ABSTRACT
This paper focuses on Deep-Seated Gravitational Slope Deformations (DSGSDs) identified in the Molise region (Italy), along the central-southern sector of the Apennines chain. These phenomena were detected and mapped through a combination of aerial-photo interpretation, morphological analyses, and field surveys, integrated through satellite SAR interferometry (InSAR). The obtained general map is organized in different sections: inventory map with identification of DSGSDs and main geological and orographic features, geostructural scheme, representative cross sections, list and characteristics of DSGSDs, geomorphometric maps, landslides map, and seismotectonic setting. This study allowed us to realize a complete inventory of DSGSDs in the Molise region and define the main characteristics of each phenomenon. Through this research, it was possible to establish a general correlation between the analyzed deformations and the geological and geomorphological setting of the region as well as with its geodynamic context.
1. Introduction
Deep-Seated Gravitational Slope Deformations (DSGSDs) are slope-scale (volumes up to km3) processes evolving on very significant time scales (i.e. order of magnitude up to 103–105 years), and widespread in several mountain ranges in the world (CitationDiscenza & Esposito, 2021; CitationPánek & Klimeš, 2016). These phenomena occur in a variety of geomorphological and geological settings, both exerting a strong control on the onset and development of the process along with the morpho-evolutionary frame (e.g. CitationAgliardi et al., 2012; CitationBianchi Fasani et al., 2011; CitationDelchiaro et al., 2019, Citation2021, Citation2022; CitationHou et al., 2014; CitationJarman & Harrison, 2019; CitationMartino et al., 2017).
DSGSDs can be referred to creep phenomena (CitationChigira, 1992; CitationHutchinson, 1988), which involve time-dependent visco-plastic deformations that can accumulate and lead the rock masses to undergo gradual damage with associated loss of mechanical strength and stiffness until reaching subcritical conditions of rupture. DSGSDs are strictly controlled by the presence and geometry of inherited and pre-existing elements (CitationDiscenza et al., 2020), such as folds, faults, bedding planes, and main discontinuities.
Distinctive features of DSGSDs are low displacement rates but large cumulative displacements, as well as typical associated landforms such as double ridges, ridge-top depressions, scarps, uphill-facing scarps, fractures, and toe-slope bulging (CitationDiscenza & Esposito, 2021). They can also interact with the morphodynamics of stream network, influencing the catchment morphoevolution on both short and long timescales. Channel deviations, knickpoints, stream terraces, and impoundments can be associated with DSGSDs (CitationSavelli et al., 2013).
A growing interest in the understanding of such processes has been recorded in the last few decades and further efforts should be made to better address research on DSGSDs in terms of related hazard and risk assessment (CitationBianchi Fasani et al., 2014; CitationMartino et al., 2020; CitationPánek & Klimeš, 2016). It is possible to define two main types of risk induced by DSGSDs, namely direct and indirect. The direct risk is associated with the damage of the structures by the deformation, while the indirect risk is related to the secondary landslides that often occur in correspondence with the DSGSDs. Furthermore, recent studies (CitationGuerriero et al., 2021; CitationMartino et al., 2020) highlighted how the superimposition of even thick deforming and damaged rock masses over a less disturbed bedrock can imply the amplification of seismic motion over areas as wide as the extent of the process.
In this regard, the systematic recognition and inventorying of slopes affected by DSGSDs is the first fundamental step in view of a proper hazard zoning. Due to their potential evolution as massive failures, a complete hazard evaluation cannot leave aside the knowledge of the state of activity in terms of displacement rates over an enough wide time span and – in more general terms – the degree of maturity of the process, which is in turn related to the morpho-evolutionary rates.
Gravity-induced slope deformations have been observed in a wide variety of geological settings, geomorphological environments, and morpho-climatic conditions, especially in tectonically active areas (e.g. see CitationDiscenza & Esposito, 2021; CitationPánek & Klimeš, 2016 for a review). Central and southern Apennines show numerous and well-known DSGSDs (e.g. CitationBianchi Fasani et al., 2011, Citation2014; CitationBozzano et al., 2013; CitationDella Seta et al., 2017; CitationDi Luzio et al., 2004; CitationDi Luzio et al., 2022; CitationDi Martire et al., 2016; CitationEsposito et al., 2007; CitationEsposito et al., 2021; CitationGaladini, 2006; CitationGori et al., 2014; CitationMartino et al., 2004, Citation2020), sometimes partially related to active faults and seismic shaking (CitationMoro et al., 2007, Citation2012).
This paper reports the preliminary results of a wider research project aimed at investigating the kinematics of DSGSDs in the Apennine belt and the role of conditioning geo-thematic factors on their onset and development. Although the research is so far being carried out in the Molise region () featured by high proneness to landslides (CitationBorgomeo et al., 2014; CitationMartino et al., 2020), only few studies focused on DSGSDs (CitationCorniello & Santo, 1994; CitationDiscenza et al., 2009, Citation2011). This region, in a relatively small territory, includes a large variety of geological and geomorphological environments, with peculiar litho-structural and morphostructural features in the central-southern Apennines.
Figure 1. Location map of the study area and the Molise region, produced with 5 m/px digital elevation model (DEM) superposed on Molise hillshade.
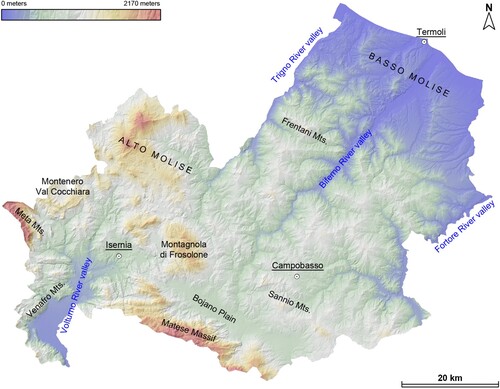
Specifically, we (i) inventoried, classified, and mapped DSGSDs landforms; (ii) collected and elaborated geo-thematic data (i.e. geological, geomorphological, and structural data); (iii) collected and processed satellite SAR data. Indeed, although several limits affect the ability of the interferometric technique to monitor DSGSDs transposing a decennial observation on an extremely longer process, the interferometric technique enhanced the identification of the slope accessory landforms, which testify to a wider landslide system (e.g. CitationDelchiaro et al., 2021, Citation2022). Moreover, the overlay of the inventory and the geo-thematic data allowed us to perform a preliminary exploration of the relationships among some selected geo-related variables, referred to as predisposing factors, and the presence and type of DSGSDs.
2. Geological, geomorphological, and seismological setting
Different geological domains are distinguished in the Molise region. Its Meso-Cenozoic paleogeography () was characterized by carbonate platforms, transitional zones, and marine basins (CitationMostardini & Merlini, 1986; CitationPatacca et al., 1992; CitationPatacca & Scandone, 2007; CitationVezzani et al., 2010).
Figure 2. Paleogeographic domains of central and southern Apennines including the Molise area (redrawn from CitationVezzani et al., 2010).
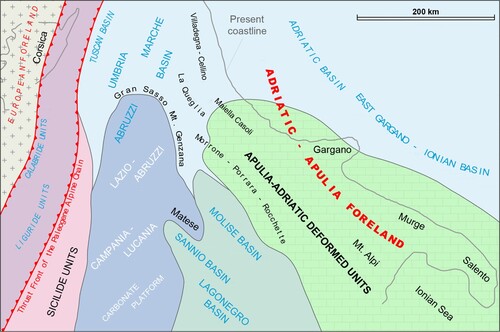
To the southwest, the Matese Massif is made by a sequence of Upper Triassic–Upper Cretaceous shallow-water carbonates overlaid by Lower to Middle Miocene ramp deposits, followed upwards by Upper Tortonian–Lower Messinian flysch (CitationFerranti et al., 2015; CitationScrocca & Tozzi, 1999). A proximal transitional sequence crops out in the Montagnola di Frosolone area, Venafro Mts., and Montenero Val Cocchiara area: Jurassic-Middle Miocene biodetritic limestones are interlayered with subordinate marls and cherty limestones and closed upwards by Lower Messinian flysch deposits (CitationAntonucci et al., 2000; CitationDe Corso et al., 1998).
During the Mio-Pliocene orogenesis (CitationCorrado et al., 1997), the transitional sequence was deformed in wide anticlines and large dome-like structures developed in the hanging wall of main thrusts. Lithological and structural inheritance gave rise to high mountain ranges with large summit areas and flanks having variable steepness. In the south and south-west ridge boundaries, topography and energy relief were increased by the morphotectonic activity of Plio-Quaternary normal and transtensional faults (CitationCorrado et al., 1997; CitationDi Bucci et al., 1999).
Distal basin facies become predominant moving north in the Alto Molise area (CitationCorrado et al., 1998; CitationDi Bucci et al., 1999). The bottom of the geological sequence is here characterized by the Argille Varicolori Fm. (Varicoloured Clays, Late Oligocene-Early Miocene in age), overlayered by a few hundred meters-thick interval of Middle Miocene biodetritic limestones, marly limestones, and marls passing upwards to lower Messinian clays and sandstones (flysch). In the north-eastern sectors of the region, i.e. in the Frentani Mts. () the Middle Miocene interval contains a much-reduced amount of carbonate clastic deposits (CitationPatacca et al., 1992).
The morphological evidence of these basinal sequences is a low-topography, hilly landscape dominated by large outcrops of clays separated by narrow, elongated, N–S or NW–SE-oriented ridges which resulted from Miocene thrusting. In the reason of mechanical anisotropies within the basin sequences, several fault-propagation folds developed, later overriding the flysch deposits through breakthrough thrusts (CitationDi Luzio et al., 1999). Steep slopes then originated due to high-dipping or overturned strata in fold forelimbs.
The central and south-eastern sectors of the Molise region are characterized by an Upper Cretaceous–Miocene deep basin sequence, named as Sicilidi (CitationVezzani et al., 2004) or Sannio units (CitationPatacca et al., 1992) and which is composed, from the bottom to the top, of detritic limestones, marls, varicolored clays, and sandstones (CitationCesarano et al., 2011). Post-orogenic, Plio-Quaternary sequences unconformably cover the Molise thrust belts along the peri-Adriatic region and are found within intra-mountain basin/valleys (CitationPatacca & Scandone, 2007).
Quaternary tectonics in the region is featured by normal faults with a main NW–SE orientation, such as in the Boiano-Isernia continental basins extending in the axial zone of the belt (CitationCorrado et al., 1997; CitationDi Bucci et al., 1999, Citation2005). Since the Early-Middle Pleistocene transition, localized extensional faulting was contemporaneous with a process of regional uplift that shaped the present morphology of the region (CitationAmato et al., 2017).
Central and southern Apennines are among the sectors of the Italian peninsula with the highest seismic potential, with recurrence times for M ≥ 6.5 events between 60 and 140 years (CitationJenny et al., 2006). This area has been affected by numerous historical events with an epicentral intensity > X (CitationChiarabba et al., 2010). In the Molise region, several destructive earthquakes were recorded in the last centuries, such as that of 1456, 1627, and 1805 (CitationRovida et al., 2016). Recently, some important earthquakes struck this region causing damage and reactivating landslides with varying characteristics, as in the case of 2002 (Mw 5.8) San Giuliano di Puglia earthquake, or 2018 (Mw 5.1) Montecilfone earthquake (CitationMartino et al., 2020).
3. Materials and methods
As previously stated, this paper refers to the first phases of a research project aimed at providing a preliminary hazard zonation and the basic information for further and advanced analyses. Such basic information derives from the collection, analysis, and mapping of relevant data: from the identification and inventorying of DSGSDs to the assessment of their displacement rates and the extraction of geo-thematic factors (i.e. morphological variables and lithological/structural conditions) potentially controlling the deformational phenomenon. Finally, a data fusion process has been performed by overlaying the information previously collected and elaborated to provide the first hints on the causative relations among geo-thematic parameters and DSGSDs presence and kinematics by means of a basic exploratory spatial data analysis.
3.1. DSGSDs inventory
To detect DSGSDs-related landforms, analyses of optical images, interferometric data, and field surveys on some selected cases were performed. Aerial-photo interpretation was carried out on stereo-pairs and ortho-photos at different resolutions and covering a wide time span provided by the Ministry of Environment (years 1954 and 1987) and Molise region (years 1992, 2002, and 2007). A geographic database in a GIS environment (QGIS release 3.24) has been conceived and set up to store the geometry and attributes of the investigated phenomena. DSGSDs were mapped as polygons whose extent envelopes the slope sections featured by patterns of specific landforms.
The main information contained in the related attribute table refer to the classification of phenomena, grouped in three main macro-categories according to CitationDiscenza and Esposito (2021): sackungen, spreadings, and ‘complex type mechanisms’ (). The sackung is characterized by a significant vertical component of the deformation in the upper part of the slope, which is also associated with horizontal displacements in the middle-lower portion of the relief, while the spreading is characterized by a prevalent horizontal component. Finally, the complex type refers to DSGSDs characterized by superimposition in space and time of the aforementioned typologies of deformation.
Figure 3. Examples of different types of DSGSDs mapped in Molise region (for location see the Inventory map): (a) sackung at Mt. Patalecchia (ID: 56), in the NW sector of Matese Massif; (b) sackung at Mt. Pietrereie (ID: 44), in the SW sector of Montagnola di Frosolone; (c) lateral spreading at Mt. Pizzi (ID: 46), in the Alto Molise sector; (d) rock mass spreading at Mt. Rocchetta (ID: 13), in the eastern sector of Meta Mts.; (e) complex type mechanism at Mt. Matese (ID: 74), along the NE margin of Matese Massif. All the images were produced with Google Earth.
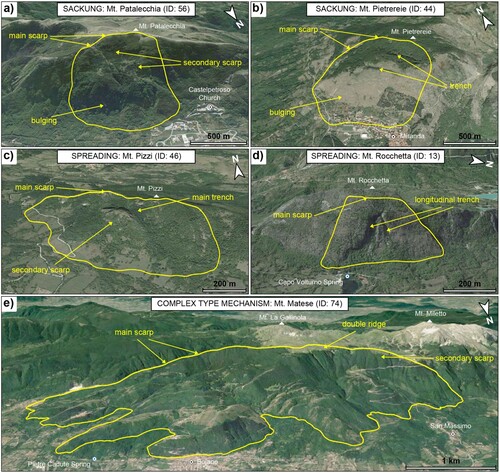
3.2. Geo-thematic database and maps
We first acquired, digitized and georeferenced the 1:100,000 scale geological map of Molise region by CitationVezzani et al. (2004): the resulting polygons referred to geological units/formations and lines representing main tectonic elements have been then stored in a geographic database (GDB). Based on the original description, the geological units/formations were grouped according to a pure lithologic criterion that indirectly accounts for mechanical properties.
Furthermore, GDB has been integrated with topography retrieved by the 5 m/px digital elevation model (DEM) and the 1:5000 scale topographic map provided by the Molise region (https://sciamlab.com/opendatahub/it/organization/r_molise). DEM-derived terrain analyses (slope, aspect, and local relief) were computed using QGIS and MATLAB-based TopoToolbox (CitationSchwanghart & Scherler, 2014). In detail, local relief quantifies the elevation range within a specific radius (500 m), and it was computed using ‘localtopography’ function of TopoToolbox.
Finally, in view of further analyses, a landslide density map and a seismotectonic map were produced. The former derives from the 22,500 landslide processes reported in the IFFI Project (CitationAPAT, 2007): the heat map was produced with QGIS, through a Kernel density function on a 2-km fixed radius. For the seismotectonic map, Individual Seismogenic Sources from DISS catalogue (version 3.3.0; 2021) were used (https://diss.ingv.it/download-diss-3-3-0). Plots were made with MATLAB release 2021b.
3.3. Interferometric analysis and displacement maps
Interferometric analyses () were carried out using data available on the National Cartographic Portal of the Ministry of the Environment (http://www.pcn.minambiente.it) acquired as part of the Not-ordinary Plan of Environmental Remote Sensing. Data from the European Space Agency's ERS1/2 and ENVISAT satellites were used for the periods 1992–2000 and 2002–2010 respectively. In addition, data from the COSMO-SkyMed constellation of the Italian Space Agency were used for the period 2011–2014. Data were acquired in the two acquisition geometries (ascending and descending).
Figure 4. Line of Sight (LoS) mean displacement rate maps: (a) ERS ascending; (b) ERS descending; (c) ENVISAT ascending; (d) ENVISAT descending; COSMO-SkyMed ascending; (f) COSMO-SkyMed descending.
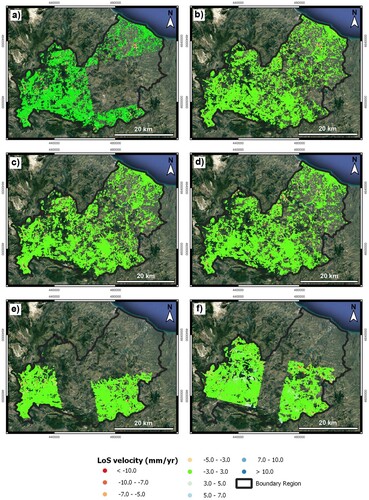
To identify slope sectors characterized by significant displacement rates, the procedure for identifying anomalous areas was applied as described by CitationMeisina et al. (2008). A cluster analysis was carried out. Such post-processing allows to identify, within fishnet grid, possible ‘anomalous’ areas where similar displacement rate values tend to be clustered, by defining an ‘Homogeneity Index’ (HI) which is the ratio between the number of moving targets and the total number of targets within each pixel of fishnet. Only pixels of fishnet grid with at least 50% of ‘moving’ targets inside have been considered as unstable, thus obtaining a map of stable/unstable pixels. Such an approach was implemented in a GIS-semiautomatic routine to a preliminary analysis over large areas by creating easy-to-read anomaly maps.
4. Results
The presented map shows the inventory of 80 DSGSDs identified in the Molise region, distinguished in 42 sackungen, 33 spreadings, and 5 complex type mechanisms. It contains seven sections, described in the following paragraphs.
4.1. Inventory map
In the main map the 80 DSGSDs identified and mapped in the Molise region are reported, distinguished in relation to their type of mechanism. The inventory is supported by a geological-technical map which highlights the main lithostratigraphic units outcropping in the study area, as well as the main tectonic elements. The latter are divided into normal or strike-slip faults and reverse faults or thrusts. All the aforementioned themes are shown on a hillshade useful for a better comprehension of the main morphological characteristics of the territory.
The mapped DSGSDs are not homogeneously distributed throughout the territory. Most of the phenomena are found in the western and southern sectors of the region, or rather in the mountainous areas where predominantly calcareous, dolomitic, calciruditic, and calcareous-marly lithological units crops out. Only three DSGSDs are present in the NE sector of the Molise region, along the NW sector of the Frentani Mts.
The types of mechanisms also reveal a close relationship with the morphological and geological setting of the territory. Sackungen are found mainly in the southern and western portions of the region, where the most important and extensive carbonate reliefs are present. Instead, spreadings are mainly represented in the north-western portion of the study area, i.e. where thin carbonate sequences are superimposed on pelitic terms with more ductile behavior. Complex mechanisms are very few and located in the central portion of the area, in correspondence with the carbonate structures of Montagnola di Frosolone and the NE margin of the Matese Massif.
The distribution of DSGSDs over the study area is reflected in the frequency of the involved lithologies (). Statistical analyses show that these processes mainly involve limestone and dolostone rocks, while other lithologies are only subordinately affected. Peculiar is the distribution for complex movements, for which there is a very clear prevalence of calcareous-dolomitic rocks, while sackungen are the processes with the greatest dispersion in terms of involved lithotypes. Although this distribution highlights some specific characteristics of the analyzed DSGSDs, the type of mechanism is only partially controlled by the outcropping rocks, whereas the geological–structural setting assumes greater importance.
Figure 5. Statistical distribution of DSGSDs in relation to lithological units. (A) Undifferentiated marine, transitional, and continental Quaternary deposits; (B) Sands, gravels, and conglomerates, with intercalations of calcarenites, sandstones, and micro-conglomerates; (C) Clays, silty clays, marls, and sands, with intercalations of sandstones, conglomerates, calcarenites, and gypsum; (D) Varicolored clays, silty clays, and marly clays, with olistoliths and intercalations of marls, limestones, and radiolaritis; (E) Marls, calcarenites, and calcilutites, with intercalations of clays, marly clays, calcirudites, and marly limestones; (F) Calcirudites and calcarenites, with intercalations of conglomerates and marly limestones; (G) Calcilutites, micritic limestones, and dolostones; detritic limestones, cherty limestones, and marly limestones.
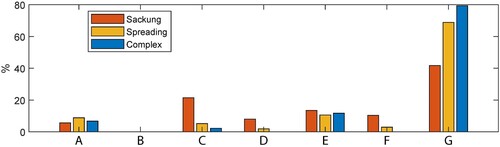
4.2. Location map and geostructural scheme
In this section, the geo-localization of the study area and the main geological–structural characteristics of this sector of the central-southern Apennines are reported. In particular, the geostructural scheme of CitationVezzani et al. (2004) was redrawn and used to better describe the peculiar features of the Molise region.
4.3. Representative cross-sections
In this part, two cross-sections representative of the main types of deformations present in the study area are reported. One section is representative of sackung phenomena (Mt. Pesche dell’Acqua DSGSD), while the other is representative of spreading phenomena (Mt. Pizzi and Mt. La Penna DSGSDs). No section has been produced for complex mechanisms, as precisely because of the considerable variability of the phenomena it is not possible to define schemes that can represent general characteristics and conditions.
Sackung mechanisms are characterized by moderately dipping slopes, consisting mainly of rigid calcareous-dolomitic and calcareous-marly rocks; there are no significant rheological contrasts, while high-angle tectonic elements can affect slopes favoring and controlling the development of such phenomena. Spreading mechanisms are instead characterized by slightly steep slopes, consisting of rigid calcareous-dolomitic and calcareous-marly rocks superimposed (tectonically or stratigraphically) to pelitic successions with ductile behavior; rheological contrasts are therefore very relevant, while tectonic elements are predominantly at low angles.
4.4. Type and morphometric characteristics
In the table is reported a list of the mapped DSGSDs and their major characteristics. The phenomena are ordered with West-to-East progressive ID numbers and named by a reference toponym. DSGSDs are classified according to the previous described scheme. These general data are supported by the major morphological and morphometrical characteristics of phenomena, such as area, H/L and W/L ratios, and mean slope, aspect, and relief. For these variables, an extensive summary is reported in the geomorphometric section paragraph.
4.5. Geomorphometric maps
In this section, the maps used for the definition of the main morphological and morphometric variables are reported. The morphometric features (and related variability) of the inventoried DSGSDs (), distinguished by mechanism, are shown as boxplots for area, H/L and W/L ratios, mean slope, aspect, and relief variables. On each box, the central mark indicates the median, and the bottom and top edges of the box indicate the 25th and 75th percentiles, respectively; whiskers extend to the most extreme data points not considered as outliers, which are plotted individually. In , the corresponding values are reported.
Figure 6. Boxplots derived for the morphometric variables of DSGSDs. The bar plot is shown for aspect factor.
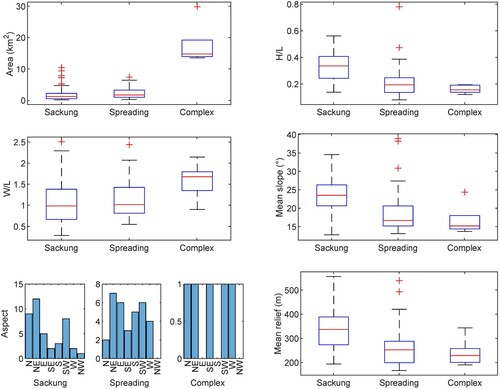
Table 1. Morphometric statistical variables of Molise DSGSDs.
In terms of the involved area, the distribution of complex mechanisms (13.5–29.8 km2) is characterized by one order of magnitude greater than the values of sackungen (0.2–10.4 km2) and spreadings (0.3–7.4 km2). Regarding height/length ratio (H/L), sackungen dispersion is generally greater than spreadings and complex ones, while regarding deformation width/length ratio (W/L), sackung and spreading mechanisms show a similar distribution, with values lower than complex phenomena.
Regarding slope angle values, the distribution for sackung mechanisms is concentrated between 12.8° and 34.6°, while spreadings and complex mechanisms show lower values. Aspect values instead show a strong correspondence between the distributions of each typology of DSGSD. Specifically, values are concentrated towards N, NE, E, and SW. The distribution of relief energy shows that values for sackungen are generally higher (555–194 m) in comparison with those of spreadings (539–167 m) and complex mechanisms (343–190 m).
4.6. Landslides section
The present map highlights the conventional landslides inventoried in the IFFI Project (CitationAPAT, 2007) and the related heat map, realized using a Kernel density function on a 2 km fixed radius. The concentration was calculated using the centroids of the inventoried landslides. Indeed, although a density based on the number of landslides can underestimate wide or diffuse landslide mechanisms, such as falls and topples, it makes sense in relation to the regional scale of the study, whereby the variability in the size of the phenomena is not very large.
In general, a close correspondence between DSGSDs and conventional landslides is not observed, except in local cases. Sackungen and complex mechanisms are the least correlated, especially as they involve rock masses that are not subject to the onset of landslides and small gravity-induced processes. Falls and topples, generally correlated to these types of DSGSDs, are registered in this database as extensive areas subject to instability and, therefore, the use of the centroid is not able to highlight particular concentrations.
Spreadings are often located in sectors with a fair concentration of conventional landslides, even if significant increases in conventional mass movements are not visible in correspondence with these phenomena. With few exceptions, the IFFI database does not highlight the increase in secondary, peripherical landslides that is often described in the literature in association with DSGSDs. Although it should also be emphasized that the results of the heat map are influenced by the census methods of the phenomena and by the accuracy of the inventory. In some cases, few and large landslides can produce a low concentration that does not correctly describe the local geomorphological conditions.
4.7. Seismotectonic section
This map shows the censed DSGSDs and the main seismogenic structures, as indicated in the Individual Seismogenic Sources from DISS catalogue (version 3.3.0; 2021). Specifically, the seismogenetic sources are distinguished into single and composite.
With respect to these elements, the distribution of large-scale gravity-induced slope deformations is rather uneven, as it does not follow a univocal and identifiable pattern. Many DSGSDs fall outside seismogenic structures and have no spatial correlation with them. This condition is particularly evident for spreading phenomena, which generally are put outside the aforementioned structures with few exceptions. Different is the condition of sackungen, which are instead partially correlated with some seismogenic structures. Complex mechanisms are the ones most evidently correlated with the seismogenic structures, as they are all placed in correspondence with one of these or, at least, in the immediate surroundings.
5. Discussion
The study allowed us to identify and map 80 DSGSDs, with variable characteristics and dimensions. Each phenomenon was coded and classified according to its mechanism into three main types. Through the analysis of morphological data, the main morphometric variables of each DSGSD were defined, to derive the geometric characteristics of the three types of movements. DSGSDs show a peculiar distribution, as they are mainly concentrated in the south-western portion of Molise region, along the axial sector of the Apennine belt. Sackungen are mainly distributed along the major carbonate ridges (such as Matese Massif and Venafro Mts.), while spreadings are more diffuse in the NW sector of the studied region (where peculiar geological conditions are present). Complex mechanisms are few and limited to some huge, very large phenomena affecting the NE sector of the Matese Massif and the whole Montagnola di Frosolone.
The study and census of the DSGSDs in the Molise region made it possible to preliminarily discriminate the main morphometric characteristics of the phenomena based on the data preliminary collected here. As it can be seen from the matrix plot (), some correlations between the DSGSD morphometric variables output. There is a direct proportionality relationship between mean slope, H/L ratio, and mean relief variables, while the relation described by landslides area and the other variables shows an exponential distribution. By comparing these relationships with the distribution of the values for each mechanism for each variable, it results that sackungen develop more with dipper slopes, with higher relief and H/L ratio than with spreadings or complex mechanisms.
Figure 7. Plot matrix derived for the morphometric variables of DSGSDs. The analyses were conducted considering the three types of slope deformations mapped in the study area.
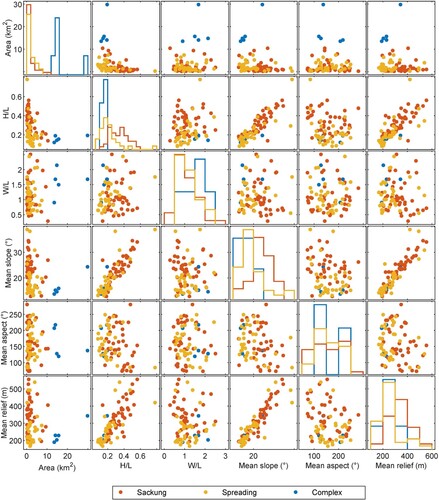
Finally, to analyze the evolutionary stages and/or kinematic conditions characteristics of the mapped DSGSDs, interferometric analyses were carried out on the entire study area (). Interferometric data analysis identified anomalous areas within 53 of the 80 DSGSDs, thus about 66%: 4 of the 5 phenomena for complex mechanism, 27 of the 42 for sackungen, and 22 of the 33 for spreading. The remaining unidentified phenomena are probably due to the complete absence of natural reflectors (rocks, structures, etc.) or sometimes to the rather small size of the phenomena themselves.
6. Conclusion
Molise region shows the typical geological and geomorphological features of the Apennine belt, due to a complex Mesozoic paleogeography, style of deformation during Miocene orogenesis, and regional post-early Pleistocene uplift. The whole area is affected by a high number of gravity-induced slope deformations as well as landslide processes, although up to now there have been very few studies conducted on DSGSDs in the region. For these reasons, a wide census/inventory was conducted in this sector of the central-southern Apennines to identify the main large-scale gravitational phenomena.
This study resulted in the mapping of 80 DSGSDs, variously distributed throughout the territory. Each phenomenon was classified in terms of the type of movement and analyzed with respect to the main morphological and morphometric characteristics. Preliminarily, DSGSDs were correlated with the main geological, geomorphological, and seismotectonic characteristics of the study area. This dataset will allow, in the subsequent study phases, to correlate large-scale gravity-induced phenomena with the inherited predisposing factors that control their diffusion and development. It is worth stressing that the inventory itself represents a first-level (spatial) hazard zoning for this kind of processes.
Software
The vector/raster data and main map were managed using QGIS 3.24 ®, with final editing performed using Adobe Illustrator ®. DEM-derived geomorphic variables were computed using QGIS 3.24 ® and MATLAB-based TopoToolbox (CitationSchwanghart & Scherler, 2014). Plots were made with MATLAB release 2021b.
Supplemental Material
Download Zip (42.2 MB)Data availability statement
The inventory of ‘Deep-Seated Gravitational Slope Deformations (DSGSDs) and main geomorphological features of Molise region (Italy)’ is stored at the link https://doi.org/10.5281/zenodo.7445688. Moreover, a web-GIS version of the database can be found at the Centro di ricerca, previsione e controllo dei rischi geologici (CERI) website https://gdb.ceri.uniroma1.it/index.php/view/.
Acknowledgments
The authors thank Geoservizi srl for the data and information provided. This study is part of the research project ‘Integrated analysis and hazard-oriented modeling of large-scale slope instabilities featured by Mass Rock Creep’ (scientific responsible Carlo Esposito) funded by Sapienza University of Rome. The Authors gratefully acknowledge the Editors Mike J. Smith and Chi-Wen Chen, and the reviewers Samuele Segoni, Su-Min Shen, and Cecilia Furlan for their suggestions that improved the manuscript.
Disclosure statement
No potential conflict of interest was reported by the author(s).
Additional information
Funding
References
- Agliardi, F., Crosta, G. B., & Frattini, P. (2012). Slow rock-slope deformation. In J. J. Clague, & D. Stead (Eds.), Landslides: Types, mechanisms and modeling (pp. 207–221). Cambridge University Press.
- Amato, V., Aucelli, P. P. C., Bracone, V., Cesarano, M., & Rosskopf, C. M. (2017). Long-term landscape evolution of the Molise sector of the central-southern Apennines, Italy. Geologica Carphatica, 68(1), 29–42. https://doi.org/10.1515/geoca-2017-0003
- Antonucci, A., De Corso, S., Di Luzio, E., Lenci, F., Sansonne, P., Scrocca, D., & Tozzi, M. (2000). La Montagnola di Frosolone ed il Matese settentrionale: nuovi dati sulla geologia molisana. Italian Journal of Geosciences, 119(3), 637–654.
- APAT. (2007). Report on landslides in Italy: the IFFI project. Methodology, results and regional reports. APAT Reports, 78, 681.
- Bianchi Fasani, G., Di Luzio, E., Esposito, C., Evans, S. G., & Scarascia Mugnozza, G. (2014). Quaternary, catastrophic rock avalanches in the central Apennines (Italy): Relationships with inherited tectonic features, gravity-driven deformations and the geodynamic frame. Geomorphology, 211, 22–42. https://doi.org/10.1016/j.geomorph.2013.12.027
- Bianchi Fasani, G., Di Luzio, E., Esposito, C., Martino, S., & Scarascia-Mugnozza, G. (2011). Numerical modelling of Plio-quaternary slope evolution based on geological constraints: A case study from the caramanico valley (Central Apennines, Italy). Geological Society, London, Special Publications, 351(1), 201–214. https://doi.org/10.1144/SP351.1
- Borgomeo, E., Hebditch, K. V., Whittaker, A. C., & Lonergan, L. (2014). Characterizing the spatial distribution, frequency and geomorphic controls on landslide occurrence, Molise, Italy. Geomorphology, 226, 148–161. https://doi.org/10.1016/j.geomorph.2014.08.004
- Bozzano, F., Bretschneider, A., Esposito, C., Martino, S., Prestininzi, A., & Scarascia Mugnozza, G. (2013). Lateral spreading processes in mountain ranges: Insights from an analogue modelling experiment. Tectonophysics, 605, 88–95. https://doi.org/10.1016/j.tecto.2013.05.006
- Cesarano, M., Pappone, G., Amato, V., Aucelli, P. P. C., Baranello, S., Cascella, A., Casciello, E., Ferrarini, F., Lirer, F., Monaco, R., & Rosskopf, C. (2011). Stratigrafia ed assetto geometrico dell’Unità del Sannio nel settore settentrionale dei monti del Matese. Rendiconti Online della Società Geologica Italiana, 12, 35–38.
- Chiarabba, C., Bagh, S., Bianchi, I., De Gori, P., & Barchi, M. (2010). Deep structural heterogeneities and the tectonic evolution of the Abruzzi region (Central Apennines, Italy) revealed by microseismicity, seismic tomography, and teleseismic receiver functions. Earth Planetary Science Letters, 295(3-4), 462–476. https://doi.org/10.1016/j.epsl.2010.04.028
- Chigira, M. (1992). Long-term gravitational deformation of rocks by mass rock creep. Engineering Geology, 32(3), 157–184. https://doi.org/10.1016/0013-7952(92)90043-X
- Corniello, A., & Santo, A. (1994). Geologia e fenomeni gravitativi profondi nell'area dell'alto corso del Fiume Trigno (Molise). Geologica Romana, 30, 67–74.
- Corrado, S., Di Bucci, D., Leschiutta, I., Naso, G., & Trigari, A. (1997). La tettonica quaternaria della piana d’Isernia nell’evoluzione strutturale del settore molisano. Il Quaternario, 10(2), 609–614.
- Corrado, S., Di Bucci, D., Naso, G., & Butler, R. W. H. (1997). Thrusting and strike-slip tectonics in the Alto Molise region (Italy): implications for the Neogene-Quaternary evolution of the Central Apennines orogenic system. Journal of the Geological Society, London, 154(4), 679–688. https://doi.org/10.1144/gsjgs.154.4.0679
- Corrado, S., Di Bucci, D., Naso, G., & Damiani, A. V. (1998). Rapporti tra le grandi unità stratigrafico-strutturali dell’Alto Molise (Appennino centrale). Italian Journal of Geosciences, 117(3), 761–776.
- De Corso, S., Scrocca, D., & Tozzi, M. (1998). Geologia dell’anticlinale del Matese e implicazioni per la tettonica dell’Appennino Molisano. Italian Journal of Geosciences, 117(2), 419–441.
- Delchiaro, M., Della Seta, M., Martino, S., Dehbozorgi, M., & Nozaem, R. (2019). Reconstruction of river valley evolution before and after the emplacement of the giant Seymareh rock avalanche (Zagros Mts., Iran). Earth Surface Dynamics, 7(4), 929–947. https://doi.org/10.5194/esurf-2018-91
- Delchiaro, M., Della Seta, M., Martino, S., Nozaem, R., & Moumeni, M. (2022). Tectonic deformation and landscape evolution inducing mass rock creep driven landslides: The Loumar case-study (Zagros Fold and Thrust Belt, Iran). Tectonophysics, 846, 229655. https://doi.org/10.1016/j.tecto.2022.229655
- Delchiaro, M., Mele, E., Della Seta, M., Martino, S., Mazzanti, P., & Esposito, C. (2021). Quantitative investigation of a mass rock creep deforming slope through A-Din SAR and geomorphometry. In V. Vilímek, F. Wang, A. Strom, K. Sassa, P. T. Bobrowsky, & K. Takara (Eds.), Understanding and reducing landslide disaster risk. WLF 2020. ICL contribution to landslide disaster risk reduction. Springer. https://doi.org/10.1007/978-3-030-60319-9_18
- Della Seta, M., Esposito, C., Marmoni, G. M., Martino, S., Scarascia Mugnozza, G., & Troiani, F. (2017). Morpho-structural evolution of the valley-slope systems and related implications on slope-scale gravitational processes: New results from the Mt. Genzana case history (central Apennines, Italy). Geomorphology, 289, 60–77. https://doi.org/10.1016/j.geomorph.2016.07.003
- Di Bucci, D., Corrado, S., Naso, G., Parotto, M., & Praturlon, A. (1999). Evoluzione tettonica neogenico-quaternaria dell’area molisana. Italian Journal of Geosciences, 118(1), 13–30.
- Di Bucci, D., Naso, G., Corrado, S., & Villa, I. M. (2005). Growth, interaction and seismogenic potential of coupled active normal faults (Isernia Basin, central-southern Italy). Terra Nova, 17(1), 44–55. https://doi.org/10.1111/j.1365-3121.2004.00582.x
- Di Luzio, E., Discenza, M. E., Di Martire, D., Putignano, M. L., Minnillo, M., Esposito, C., & Scarascia Mugnozza, G. (2022). Investigation of the Luco dei Marsi DSGSD revealing the first evidence of a basal shear zone in the Central Apennine belt (Italy). Geomorphology, 408, 108249. https://doi.org/10.1016/j.geomorph.2022.108249
- Di Luzio, E., Paniccia, D., Pitzianti, P., Sansonne, P., & Tozzi, M. (1999). Evoluzione tettonica dell’Alto Molise. Italian Journal of Geosciences, 118(2), 287–315.
- Di Luzio, E., Saroli, M., Esposito, C., Bianchi Fasani, G., Cavinato, G. P., & Scarascia Mugnozza, G. (2004). Influence of structural framework on mountain slope deformation in the Maiella anticline (Central Apennines, Italy). Geomorphology, 60(3–4), 417–432. https://doi.org/10.1016/j.geomorph.2003.10.004
- Di Martire, D., Novellino, A., Ramondini, M., & Calcaterra, D. (2016). A-Differential synthetic aperture radar interferometry analysis of a deep seated gravitational slope deformation occurring at Bisaccia (Italy). Science of The Total Environment, 550, 556–573. https://doi.org/10.1016/j.scitotenv.2016.01.102
- Discenza, M. E., & Esposito, C. (2021). State-of-art and remarks on some open questions about DSGSDs: Hints from a review of the scientific literature on related topics. Italian Journal of Engineering Geology and Environment, 21(1), 31–59. https://doi.org/10.4408/IJEGE.2021-01.O-03
- Discenza, M. E., Esposito, C., Martino, S., Petitta, M., Prestininzi, A., & Scarascia Mugnozza, G. (2011). The gravitational slope deformation of Mt. Rocchetta ridge (central Apennines, Italy): geological-evolutionary model and numerical analysis. Bulletin of Engineering Geology and the Environment, 70(4), 559–575. https://doi.org/10.1007/s10064-010-0342-7
- Discenza, M. E., Esposito, C., Martino, S., Petitta, M., & Scarascia Mugnozza, G. (2009). Modello geologico-tecnico ed analisi numerica con approccio equivalente continuo della deformazione gravitativa di Monte della Rocchetta (Appennino Centrale, Italia). Rendiconti Online della Società Geologica Italiana, 6, 225–226. https://doi.org/10.2139/ssrn.3938163
- Discenza, M. E., Martino, S., Bretschneider, A., & Scarascia Mugnozza, G. (2020). Influence of joints on creep processes involving rock masses: Results from physical-analogue laboratory tests. International Journal of Rock Mechanics and Mining Sciences, 128, 104261. https://doi.org/10.1016/j.ijrmms.2020.104261
- Esposito, C., Di Luzio, E., Baleani, M., Troiani, F., Della Seta, M., Bozzano, F., & Mazzanti, P. (2021). Fold architecture predisposing deep-seated gravitational slope deformations within a flysch sequence in the Northern Apennines (Italy). Geomorphology, 380, 107629. https://doi.org/10.1016/j.geomorph.2021.107629
- Esposito, C., Martino, S., & Scarascia Mugnozza, G. (2007). Mountain slope deformations along thrust fronts in jointed limestone: An equivalent continuum modelling approach. Geomorphology, 90(1-2), 55–72. https://doi.org/10.1016/j.geomorph.2007.01.017
- Ferranti, L., Milano, G., Burrato, P., Palano, M., & Cannavò, F. (2015). The seismogenic structure of the 2013-2014 Matese seismic sequence, Southern Italy: Implication for the geometry of the Apennines active extensional belt. Geophysical Journal International, 201(2), 823–837. https://doi.org/10.1093/gji/ggv053
- Galadini, F. (2006). Quaternary tectonics and large-scale gravitational deformations with evidence of rock-slide displacements in the central Apennines (central Italy). Geomorphology, 82(3–4), 201–228. https://doi.org/10.1016/j.geomorph.2006.05.003
- Gori, S., Falcucci, E., Dramis, F., Galadini, F., Galli, P., Giaccio, B., Messina, P., Pizzi, A., Sposato, A., & Cosentino, D. (2014). Deep-seated gravitational slope deformation, large-scale rock failure, and active normal faulting along Mt. Morrone (Sulmona basin, Central Italy): Geomorphological and paleoseismological analyses. Geomorphology, 208, 88–101. https://doi.org/10.1016/j.geomorph.2013.11.017
- Guerriero, L., Prinzi, E. P., Calcaterra, D., Ciarcia, S., Di Martire, D., Guadagno, F.M., Ruzza, G. & Revellino, P. (2021). Kinematics and geologic control of the deep-seated landslide affecting the historic center of Buonalbergo, southern Italy. Geomorphology, 394, 107961. https://doi.org/10.1016/j.geomorph.2021.107961
- Hou, Y. L., Chigira, M., & Tsou, C. Y. (2014). Numerical study on deep-seated gravitational slope deformation in a shale-dominated dip slope due to river incision. Engineering Geology, 179, 59–75. https://doi.org/10.1016/j.enggeo.2014.06.020
- Hutchinson, J. N. (1988, July 15). General report: Morphological and geotechnical parameters of landslides in relation to geology and hydrogeology. Proceedings of the 5th international symposium on landslides, Lausanne, Vol 1, pp 3–35.
- Jarman, D., & Harrison, S. (2019). Rock slope failure in the British mountains. Geomorphology, 340, 202–233. https://doi.org/10.1016/j.geomorph.2019.03.002
- Jenny, S., Goes, S., Giardini, D., & Kahle, H. G. (2006). Seismic potential of southern Italy. Tectonophysics, 415(1-4), 81–101. https://doi.org/10.1016/j.tecto.2005.12.003
- Martino, S., Antonielli, B., Bozzano, F., Caprari, P., Discenza, M. E., Esposito, C., Fiorucci, M., Iannucci, R., Marmoni, G. M., & Schilirò, L. (2020). Landslides triggered after the 16 August 2018 Mw 5.1 Molise earthquake (Italy) by a combination of intense rainfalls and seismic shaking. Landslides, 17(5), 1177–1190. https://doi.org/10.1007/s10346-020-01359-w
- Martino, S., Cercato, M., Della Seta, M., Esposito, C., Hailemikael, S., Iannucci, R., Martini, G., Paciello, A., Scarascia Mugnozza, G., Seneca, D., & Troiani, F. (2020). Relevance of rock slope deformations in local seismic response and microzonation: Insights from the Accumoli case-study (central Apennines, Italy). Engineering Geology, 266, 105427. https://doi.org/10.1016/j.enggeo.2019.105427
- Martino, S., Della Seta, M., & Esposito, C. (2017). Back-analysis of rock landslides to infer rheological parameters. In X. T. Feng (Ed.), Rock mechanics and engineering, analysis, modeling and design. Taylor and Francis, vol. 3, pp. 237–268.
- Martino, S., Prestininzi, A., & Scarascia Mugnozza, G. (2004). Geological-evolutionary model of a gravity-induced slope deformation in the carbonate Central Apennines (Italy). Quarterly Journal of Engineering Geology and Hydrogeology, 37(1), 31–47. https://doi.org/10.1144/1470-9236/03-030
- Meisina, C., Zucca, F., Notti, D., Colombo, A., Cucchi, A., Savio, G., Giannico, C., & Bianchi, M. (2008). Geological interpretation of PSInSAR data at regional scale. Sensors, 8(11), 7469–7492. https://doi.org/10.3390/s8117469
- Moro, M., Saroli, M., Gori, S., Falcucci, F., Galadini, F., & Messina, P. (2012). The interaction between active normal faulting and large scale gravitational mass movements revealed by paleoseismological techniques: A case study from central Italy. Geomorphology, 151, 164–174. https://doi.org/10.1016/j.geomorph.2012.01.026
- Moro, M., Saroli, M., Salvi, S., Stramondo, S., & Doumaz, F. (2007). The relationship between seismic deformation and deep-seated gravitational movements during the 1997 Umbria-Marche (Central Italy) earthquakes. Geomorphology, 89(3-4), 297–307. https://doi.org/10.1016/j.geomorph.2006.12.013
- Mostardini, F., & Merlini, S. (1986). Appennino centro-meridionale: Sezioni geologiche e proposta di modello strutturale. Memorie della Società Geologica Italiana, 35, 177–202.
- Pánek, T., & Klimeš, J. (2016). Temporal behavior of deep-seated gravitational slope deformations: A review. Earth-Science Reviews, 156, 14–38. https://doi.org/10.1016/j.earscirev.2016.02.007
- Patacca, E., & Scandone, P. (2007). Geology of the southern Apennines. Italian Journal of Geosciences, 7(special issue), 7, 75–119.
- Patacca, E., Scandone, P., Bellatalla, M., Perilli, N., & Santini, U. (1992). La zona di giunzione tra l'arco appenninico settentrionale e l'arco appenninico meridionale nell'Abruzzo e nel Molise. In M. Tozzi, G. P. Cavinato, & M. Parotto (Eds.), Studi preliminari all’acquisizione dati del profile CROP11 Civitavecchia-Vasto. Studi Geologici Camerti, spec. issue 1991/2 (pp. 417–441). Università di Camerino.
- Rovida, A., Locati, M., Camassi, R., Lolli, B., & Gasperini, P. (2016). CPTI15, the 2015 version of the parametric catalogue of Italian earthquakes. Milano, Bologna. http://emidius.mi.ingv.it/CPTI. https://doi.org/10.6092/INGV.IT-CPTI15
- Savelli, D., Troiani, F., Bruciapaglia, E., Calderoni, G., Cavitolo, P., Dignani, A., Ortu, E., Teodori, S., Veneri, F., & Nesci, O. (2013). The landslide-dammed paleolake of Montelago (North Marche Apennines, Italy): Geomorphological evolution and paleoenvironmental outlines. Geografia Fisica e Dinamica Quaternaria, 36(2), 267–287. https://doi.org/10.4461/GFDQ.2013.36.22
- Schwanghart, W., & Scherler, D. (2014). Topotoolbox 2–MATLAB-based software for topographic analysis and modeling in earth surface sciences. Earth Surface Dynamics, 2(1), 1–7. https://doi.org/10.5194/esurf-2-1-2014
- Scrocca, D., & Tozzi, M. (1999). Tettogenesi mio-pliocenica dell’Appennino molisano. Italian Journal of Geosciences, 118(2), 255–286.
- Vezzani, L., Festa, A., & Ghisetti, F. (2010). Geology and tectonic evolution of the Central-Southern Apennines, Italy. Geological Society of America Special Paper, 469, 1–58. https://doi.org/10.1130/SPE469
- Vezzani, L., Ghisetti, F., & Festa, A. (2004). Geological map of Molise (scale 1: 100000). S.E.L.CA., Firenze.