Vega, J. M., & Prieto-Sarro, I. (2017). Geomorphology of the Curueño River headwaters, Cantabrian mountains (NW Spain). Journal of Maps, 13(2), 382–394. https://doi.org/10.1080/17445647.2017.1316217
- González-Sampériz, P., Aranbarri, J., Pérez-Sanz, A., Gil-Romera, G., Moreno, A., Leunda, M., Sevilla-Callejo, M., Corella, J. P., Morellón, M., Oliva, B., & Valero-Garcés, B. L. (2017). Environmental and climate change in the southern Central Pyrenees since the last glacial maximum: A view from the lake records. Catena, 149, 668–688. https://doi.org/10.1016/j.catena.2016.07.041
- Jarman, D., Calvet, M., Corominas, J., Delmas, M., & Gunnell, Y. (2014). Large-scale rock slope failures in the eastern pyrenees: Identifying a sparse but significant population in paraglacial and parafluvial contexts. Geografiska Annaler: Series A, Physical Geography, 96(3), 357–391. https://doi.org/10.1111/geoa.12060
- Joly, F. (1997). Glossaire de géomorphologie. Base de donnés sémiologiques pour la cartographie, Masson/Arm. ed. Paris.
- Kääb, A. (2013). Rock glaciers and protalus forms. In E. Scott, & C. Mock (Eds.), Encyclopedia of quaternary science (pp. 535–541). Elsevier.
- Knight, J., & Harrison, S. (2009). Periglacial and paraglacial environments: A view from the past into the future. Geological Society, London, Special Publications, 320(1), 1–4. https://doi.org/10.1144/SP320.1
- Knight, J., Mitchell, W. A., & James, R. (2011). Geomorphological field mapping. In M. J. Smith, P. Paron, & J. S. Griffiths (Eds.), Geomorphological mapping methods and applications (pp. 151–188). Elsevier.
- Lambiel, C., Maillard, B., Kummert, M., & Reynard, E. (2016). Geomorphology of the Hérens valley (Swiss Alps). Journal of Maps, 12(1), 160–172. https://doi.org/10.1080/17445647.2014.999135
- Lambiel, C., Maillard, B., Regamey, B., Martin, S., Kummert, M., Schoeneich, P., Ondicol, R. P., & Reynard, E. (2013). Adaptation of the geomorphological mapping system of the University of Lausanne for ArcGIS. In 8th International Conference on Geomorphology (IAG). Paris (p. 1176). https://doi.org/10.1016/j.geomorph.2010.03.00
- Lindholm, M.S., Heyman, J. (2016). Glacial geomorphology of the Maidika region, Tibetan Plateau. Journal of Maps, 12, 797–803. https://doi.org/10.1080/17445647.2015.1078182
- Lopes, L., Oliva, M., Fernandes, M., Pereira, P., Palma, P., & Ruiz-Fernández, J. (2018). Spatial distribution of morphometric parameters of glacial cirques in the Central Pyrenees (Aran and Boí valleys). Journal of Mountain Science, 15(10), https://doi.org/10.1007/s11629-018-4873-x
- López-Moreno, J. I., Revuelto, J., Rico, I., Chueca-Cía, J., Julián-Andrés, A., Serreta, A., Serrano, E., Vicente-Serrano, S. M., Azorín-Molina, C., Alonso-González, E., & García-Ruiz, J. M. (2016). Thinning of the Monte Perdido glacier in the Spanish Pyrenees since 1981. The Cryosphere, 10(2), 681–694. https://doi.org/10.5194/tc-10-681-2016
- Martí-Bono, C., & García-Ruiz, J. M. (1994). El glaciarismo surpirenaico: Nuevas aportaciones. Geoforma Ediciones.
- Martínez, F. J., Dietsch, C., Aleinikoff, J., Cirés, J., Arboleya, M. L., Reche, J., & Gómez-Gras, D. (2016). Provenance, age, and tectonic evolution of Variscan flysch, southeastern France and northeastern Spain, based on zircon geochronology. Geological Society of America Bulletin, 128(5-6), 842–859. https://doi.org/10.1130/B31316.1
- Martínez-Rius, A. (2010). Parque Nacional de Aigüestortes i Estany de Sant Maurici. Guía geológica. Editorial Everest.
- Martí-Soler, M. (1988). Estudi geomorfològic del massis central de la Vall d’Aran (Pirineu Central) [Bachelor thesis]. University of Barcelona.
- Matsuoka, N., Ikeda, A., & Date, T. (2005). Morphometric analysis of solifluction lobes and rock glaciers in the Swiss Alps. Permafrost and Periglacial Processes, 16(1), 99–113. https://doi.org/10.1002/ppp.517
- Mayewski, P. A., Rohling, E. E., Stager, J. C., Karlén, W., Maasch, K. A., Meeker, L. D., Meyerson, E. A., Gasse, F., van Kreveld, S., Holmgren, K., Lee-Thorp, J., Rosqvist, G., Rack, F., Staubwasser, M., Schneider, R. R., & Steig, E. J. (2004). Holocene climate variability. Quaternary Research, 62(3), 243–255. https://doi.org/10.1016/j.yqres.2004.07.001
- Mezger, J. E., & Gerdes, A. (2016). Early Variscan (Visean) granites in the core of central Pyrenean gneiss domes: Implications from laser ablation U-Pb and Th-Pb studies. Gondwana Research, 29(1), 181–198. https://doi.org/10.1016/j.gr.2014.11.010
- Oguchi, T., Yuichi, H., & Thad, W. (2012). Data sources. In S. Mike, P. Paolo, & G. James (Eds.), Geomorphological mapping methods and applications (pp. 189–224). Elsevier.
- Oliva, M., Fernandes, M., Palacios, D., Fernández-Fernández, J. M., Schimmelpfennig, I., Team, A., & Antoniades, D. (2021). Rapid deglaciation during the Bølling-Allerød Interstadial in the Central Pyrenees and associated glacial and periglacial landforms. Geomorphology, 107735, https://doi.org/10.1016/j.geomorph.2021.107735
- Oliva, M., Palacios, D., Fernández-Fernández, J. M., Rodríguez-Rodríguez, L., García-Ruiz, J. M., Andrés, N., Carrasco, R. M., Pedraza, J., Pérez-Alberti, A., Valcárcel, M., & Hughes, P. (2019). Late quaternary glacial phases in the Iberian peninsula. Earth-Science Reviews, 192, 564–600. https://doi.org/10.1016/j.earscirev.2019.03.015
- Oliva, M., Žebre, M., Guglielmin, M., Hughes, P. D., Çiner, A., Vieira, G., Bodin, X., Andrés, N., Colucci, R. R., García-Hernández, C., Mora, C., Nofre, J., Palacios, D., Pérez-Alberti, A., Ribolini, A., Ruiz-Fernández, J., Sarıkaya, M. A., Serrano, E., Urdea, P., Valcárcel, M., Woodward, J. C., & Yıldırım, C. (2018). Permafrost conditions in the Mediterranean region since the Last Glaciation. Earth-Science Reviews, 185, 397–436. https://doi.org/10.1016/j.earscirev.2018.06.018
- Palacios, D., García-Ruiz, J. M., Andrés, N., Schimmelpfennig, I., Campos, N., Léanni, L., Aumaître, G., Bourlès, D. L., & Keddadouche, K. (2017). Deglaciation in the central Pyrenees during the Pleistocene–Holocene transition: Timing and geomorphological significance. Quaternary Science Reviews, 162, 111–127. https://doi.org/10.1016/j.quascirev.2017.03.007
- Paron, P., & Claessens, L. (2011). Makers and users of geomorphological maps. In M. J. Smith, P. Paron, & J. S. Griffiths (Eds.), Geomorphological mapping methods and applications (Vol. 15, pp. 75–106). Elsevier B.V. https://doi.org/10.1016/B978-0-444-53446-0.00004-5
- Parry, S. (2011). The Application of geomorphological mapping in the assessment of landslide hazard in Hong Kong. In M. J. Smith, P. Paron, & J. S. Griffiths (Eds.), Geomorphological mapping methods and applications (pp. 413–442). Elsevier B.V.
- Pellitero, R. (2014). Geomorphology and geomorphological landscapes of Fuentes Carrionas. Journal of Maps, 10(2), 313–323. https://doi.org/10.1080/17445647.2013.867822
- Perdices Cos, P. (2019). Análisis de un deslizamiento en el Valle de Arán y simulación mediante el método del punto. Polytechnic University of Catalunha.
- Quesada, C., & Oliveira, J. (2019). The geology of Iberia: A geodynamic approach: The Variscan cycle. Springer.
- Rapp, A. (1960). Talus slopes and mountain walls at Tempelfjorden, Spitsbergen. Skrifter, 125.
- Schneider, R. A. A., Blomdin, R., Fu, P., Xu, X. K., & Stroeven, A. P. (2021). Paleoglacial footprint and fluvial terraces of the Shaluli Shan, SE Tibetan Plateau. Journal of Maps, https://doi.org/10.1080/17445647.2021.1946443
- Serrano, E., González-Trueba, J. J., & Sanjosé, J. (2011). Dynamic, evolution and structure of Pyrenean rock glaciers. Cuadernos de Investigación Geográfica, 37, https://doi.org/10.18172/cig.1260
- Serrano, E., López-Moreno, J. I., Gómez-Lende, M., Pisabarro, A., Martín-Moreno, R., Rico, I., & Alonso-González, E. (2020). Frozen ground and periglacial processes relationship in temperate high mountains: A case study at Monte Perdido-Tucarroya area (The Pyrenees, Spain). Journal of Mountain Science, 17(5), 1013–1031. https://doi.org/10.1007/s11629-019-5614-5
- Serrat, D., Martí, M., & Bordonau, J. (1994). Geologia, geomofologia e risques, em: Geografia física, em: Atlas comarcau de catalunya-Val d’Aran. Inst. Cart. Catalunya and Conselh Generau d'Aran, Val d'Aran.
- Sitter, L. U., & Zwart, H. J. (1962). Geological map of the Paleozoic of the Central Pyrenees. Leidse Geologische Mededelingen, 33, 191–254.
- Tejedor-Rodríguez, C., Moreno-García, M., Tornero, C., Hoffmann, A., García-Martínez de Lagrán, Í, Arcusa-Magallón, H., Garrido-Pena, R., Royo-Guillén, J. I., Díaz-Navarro, S., Peña-Chocarro, L., Alt, K. W., & Rojo-Guerra, M. (2021). Investigating Neolithic caprine husbandry in the Central Pyrenees: Insights from a multi-proxy study at Els Trocs cave (Bisaurri, Spain). PLoS One, 16(1), e0244139. https://doi.org/10.1371/journal.pone.0244139
- Victoriano, A., García-Silvestre, M., Furdada, G., & Bordonau, J. (2016). Long-term entrenchment and consequences for present flood hazard in the Garona River (Val d’Aran, Central Pyrenees, Spain). Natural Hazards and Earth System Sciences, 16(9), 2055–2070. https://doi.org/10.5194/nhess-16-2055-2016
- Wanner, H., Mercolli, L., Grosjean, M., & Ritz, S. P. (2015). Holocene climate variability and change; a data-based review. Journal of the Geological Society, 172(2), 254–263. https://doi.org/10.1144/jgs2013-101
- Zwart, H. J. (1979). The geology of the Central Pyrenees. Leidse Geologische mededelingen, 90(1), 1–74.