ABSTRACT
Geomorphological field surveys and mapping have been carried out in a catchment of the Dolomites (eastern Italian Alps) as part of a research project aiming at the assessment of sediment availability for mass wasting in mountain environments. The study area is the Liera catchment which was severely affected by a high-intensity, regional-scale storm named ‘Vaia’ (27–30 October 2018), which induced relevant morphological changes, caused a major flood in the main stream and triggered debris flows in many tributaries. This contribution gives a snapshot of the present-day state of the study area to improve the understanding of such a landscape by characterizing the processes that control its geomorphological spatio-temporal dynamics and evolution.
1. Introduction
In recent decades, the role of geomorphological mapping in spatial planning and environmental studies has made a revival with new operating standards (e.g. CitationMagliulo & Valente, 2020; CitationSeijmonsbergen, 2013). GIS-based methods, aerial and satellite images availability, in addition to expert-based observation, are the tools for modern geomorphological mapping (CitationBufalini et al., 2021). Traditionally, geomorphological maps have been used to describe the process and spatial distribution of landforms; today, geomorphological maps are used for land-use and environmental planning activities carried out at various institutional levels, with particular reference to the assessment, management, and mitigation of geomorphological hazards (CitationBufalini et al., 2021 CitationSmith et al., 2011), and also for georesources identification and conservation (e.g. CitationBollati & Cavalli, 2021; CitationCoratza et al., 2021). From this perspective, the impact of severe meteorological events on mountain environments can produce important landscape modifications that are mostly related to landslides, debris flows, and floods. The occurrence of these phenomena is also related to predisposing factors that are linked to geomorphological settings. As a consequence, the assessment of the hazards related to floods and landslides in mountain catchments greatly benefits from a detailed geomorphological map (e.g. CitationVan Westen et al., 2003; CitationSmith et al., 2011), which has to include also general geological information. The study area, the Liera catchment area (Dolomites), has been included in previous works focusing mainly on the geological evolution of the mountainous area of the Dolomites (e.g. CitationBosellini & Rossi, 1974; CitationPanizza et al., 2011 CitationPasuto et al., 2005; CitationSarti & Ardizzoni, 1984) or hydrogeological and karstic features of the Pale di San Martino plateau (e.g. CitationLucianetti et al., 2019 CitationMeneghel & Sauro, 2006). However, there is still a lack of a complete and detailed mapping, which is limited to a 1:100,000 geological map. This work presents a geomorphological map (Main Map) devised to support analyses for the assessment of the effects of the geomorphic changes induced by an extreme meteorological event in a mountain catchment. The map (scale 1:15,000) is the result of detailed field surveys and remote sensing analysis of the Liera catchment, which was strongly affected by an extreme meteorological event, named ‘Vaia Storm’, from 27 to 30 October 2018 (CitationDavolio et al., 2020). Preliminary results of the assessment of the effect of Vaia storm in the catchment are reported in the work by CitationMacchi et al. (2022). Here we focus on presenting methods and the main features characterizing the geomorphological map used as a basic information for the analysis.
2. Study area
The Liera basin is located in the southern sector of the western Dolomites (eastern Italian Alps). The mapped catchment, considered just upstream of the Canale d’Agordo village, drains an area of 34.6 km2 and its elevation ranges between 1027 and 3192 m a.s.l. with an average slope of 36° (). The climate is typically alpine, characterized by cold winters and mild summers. Mean annual precipitation is about 1250 mm; between November and April, precipitation occurs mainly as snow.
Figure 1. Location map and elevation pattern of the Liera catchment.
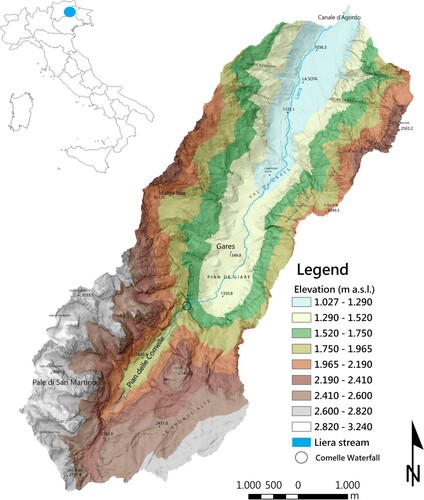
From the geological point of view, the study area is characterized by Triassic sedimentary sequences (see chapter 4.1); it is separated from the Belluno Prealps by the ‘Valsugana Line’ (CitationSelli, 1998). Several tectonic phases affected the area, generating a complex pattern of folds, faults, thrust and fractures. The last one, occurred during the Tertiary Periods and is the W- to S-trending Alpine compression (e.g. CitationDoglioni, 1987). The structural and tectonic setting as well as the glacial advances that occurred during the last glacial cycle of the Late Pleistocene (CitationMonegato & Ravazzi, 2018; CitationSeguinot et al., 2018) have intensively modified the topography and the morphology of the valley. From the morphological point of view, it is possible to split the valley into two main zones separated by a structural edge, which is reflected in a sub-vertical wall (∼400 m) where the Comelle waterfall takes place (A). The southern part of the catchment, set in a low-slope hanging valley, is characterized by massive dolostones, which generate a karstic area in the Pale di San Martino plateau (CitationMeneghel & Sauro, 2006), dikes of volcanic rocks (andesite) also outcrop in the SW hillslope; downstream of the morphological step, the topography is closely related to the molding of late Pleistocene glacial phases, which gave to the Gares valley its typical glacial U-shape profile. The flanks of the valley are interested by a complex tectonic deformation and volcanic and sedimentary rocks outcrop, while the valley bottom is dominated by the fluvial activity and covered by widespread quaternary deposits (fluvial, alluvial, glacial, and talus) (see chapter 4.2).
Figure 2. A) Overview of the Gares Valley where is clearly visible the morphological step at the Comelle waterfall; B) View of the Malga Stia glacial cirque (pink line), all around Ladinian lavas are present. Near Malga Stia glacial deposits are present and the slope between the Gares village and Malga Stia is affected by creep deformation. The alluvial fan, where the Village of Gares developed, is clearly visible.
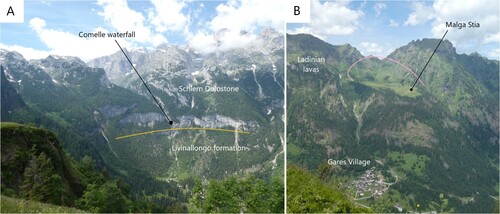
As mentioned before, the Liera catchment was severely affected by the Vaia storm in October 2018. In the Liera catchment, the Vaia storm caused a major flood in the main channel, which underwent substantial widening, triggered debris flows in many tributaries draining small steep catchments, and produced widespread windthrows (). A twofold relationship exists between the Vaia storm and the geomorphological map presented in this paper. On the one hand, the post-event analysis of the impact of the Vaia storm urges a background map that shows the control of geolithological settings and long-term geomorphological evolution on current geomorphic processes. On the other hand, the Vaia Storm, acting as a ‘system forcing event’, made the fragility of the territory much more evident thus helping in geomorphological mapping by showing fresh landforms that express the catchment response to such type of extreme events.
Figure 3. Examples of the effects of the Vaia extreme event in the Liera Catchment: A) effect of the flood in the main channel; B) debris-flow deposits on the fan of a tributary channel; C) landslide on the bank of the Liera stream; D) overbank deposits of the Liera stream including large wood.
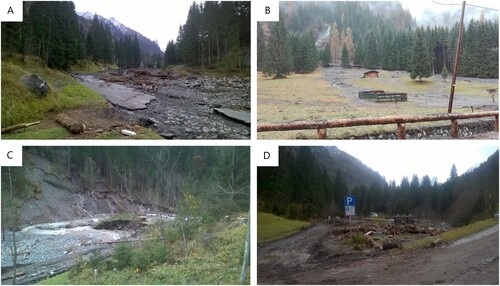
3. Methods
The Liera Catchment area was investigated by using an integrated approach incorporating two steps: (i) the preparation of the geological base map by geological field surveys and using morphometric analysis from LiDAR-derived Digital Terrain Models (DTMs) (i.e. slope and change detection analysis); (ii) the integration of the base map with geomorphological surveys, analysis of the most recent DTM, and multi-temporal orthophotos analysis.
Airborne LiDAR DTMs (spatial resolution of 1 m) were used to provide topographic reference for geomorphological mapping. Two DTMs were used to identify the active landforms by assessing the DEM of Difference (DoD); the most recent DTM was carried out in 2019 (i.e. after the Vaia storm) and the previous one results from data fusion of two LiDAR datasets covering different parts of the catchment: the 2010 LiDAR covers mainly the hillslopes and the 2015 LiDAR covers mainly the valley floor. The area has excellent multi-temporal aerial photo and orthophoto coverage starting from 1954 through 2015 and 2018. In 2019, aerial imagery was acquired during post-Vaia LiDAR flights. The geomorphological map is based on the official cartography of Veneto Region, and the hillshade derived from the 2019 LiDAR DTM.
The map has two different levels of reading themes which are shown in detail:
Geological background: the geological data as well as the succession of the stratigraphic units belong to the Geological Map of Italy at the scale 1:100,000 (sheets 22 ‘Feltre’ and 11 ‘M. Marmolada’). In addition, more detailed maps were also used (CitationSarti & Ardizzoni, 1984). Bibliographic information was integrated with ad hoc field surveys. Only the Anisian formations were grouped because of their complex stratigraphical architecture (CitationAbbà, 2019).
Geomorphological setting: landforms and deposits, mapped in agreement with the guidelines for the Geomorphological Map of Italy (Servizio Geologico Nazionale, Citation1994, CitationCampobasso et al., 2021), have been outlined with continuous or dashed (in case of uncertain or buried limit) lines of different colors according to the genesis and with areal, linear, and punctual symbols of the same color. Two classes of activity are distinguished:
Active landforms, evolving under the action of the main morphogenetic agent for processes in place or reactivatable.
Inactive landforms, no longer evolving under the action of the morphogenetic agent that generated them and no longer reactivatable for the same process in the current geomorphological context and morphoclimatic conditions.
4. The geomorphological map
The geomorphological map (Main Map) of the Liera catchment is produced at scale 1:15,000 in A1 format. The main geomorphological features that characterize the area are mapped, according to their origin, on a synthetic geological map of the area.
4.1. Geological background
The Liera basin can be subdivided into two large areas characterized by different geomorphological and geological settings. The southern region is characterized by Ladinian platform dolostones (Schlern Dolomite) showing clinostratification towards the north, where the basinal sedimentation areas were located. The Schlern Dolomite characterizes the Pale di San Martino Plateau (CitationCastiglioni, 1939; CitationZampieri, 1987), a very large and flat karstic area at an elevation of about 2500–2800 m (CitationLucianetti et al., 2017; CitationMeneghel & Sauro, 2006) characterized by a wide exposure of bare, fractured rocks that favor, along with a large number of caves, a direct recharge path with strong vertical components (CitationLucianetti et al., 2019). This area hosts the main fractured karstified aquifers, which are hydrologically connected with the alluvial and glacial porous aquifers of the valleys (CitationLucianetti et al., 2019).
Andesite volcanic dikes, related to Ladinian volcanism, cross-cut the dolostones in this sector (CitationBosellini et al., 1982). Quaternary deposits of glacial origin are related to post Last Glacial Maximum (LGM) stadial phases (CitationCastiglioni, 1964). Alluvial and debris-flow deposits are present on the Comelle valley floor.
The Comelle waterfall marks the transition between the upper Liera basin and the Gares Valley (A). The valley slopes present a more complex stratigraphic setting because this sector corresponded to a deep-sea basin during the contemporary build-up of the Schlern Dolomite platforms, which were as much as more than 1000 m thick (CitationBosellini & Rossi, 1974). At the foot of the Comelle waterfall escarpment, the Livinallongo Formation (CitationBosellini & Ferri, 1980) consisting of limestones, flinty limestones, green siltstones, and densely stratified sandstones outcrops; it is heterophic to the Schlern Dolomite.
The Livinallongo Formation crops out in the two valley sides in a discontinuous way due to the subsequent fragmentation caused by the tectonic activity during the Triassic and the Alpine phases. Ladinian sedimentary sequence was buried by volcanic rocks (pyroclastites and lavas of intermediate/basic chemistry), volcanoclastic breccias, and hyaloclastites of late Ladinian age (CitationSarti & Ardizzoni, 1984). These are part of the ‘Alpine magmatic cycle’ (CitationPellegrinon et al., 1984). Monzogabbrian intrusions are also present in the volcanic succession along the right side of the Gares Valley. Volcanites widely crop out also in the upper part of the left valley side, where they form the headwall of Malga Stia cirque (B). The Ladinian sequence is then closed by the Marmolada Conglomerate, consisting of breccias and conglomerates outcropping in the hydrographic right in the area of Cima Pape. At the base of the Ladinian units, along the Gares Valley, the Anisian (Masetti & Trombetta, Citation1998) and Lower Triassic (CitationPosenato, 2019) sequences crop out. The Anisian formations consist of three sedimentary cycles. These are characterized by platform dolostones and limestones (Serla Dolomite and Contrin Formation) that are heterophic to transitional and marine-marine units (Morbiac Limestones and Recoaro Limestones). Some continental intervals (Voltago Conglomerate and Richtofen Conglomerate) mark phases of sea-level lowstand. The average thickness of the Anisian sequence is about 300 m (CitationAbbà, 2019). The Triassic succession is closed at the base by the Werfen Formation (Lower Triassic) consisting of siltstones, dolomitic, and locally evaporitic limestones. The Werfen Formation is present near the outlet of the valley on both sides and is about 500 m thick. The rock mass is affected by tectonic distensive structures (normal faults) of Ladinian age, which were subsequently resumed and developed in compression ones (reverse and transcurrent faults) during the Neo-Alpine phase of the Alpine orogeny (Cenozoic).
4.2. Geomorphological setting
From the geomorphological point of view, in the Liera catchment four sectors with different features ( and ) can be distinguished:
The ‘Altopiano delle Pale’, characterized by a dolomitic plateau lacking surface drainage, mostly consisting of outcropping bedrock.
The ridge of the Pale di San Martino, between the ‘Cimon della Pala’ and the ‘Cima di Focobon’, characterized by peaks exceeding 3000 m, with deep valleys of glacial origin that flow eastward into the suspended valley. These valleys hosted small glaciers until the end of the 1980s (CitationBaccolo, 2020). At the base of the walls, there are thick scree mixed with rock fall deposits.
The valley slopes downstream of the Comelle waterfall: they are characterized by steep slopes with a relief of more than 1000 m in elevation, which locally host glacial cirques above 2000 m. These slopes are furrowed by very steep valleys and gullies. Below the cirque of Malga Stia ((B)), the slope shows clear evidence of surface deformations linked to slow bedrock creep and identified in the geomorphological map. In the left sector of the valley, the base of the slope consists of glacial deposits.
The bottom of the Liera valley, characterized by the thalweg of the stream that locally narrows to cross morphological thresholds related to the presence of landslide accumulations or glacial deposits. These landslides most probably dammed the valley in the past, thus leading to its overflowing and subsequent terracing of the alluvial plain. In the valley bottom, the alluvial fans at the mouth of the lateral valleys are also very developed. Short paleochannels of the Liera, with outcropping gravels, are also present.
Figure 4. A) Surficial landslide on the left slope of the Liera stream. In this image it is possible to see the glacial deposits (grey matrix – identified with a white line) covered by the newest slope deposit (red matrix); C shows the landslide before the mitigation measures; B) Alluvial deposit composed of sub-rounded gravel and cobbles derived from Liera creek; C) Rock fall and its deposit located on the upper part of the Cima Pape; D) Debris-flow deposit composed of gravel, pebbles, and cobbles arranged in a chaotic matter.
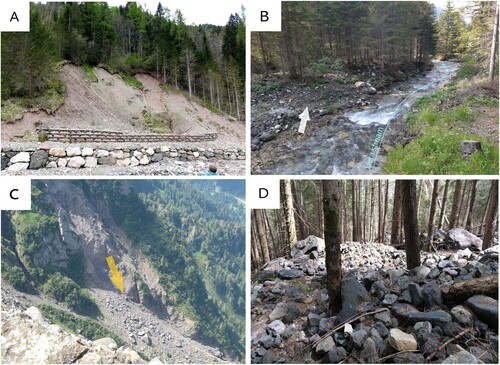
Table 1. Deposits recognized and mapped in the Liera catchment.
5. Conclusions
The geomorphological map of the Liera catchment combines the geological background with the representation of landforms created by ancient and recent (the Vaia storm of October 2018) geomorphic processes. The geomorphological map is not only necessary for characterizing the environment but also for a better landscape management and territorial planning. In addition, the availability of a geomorphological map can also contribute to effectively assessing geomorphological hazards for an improved landscape resilience and increased civil protection purposes. Finally, this research enriches the knowledge of the geomorphology of a key sector of the Western Dolomites.
Software
The map presented was produced in the Geographic Information System ArcGis® 10.3.1 (ESRI) and the final editing of the map and photos was made with Adobe Illustrator® 2019.
Supplemental Material
Download Zip (34.8 MB)Acknowledgements
We wish to thank Prof. Mauro Soldati, Dr Chandra Jayasuriya and Dr Irene Bollati for the useful comments and accurate revisions to our manuscript.
Disclosure statement
No potential conflict of interest was reported by the author(s).
Data availability statement
The 2019 1-m resolution DTM, used as a basemap, is available under request at the Veneto Region. The technical cartography can be downloaded from the Veneto Region website (https://www.regione.veneto.it/web/ambiente-e-territorio/ctr-vettoriale). The other data supporting the findings of this study are available from the corresponding author, upon reasonable request.
Additional information
Funding
References
- Abbà, T. (2019). Conoscere la geologia del veneto. Vol. I, CAI, Fond. Berti, Duck Ed.
- Baccolo, G. (2020). Piccoli ghiacciai alpini. Mappamondi, 2. Cierre Edizioni.
- Bollati, I. M., & Cavalli, M. (2021). Unraveling the relationship between geomorphodiversity and sediment connectivity in a small alpine catchment. Transactions in GIS, 25(5), 2481–2500. https://doi.org/10.1111/tgis.12793
- Bosellini, A., Castellarin, A., Doglioni, C., Guy, F., Lucchini, F., Perri, M. C., Rossi, P. L., Simboli, G., & Sommavilla, E. (1982). Magmatismo e tettonica nel Trias delle Dolomiti. In A. Castellarin & G. B. Vai (a cura di) (Eds.), Guida alla geologia del Sudalpino centro-orientale, (pp. 189–210). Società Geologica Italiana.
- Bosellini, A., & Ferri, R. (1980). La Formazione di Livinallongo (Buchenstein) nella Valle di San Lucano (Ladinico inferiore, Dolomiti Bellunesi). Ann. Univ. Ferrara (N.S.) Sez. Sc. Geol e Paleont., 9(6/5), 63–89.
- Bosellini, A., & Rossi, D. (1974). Triassic carbonate buildups of the Dolomites, northern Italy. In L. F. Laporte (Ed.), Reefs in time and space, 18 (pp. 209–233). Soc. Econ. Paleont. Miner. Spec. Publ.
- Bufalini, M., Materazzi, M., De Amicis, M., & Pambianchi, G. (2021). From traditional to modern ‘full coverage’ geomorphological mapping: a study case in the Chienti river basin (Marche region, central Italy). Journal of Maps, 17(3), 17–28. https://doi.org/10.1080/17445647.2021.1904020
- Campobasso, C., Carton, A., Chelli, A., D’Orefice, M., Dramis, F., Graciotti, G., Guida, D., Pambianchi, G., Peduto, F., & Pellegrini, L. (2021). Aggiornamento ed integrazioni delle linee guida della Carta Geomorfologica d’Italia alla scala 1:50.000. Quaderni del Servizio Geologico d’Italia – ISPRA, Ser. III, 13(1), 93.
- Castiglioni, B. (1939). Il Gruppo delle Pale di San Martino e valli limitrofe. Memorie dell'Istituto Geologico della R. Università di Padova, 13, 1–101.
- Castiglioni, G. B. (1964). Sul morenico stadiale nelle Dolomiti. Memorie dell'Istituto Geologico della R. Università di Padova, 24, 1–16.
- Coratza, P., Bollati, I. M., Panizza, V., Brandolini, P., Castaldini, D., Cucchi, F., Deiana, G., Del Monte, M., Faccini, F., Finocchiaro, F., Gioia, D., Melis, R., Minopoli, C., Nesci, O., Paliaga, G., Pennetta, M., Perotti, L., Pica, A., Tognetto, F., … Pelfini, M. (2021). Advances in geoheritage mapping: Application to iconic geomorphological examples from the Italian landscape. Sustainability, 13(20), 11538. https://doi.org/10.3390/su132011538
- Davolio, S., Della Fera, S., Laviola, S., Miglietta, M. M., & Levizzani, V. (2020). Heavy precipitation over Italy from the mediterranean storm “Vaia” in October 2018: Assessing the role of an atmospheric river. Monthly Weather Review, 148(9), 3571–3588. https://doi.org/10.1175/MWR-D-20-0021.1
- Doglioni, C. (1987). Tectonics of the Dolomites (Southern Alps, Northern Italy). Journal of Structural Geology, 9(2), 181–193. https://doi.org/10.1016/0191-8141(87)90024-1
- Lucianetti, G., Cianfarra, P., & Mazza, R. (2017). Lineament domain analysis to infer groundwater flow paths: Clues from the Pale di San Martino fractured aquifer, Eastern Italian Alps. Geosphere, 13(5), 1729–1746. https://doi.org/10.1130/GES01500.1
- Lucianetti, G., Mazza, R., & Mastrorillo, L. (2019). Hydrogeology of a high Alpine carbonate aquifer (Pale di San Martino, Dolomites, Northern Italy). Journal of Maps, 15(2), 448–459. https://doi.org/10.1080/17445647.2019.1611497
- Macchi, G., Crema, S., Arziliero, L., Boretto, G., De Fanti, B., Marchi, L., Monegato, G., & Cavalli, M. ( 2022). Assessing debris-flow activity and geomorphic changes caused by an extreme rainstorm: the case study of the Liera catchment (Dolomites, northeastern Italy). Rendiconti Online Della Società Geologica Italiana, 58, 2–8. https://doi.org/10.3301/ROL.2022.13
- Magliulo, P., & Valente, A. (2020). GIS-based geomorphological map of the Calore river floodplain near Benevento (Southern Italy) overflooded by the 15th October 2015 event. Water, 12(1), 148. https://doi.org/10.3390/w12010148
- Masetti, D., & Trombetta, G. L. (1998). L'eredità anisica nella nascita delle Piattaformemedio-triassiche delle Dolomiti occidentali. Memorie di Scienzze Geologiche, 50, 213–237.
- Meneghel, M., & Sauro, U. (2006). Dolines of karstic and periglacial origin in the high mountain karst of Pale di San Martino plateau (Dolomites). Z. Geomorpf. N.F., 50(1), 63–76. https://doi.org/10.1127/zfg/50/2006/63
- Monegato, G., & Ravazzi, C. (2018). The late Pleistocene multifold glaciation in the Alps: Updates and open questions. Alpine and Mediterranean Quaternary, 31, 225–229. https://doi.org/10.26382/AIQUA.2018.AIQUAconference
- Panizza, M., Corsini, A., Ghinoi, A., Marchetti, M., Pasuto, A., & Soldati, M. (2011). Explanatory notes of the Geomorphological map of the Alta Badia valley (Dolomites, Italy). Geografia Fisica e Dinamica Quaternaria, 34(1), 105–126.
- Pasuto, A., Siorpaes, C., & Soldati, M. (2005). Carta geomorfologica dell’area circostante Cortina d’Ampezzo (Dolomiti, Italia). SELCA.
- Pellegrinon, B., Fenti, V., Andrich, O., & Pellegrini, G. B. (1984). Garès, Rivista C.A.I. Sezione Agordina, 10.
- Posenato, R.. (2019). The end-Permian mass extinction (EPME) and the Early Triassic biotic recovery in the western Dolomites (Italy): State of the art. Bollettino della Società Paleontologica Italiana, 58(1), 11–34. Doi: 10.4435/BSPI.2019.05
- Sarti, M., & Ardizzoni, F. (1984). Tettonica Triassica nel gruppo di Cima Pape – Pale di Sanson. Mem. Sc. Geol., 36, 353–370.
- Seguinot, J., Ivy-Ochs, S., Jouvet, G., Huss, M., Funk, M., & Preusser, F. (2018). Modelling last glacial cycle ice dynamics in the Alps. The Cryosphere, 12(10), 3265–3285. https://doi.org/10.5194/tc-12-3265-2018
- Seijmonsbergen, A. C. (2013). The modern geomorphological map. In J. Shroder, A. D. Switzer, & D. M. Kennedy (Eds.), In methods in geomorphology (pp. 35–52). Academic Press.
- Selli, L. (1998). Il lineamento della Valsugana fra Trento e Cima d’Asta: cinematica neogenica ed eredità strutturali permo-mesozoiche nel quadro evolutivo del Sudalpino orientale (NE-Italia). Memorie della Società Geologica Italiana, 53, 503–541.
- Servizio Geologico Nazionale. (1994). Carta Geomorfologica d'Italia - 1:50.000. Guida al rilevamento. Quaderni, Serie III (Vol. 4, pp. 1–42). Istituto Poligrafico e Zecca dello Stato.
- Smith, M. J., Paron, P., & Griffiths, J. S. (Eds.). (2011) Geomorphological mapping: Methods and applications (Developments in Earth Surface Processes Vol. 15, pp. 1–612). Elsevier Science.
- van Westen, C. J., Rengers, N., & Soeters, R. (2003). Use of Geomorphological Information in Indirect Landslide Susceptibility Assessment. Natural Hazards, 30(3), 399–419. https://doi.org/10.1023/B:NHAZ.0000007097.42735.9e
- Zampieri, D. (1987). Le piattaforme carbonatiche triassiche delle Pale di San Martino (Dolomiti). Memorie di Scienze Geologiche, 39, 73–83.