ABSTRACT
The Magic project (MArine Geohazard along Italian Coasts), a large coordinated initiative that involved the whole marine geological research community in Italy in 2007–2013, produced a series of maps of the Geohazard features of the Central-Southern Tyrrhenian Seamounts. The features derive from multibeam surveys and therefore mainly rely on the morphological expression of seafloor and shallow sub-surface processes and events. Potential geohazards related to eruption and flank instability of the volcanic seamounts of the Tyrrhenian Sea are discussed and compared.
KEYWORDS:
1. Indroduction
This article illustrates the Maps of Geohazard features of the Tyrrhenian Central-Southern volcanic seamounts obtained in the course of the Magic project (Marine Geohazard along Italian Coasts), a large, coordinated initiative that involved the entire marine geological research community in Italy in 2007–2013. The MaGIC Project focused on the acquisition of morphobatimetric data in outer shelf, slope and seamounts of the Italian continental margins, in order to depict and classify the geological hazard features. The features were derived from multibeam surveys and therefore mainly rely on the morphological expression of seafloor and shallow sub-surface processes and events. In particular, the morphological and structural features of the volcanoes are described with emphasis on the potential hazards posed by eruption and flank instability. Two levels of interpretation are presented: the Main Map of the Physiographic Domain at scale 1:250.000 and the map of the Morphological Units and Morpho-bathymetric Elements (areas and vectors respectively) at 1:100.000 scale.
2. Study area: the Central-Southern Tyrrhenian seamounts
The Tyrrhenian Sea is the youngest back-arc basin in the Mediterranean (). Its formation is related to the extension and crustal thinning which followed the subduction of the Ionian oceanic lithosphere beneath the Calabrian Arc (Kastens et al., Citation1988; Sartori, Citation1990) in a context of convergence between the African plate and Eurasia (Malinverno & Ryan, Citation1986; Ward, Citation1994. The initial E-W opening stage of the Tyrrhenian Basin, is dated Tortonian (Kastens et al., Citation1988; Zitellini et al., Citation1986). During the Pliocene, production of oceanic crust is located in the Vavilov back-arc basin, where the homonymous Vavilov Volcano developed. Subsequently, the minimum stress direction changed to ESE and production of oceanic crust migrated eastwards forming the Marsili back-arc basin (1.9-1.7 Ma) and the Marsili Volcano (<0.73 Ma), originating in the present day arc and back-arc configuration of the southern Tyrrhenian (). The magmatic products of the Tyrrhenian Sea are characterised by contemporary eruption of IAB (Island Arc Basalt) and OIB (Ocean Island Basalts) magmas. Calc-alkaline lavas are present in the Marsili Basin and in the Vavilov Basin, the Marsili Volcano, the Palinuro volcano, the Aeolian Islands and in their submarines extensions. In addition, MORB basalts are found in the Vavilov Basin. Instead, OIB magmas, of asthenospheric origin, are present only in some restricted areas, such as the Marsili Seamount, the Vavilov Volcano, Ustica Island and the Prometeo lava field located South-East of Ustica (Marani & Trua, Citation2002; Trua et al., Citation2002). In this context, the Palinuro volcanic complex developed at ∼350 ka BP (Robin et al., Citation1987). It consists of a chain of fifteen volcanic edifices that extend in an EW direction for more than 90 km (Passaro et al., Citation2010). The IAB composition of Palinuro lavas and its location in the upper margin of the Marsili back-arc basin, indicate that the Palinuro volcanic complex represents the northern limit of the Aeolian arc magmatism, at the margin between the continental and oceanic crusts (), where the direct influence of the subduction process becomes no longer effective (Cocchi et al., Citation2017). The large volcanic seamounts of the central Tyrrhenian Sea are the products that witness the creation and evolution of the back-arc basin. This paper discusses the morphology of the Marsili, Vavilov and Palinuro volcanoes in terms of landforms and tectono/magmatic features including the occurrence of hydrothermal activity and assicoated deposits. Vavilov and Marsili volcanoes are centrally located in their homonymous back-arc basins while the PalinuroVocanic Complex (PVC) developed on the eastern margin of the basin along a lithospheric fault zone delimiting the oceanic and continental crusts. Cumulatively, the three volcanoes have a volume of over 4000 km3, developed in a setting in which the lithosphere in subduction was rapidly sinking and characteriz ed by fast rollback (Corradino et al., Citation2022; De Astis et al., Citation2003; Gvirtzman & Nur, Citation1999; Manu-Marfo et al., Citation2019; Marani & Trua, Citation2002; Trua et al., Citation2018; Ventura et al., Citation2013). The active geodynamic setting, the continuing volcano/tectonic activity of Marsili together with the position of the PVC characterised by hydrothermalism and shallow-water zones can be considered multiple potential sources for the generation of hazards, both directly in terms of submarine eruptions and indirectly by the destabilisation and failure of the volcanic edifices and related tsunamigenic landslides. Repeated mapping and systematic monitoring should be undertaken to better understand the natural risks with regard to the population and the infrastructure of the surrounding coastal regions.
Figure 1. Sketch map of the geodynamic setting of the Tyrrhenian Sea within the central Mediterranean. VB, Vavilov Basin; MB Marsili Basin.
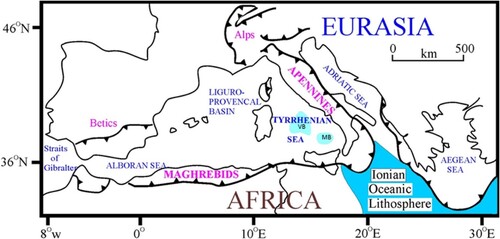
Figure 2. Multibeam, depth colour-coded, shaded relief bathymetric map of the Tyrrhenian Sea back-arc basin. The white boxes enclose the three large seamounts of the basin which are detailed in .
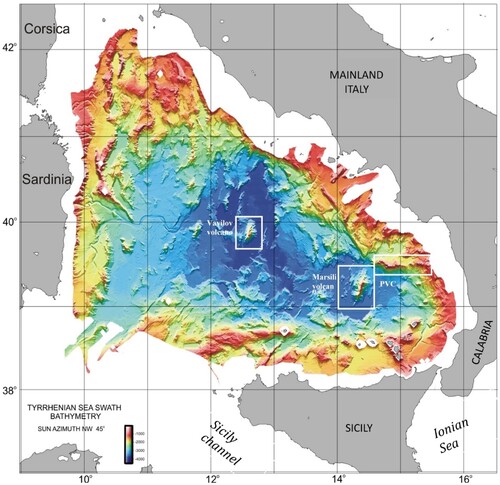
3. Methods and software
Since the maps were produced using the same interpretative and cartographic standards, the procedure is described in detail in Ridente and Chiocci (this volume). The legend of the Physiographic Domain map is present on the map while the legend of the Morphological Units and Morpho-bathymetric Elements map is present as a separate table.
4. Maps of morphological units and Morpho-bathymetric elements
4.1. Marsili area (MaGIC sheet 21)
The Sheet 21 ‘Marsili’ includes the Marsili Volcano, a NNE-SSW-elongated seamount constructed in the last 0.7 Myr, with a length of 60 km and a mean width of 20 km ((a)). The volcano rises 3,500 m from the basement level of the Marsili basin to a minimum depth of 500 m and has a volume of over 2400 km3. Marsili is presently active, characterised by high frequency and continuous tremor and volcano-tectonic swarms due to both geothermal and volcanic activity (D’Alessandro et al., Citation2009, Citation2012). Direct dating of explosive volcanism at 3kyr BP has been obtained for a tephra deposit close to Marsili summit (Iezzi et al., Citation2014). The summit axis is a narrow, 1-km-wide, linear rift zone of lower gradient stretching 25 km along the main axis of the volcano, approximately bounded by the 1000 m isobaths. The rift zone is characterised by the development of linear structures clustered in segments generated mainly by the alignment of narrow, linear cone ridges, or by the linear arrangement of several circular-based cones (Marani & Trua, Citation2002; Nicotra et al., Citation2024; Ventura et al., Citation2013). Aligned volcanic landforms continue in the tip regions of the volcano, down to the basin floor. The linear rift zone along the central portion of the volcano is the principal site of stress release, marking the preferential trend of dyke emplacement along the volcano. Numerous small seamounts grow on the flanks of Marsili Volcano, being mostly developed on its north-western and western lower flank regions. Very low gradient, flat tops characterise several seamounts. A series of volcanic terraces, with typical semi-circular, steeply sloping flanks bounding flat seafloor, develop at 2800 m depth (Marani & Gamberi, Citation2004). Two NNE-SSW-directed (N16°) fault sets, parallel to the general trend of the summit axis, develop symmetrically in the basin-floor region, bounding the south-eastern and north-western flanks of the volcano, forming horst and graben pairs at the sides of Marsili Volcano. Hydrothermal activity is present at the summit cone and along the rift zone ((a) and (c)) showing strong alteration, modelled by magnetics and gravimetriy, reaching 2 km depth at the central summit zone (Caratori Tontini et al., Citation2009, Citation2014; Ligi et al., Citation2014). The origin of the Marsili volcano is still debated. A number of authors interpret the volcano as representing the spreading centre of the southern Tyrrhenian back arc Marsili basin (e.g. CitationAlbert at al., 2022; Cocchi et al., Citation2017; Faccenna et al., Citation2005; CitationGennaro et al., 2023; Marani & Trua, Citation2002; Trua & Marani, Citation2021; Trua et al., Citation2018) or conversely as an island arc volcano (Ventura et al., Citation2013) or a relict fore-arc volcano (Corradino et al., Citation2022). Augmented magmatic production is linked to the formation of lateral tears in the subducting Ionian slab and the subsequent input of deep and hot asthenospheric material into the mantle wedge (Cocchi et al., Citation2009; Marani & Trua, Citation2002). The thickness, dimensions and hydrothermal alteration of Marsili volcano, combined with the seismic volcanic tremor events, make it a highly potential site of large-scale landslides capable of severely impacting the surrounding Italian coast (Gallotti et al., Citation2021). Furthermore, a hazard may be foreseen in the case of a submarine eruptions of the Marsili summit cone, albeit occuring at a minimum depth of 500 metres.
Figure 3. The three largest volcanic seamounts of the Tyrrhenian Sea. (a) Marsili Seamount is the largest volcano in the region. Constructed in the last 0.7 My the Marsili volcano is centred on the <2 My old Marsili oceanic back-arc basin. The volcano has symmetrical flanks and a well-developed linear axial rift zone (see text for description). There is no evidence of recent large landslides on the edifice. This is not true for (b) Vavilov volcano centred in the Pliocene Vavilov back-arc basin. This older seamount has a striking assymentry due to a sector collapse that truncates its entire western flank (see text for description). (c) The Palinuro Volcanic Complex lies for about 65 km along the eastern margin of the Tyrrhenian Basin. It is dated to 0.35 My. It is in made up of a linear series of volcanic edifices and constructed on a 1.5 km escarpment dropping to the south to the Marsili oceanic crust. This lithospheric structure, a STEP fault, juxtaposes the ‘alpine’ Calabrian nappes to the subduction dominated geodynamic province of the SE Tyrrhenain. Several of the edifices of the complex show different degrees of deformation: from gravity collapse calderas to structural failure.
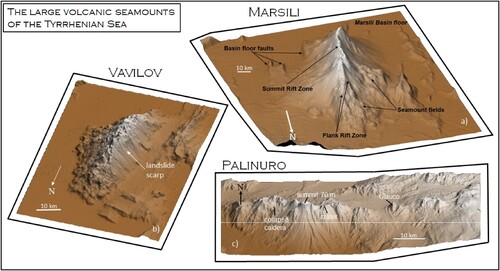
Figure 4. Examples of seafloor Volcanic landforms observed by ROV on the Marsili and Paliburo seamounts. (a) Low-temperature hydrothermal vents at the summit cone of Marsili; at right an active vent with deposits of Fe oxy/hydroxides and bacterial matte, at left an inactive vent. Field of View (FOV) 1.5 m. (b) Fe oxy/hydroxide hydrothermal mound with several active vents at the northern boundary of the caldera ring-fault cone of Palinuro. (FOV 3 m). (c) Lava ribbons descend the summit cone flanks towards the central plateau of Marsili Volcano (FOV 2 m). (d) Well developed pillow lava mound on the flanks of a volcano in the central region of the PVC (FOV 4 m). For (b) and (d) rights obtained from NOAA Office of Ocean Exploration and Research, The Ocean Exploration Trust and the Institute for Exploration, University of Rhode Island).
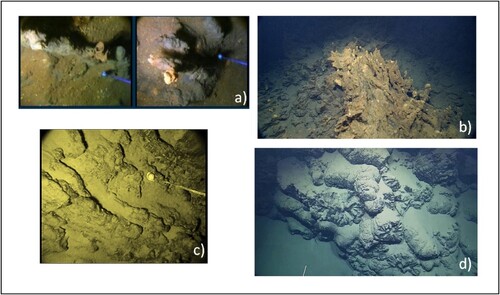
4.2. Vavilov area (MaGIC sheet 22)
Vavilov Volcano has a length of ∼30 km, elongated in the N-S direction, with a maximum width of ∼14 km. It rises 2800 m from the flat Vavilov Basin floor, at 3600 m depth, to a minimum water depth of 800 m ((b)) It is a back-arc volcano developed in the Vavilov Basin during Pliocene spreading of the basin at 4.3-2.6 Ma. Vavilov formed at the time of oceanisation of the Vavilov back-arc basin, at approximately 3 Ma (Kastens et al., Citation1988). However, from 0.4 to 0.1 Ma, the summit area seems to have been subsequently active (Robin et al., Citation1987).
The overall morphology of Vavilov Volcano is dominated by the strong asymmetry between its eastern and western flanks. While the eastern flank, dipping on average 15°, displays irregular ‘volcanic’ topography due to small cones, restricted terraces and ridges, a large portion of the western flank is steeply dipping (from >30° above 2800 m depth to 20° along the lower flanks) and is remarkably smooth, displaying a complete lack of small scale topography(Gamberi et al., Citation2006; Savelli & Ligi, Citation2017). Considering the arcuate scar that bounds the high gradient western flank, it is likely that this portion of the volcano has been affected either by one or more flank collapses or by faulting, resulting in the removal of a large volume of the pre-existing edifice. The lack of rough topography on the adjacent basin floor could be related to sediment blanketing of the landslide debris, thus, indirectly indicating a timing of the event. Submersible observations and magnetic anomaly data allow to divide the volcano edifice into three structural and age intervals (Savelli & Ligi, Citation2017). The summit of the volcano is composed of a relatively low gradient area occupied by two large 250 m high, circular cones and a number of smaller edifices. Both the southern and northern flanks of the volcano are traversed by 100–150-m-high ridges, some characterised by the development of small cones, that originate at the summit tips and continue to the base of the volcano. In particular on the northern flank, interruption of the ridges by steep transverse scarps gives rise to terrace-like morphologies. However, apart from the summit cones, Vavilov Volcano is the site of a number of circular based cones that are mainly located on the lower slope portions, between 2500 and 3500 m depth. Numerical modelling prediction of tsunami generation due to landslides (Gallotti et al., Citation2023) on Vavilov show that significant hazards relate to very large scale events, similar to the sector collapse of the western flank of the volcano.
4.3. Seamount-Palinuro area (MaGIC sheet 29)
The Sheet 29 ‘Seamount Palinuro’ includes the Palinuro Volcanic Complex (PVC), which is made up of island arc basalts and basaltic andesites, similar to the magmatism characterising the Aeolian Arc volcanoes and seamounts, and has been dated 0.35 Ma (Beccaluva et al., Citation1985). The PVC delimits the north-western extent of the Aeolian Arc volcanism ( and (c)). It is a composite volcanic complex stretched in the E-W direction for 90 km. It stands between the northern lower slope at 2000 m depth and the southern deep Marsili Basin (3400 m depth). 15 major volcanic edifices can be recognised along the PVC, their bases at times coalescing to form a near continuous volcanic ridge (Cocchi et al., Citation2017. The western region of the PVC is characterised by two caldera structures. The eastern-most caldera is breached southwards but is the site of small volcanic cones developed on ring faults at its northern and western edges. Polymetallic mineralised hydrothermal deposits ((b)) have been sampled from one of these small cones both by dredging and drilling (Marani et al., Citation1999; Petersen et al., Citation2014). Shallow water depths characterise the central portion of the PVC, with two volcanoes, Piotr's cones (Passaro et al., Citation2010) reaching 175 and 70 m depth. They display flat tops, mostly due to emersion during glacial times. A series of smaller cones develop in the to the east, ((c)) including a small cone characterised by an asymmetric crater located at a minimum depth of 500 m. This portion of the PVC is affected by tectonic structures and gravitational collapses that are very prominent in the morphology of the central PVC edifices. The far eastern region is the site of a number of relatively shallow-water seamounts of volcanic origin (De Ritis et al., Citation2019). The seamounts were emplaced during the Brunhes Cron and and are considered presently not active. Structurally, the PVC is positioned along a region of suture between the Apenninic Chain and the nappes of the Calbro-Peloritan Arc. The North flank of the PVC rests on a flat basin plain at 2000 m depth, while the southern flank of the PVC drops down to the ocean crust floored Marsili Basin, lying at 3400 m depth within a stretch of a few km. This near 1.5 km drop represents a tectonic structure of lithospheric importance interpreted as a STEP fault (Cocchi et al., Citation2017) representing the northern tear of the Ionian slab. A stability analysis of the steep southern slope of Palinuro reveals the potential of landslide generation and resulting tsumani (Gallotti et al., Citation2020) which would critically impact the peri-Tyrrhenian coast.
5. Conclusions
The Tyrrhenian Sea is a young back-arc basin developed above the subduction zone in the central Mediterranean in two stages: during Pliocene in the central Tyrrhenian, followed by spreading during Pleistocene in the southern Tyrrhenian.
Ocean crust-floored areas developed large volcanoes along their central axes: the Vavilov and Marsili volcanoes.
Magmatism is dominated by IAB lavas; MORB-type magmas floor the Vavilov basin crust and OIB magmatism occurs in restricted regions. IAB magmatism ends northwards at a tectonic structure along which a 70 km chain of coalescing volcanic edifices develop: the Palinuro Volcanic Complex. Increased magma production establishing the central volcanoes results from the lateral tearing of the subducting lithosphere provoking rapid slab roll-back and the rise of hot asthenosphere into the overlying mantle wedge. The OIB volcanism that develops laterally to the basins, is the surface trace of the slab tear edges.
The largest volcano, Marsili, is active and also structurally weak owing to pervasive hydrothermal alteration to significant depth. Its volume and topography are prone to be destabilised by a number of trigger events that may cause potential hazards impacting the surrounding coastal areas.
The STEP fault on which Palinuro Volcanic Complex develops is itself a zone of lithospheric-scale rupture, sourcing the hydrothermalism of parts of the PVC. Considering the steep, 1.5 km southern slope, a relatively mild tectono/volcanic event could have the capacity to generate large-scale submarine land-slides able to impact the surrounding Italian shoreline.
Emphasis should also be directed to hazards that are directly connected to submarine volcanic eruptions in terms of water depths, particularly in the cases of the Palinuro shallow-water vents in its central and eastern portions.
Software
Global mapper© by Blue Marble Geographics and the IHS Kingdom Suite were used for bathymetric and seismic data visualisation and interpretation.
7_gf_of_the_Central_Southern_tyrrhenian_Seamounts_Marani .pdf
Download PDF (11 MB)Acknowledgements
We thank officers and crews of R/V Urania and Maria Grazia (CNR), R/V Universitatis (Conisma) and R/V Explora (OGS), which acquired the data.
Data availability statement
Bathymetric data and interpretation data (vector format) are available at the web site https://github.com/pcm-dpc/MaGIC/tree/master/MaGIC-1
Disclosure statement
No potential conflict of interest was reported by the author(s).
Additional information
Funding
References
- Albert, H., Trua, T., Fonseca, J., Marani, M. P., Gamberi, F., Spiess, R., & Marzoli, A. (2022). Time scales of open-system processes in a complex and heterogeneous mush-dominated plumbing system. Geology, 50(8), 869–873. https://doi.org/10.1130/G49934.1
- Beccaluva, L., Gabbianelli, G., Lucchini, F., Rossi, P. L., & Savelli, C. (1985). Petrology and K/Ar ages of volcanics dredged from the Eolian seamounts: implications for geodynamic evolution of the southern Tyrrhenian basin. Earth and Planetary Science Letters, 74(2–3), 187–208. doi:10.1016/0012-821X(85)90021-4
- Caratori Tontini, F., Bortoluzzi, G., Carmisciano, C., Cocchi, L., de Ronde, C. E. J., Ligi, M., & Muccini, F. (2014). Near-bottom magnetic signatures of submarine hydrothermal systems at Marsili and Palinuro volcanoes, southern Tyrrhenian sea, Italy. Economic Geology, 109(8), 2119–2128. https://doi.org/10.2113/econgeo.109.8.2119
- Caratori Tontini, F., Marani, M. P., Muccini, F., Bortoluzzi, G., & Carmisciano, C. (2009, October). Chronology of the transition from a spreading ridge to an accretional seamount in the Marsili Backarc Basin (Tyrrhenian sea). Terra Nova, 21(5), 369–374. doi:10.1111/j.1365-3121.2009.00891.x
- Cocchi, L., Caratori Tontini, F., Muccini, F., Marani, M. P., Bortoluzzi, G., & Carmisciano, C. (2009). Chronology of the transition from a spreading ridge to an accretional seamount in the Marsili backarc basin (Tyrrhenian sea). Terra Nova, 21(5), 369–374. https://doi.org/10.1111/j.1365-3121.2009.00891.x
- Cocchi, L., Passaro, S., Tontini, F. C., & Ventura, G. (2017). Volcanism in slab tear faults is larger than in island-arcs and back-arcs. Nature Communications, 8(1), 1451. https://doi.org/10.1038/s41467-017-01626-w
- Corradino, M., Balazs, A., & Faccenna, C. (2022). Arc and forearc rifting in the Tyrrhenian subduction system. Scientific Reports, 12(1), 4728. https://doi.org/10.1038/s41598-022-08562-w
- D’Alessandro, A., D’Anna, G., Luzio, D., & Mangano, G. (2009). The INGV's new OBS/H: Analysis of the signals recorded at the Marsili submarine volcano. Journal of Volcanology and Geothermal Research, 183(1–2), 17–29. https://doi.org/10.1016/j.jvolgeores.2009.02.008
- D’Alessandro, A., D’Anna, G., & Mangano, G. (2012). Evidence of persistent seismo-volcanic activity at Marsili seamount. Annals of Geophysics, 55(2), https://doi.org/10.4401/ag-5515
- De Astis, G., Ventura, G., & Villardo, G. (2003). Geodynamic significance of the Aeolian Volcanism (Southern Tyrrhenian Sea, Italy) in light of structural, seismological and geochemical data. Tectonics, 22(4), 1040. doi:10.1029/2003TC001506
- De Ritis, R., Pepe, F., Orecchio, B., Casalbore, D., Bosman, A., Chiappini, M., Chiocci, F., Corradino, M., Nicolich, R., Martorelli, E., Monaco, C., Presti, D., & Totaro, C. (2019). Magmatism along lateral slab edges: Insights from the Diamante-Enotrio-Ovidio volcanic-intrusive complex (Southern Tyrrhenian Sea). Tectonics, 38(8), 2581–2605. https://doi.org/10.1029/2019TC005533
- Faccenna, C., Civetta, L., D'Antonio, M., Funiciello, F., Margheriti, L., & Piromallo, C. (2005). Constraints on mantle circulation around the deforming Calabrian slab. Geophysical Research Letters, 32, L06311. https://doi.org/10.1029/2004GL021874
- Gallotti, G., Passaro, S., Armigliato, A., Zaniboni, F., Pagnoni, G., Wang, L., Sacchi, M., Tinti, S., Ligi, M., & Ventura, G. (2020). Potential mass movements on the Palinuro volcanic chain (Southern Tyrrhenian Sea, Italy) and consequent tsunami generation. Journal of Volcanology and Geothermal Research, 404, 107025. https://doi.org/10.1016/j.jvolgeores.2020.107025
- Gallotti, G., Zaniboni, F., Arcangeli, D., Angeli, C., Armigliato, A., Cocchi, L., Muccini, F., Zanetti, M., Tinti, S., & Ventura, G. (2023). The tsunamigenic potential of landslide-generated tsunamis on the Vavilov seamount. Journal of Volcanology and Geothermal Research, 434, 107745. https://doi.org/10.1016/j.jvolgeores.2023.107745
- Gallotti, G., Zaniboni, F., Pagnoni, G., Romagnoli, C., Gamberi, F., Marani, M., & Tinti, S. (2021). Tsunamis from prospected mass failure on the Marsili submarine volcano flanks and hints for tsunami hazard evaluation. Bulletin of Volcanology, 83. https://doi.org/10.1007/s00445-020-01425-0
- Gamberi, F., Marani, M., Landuzzi, V., Magagnoli, A., Penitent, D., Rosi, M., Bertagnini, A., & Di Roberto, A. (2006). Sedimentologic and volcanologic investigation of the deep Tyrrhenian sea; preliminary results of cruise Vst02. Annals of Geophysics, 49, 767–781.
- Gennaro, E., Iezzi, G., Cocchi, L., & Ventura, G. (2023). Large silicic magma chambers at the Moho depth characterize the multi-level plumbing system of back-arc spreading ridges. Lithos, 456–457, 107325. https://doi.org/10.1016/j.lithos.2023.107325
- Gvirtzman, Z., & Nur, A. (1999). The formation of Mount Etna as the consequence of slab rollback. Nature, 401(6755), 782–785. doi:10.1038/44555
- Iezzi, C. C., Ventura, G., Vallefuoco, M., Cavallo, A., Behrens, H., Mollo, S., Paltrinieri, D., Signanini, P., & Vetere, F. (2014). First documented deep submarine explosive eruptions at the Marsili Seamount (Tyrrhenian Sea, Italy): A case of historical volcanism in the Mediterranean Sea. Gondwana Research, 25(2), 764–774. https://doi.org/10.1016/j.gr.2013.11.001
- Kastens, K. A., Mascle, J., Auroux, C., Bonatti, E., broglia, A., Channell, J., & Torii, M. (1988). ODP Leg 107 in the Tyrrhenian sea: Insight into passive margin and back-arc basin evolution. Geological Society of America Bulletin, 100(7), 1140–1156. https://doi.org/10.1130/0016-7606(1988)100<1140:OLITTS>2.3.CO;2
- Ligi, M., Cocchi, L., Bortoluzzi, G., D’Oriano, F., Muccini, F., Tontini, F. C., de Ronde, C. E. J., & Carmisciano, C. (2014). Mapping of seafloor hydrothermally altered rocks using geophysical methods: Marsili and Palinuro Seamounts, Southern Tyrrhenian Sea. Economic Geology, 109(8), 2103–2117. https://doi.org/10.2113/econgeo.109.8.2103
- Malinverno, A., & Ryan, W. B. F. (1986). Extension in the Tyrrhenian Sea and shortening in the Apennines as result of arc migration driven by sinking of the lithosphere. Tectonics, 5(2), 227–245. doi:10.1029/TC005i002p00227
- Manu-Marfo, D., Aoudia, A., Pachhai, S., & Kherchouche, R. (2019). 3D shear wave velocity model of the crust and uppermost mantle beneath the Tyrrhenian basin and margins Sci. Scientific Reports, 9, 3609. https://doi.org/10.1038/s41598-019-40510-z
- Marani, M. P., & Gamberi, F. (2004). Distribution and nature of submarine volcanic landforms in the Tyrrhenian Sea: the arc vs the back-arc. In M. P. Marani, F. Gamberi, & E. Bonatti (Eds.), From seafloor to deep mantle: Architecture of the Tyrrhenian backarc basin (Vol. 44, pp. 1–2). APAT, Memorie Descrittive della Carta Geologica d'Italia.
- Marani, M. P., Gamberi, F., Casoni, L., Carrara, G., Landuzzi, V., Musacchio, M., Penitenti, D., Rossi, L., & Trua, T. (1999). New rock and hydrothermal samples from the Southern Tyrrhenian Sea. Giornale Di Geologia, 29, 1–21. Issn: 0017-0291. Scopus:2-s2.0-0033503133.
- Marani, M. P., & Trua, T. (2002). Thermal constriction and slab tearing at the origin of a superinflated spreading ridge: Marsili volcano (Tyrrhenian Sea). Journal of Geophysical Research: Solid Earth, 107(B9), 2188. https://doi.org/10.1029/2001JB000285
- Nicotra, E., Passaro, S., & Ventura, G. (2024). The formation and growth mechanisms of young back-arc spreading ridges from high-resolution bathymetry: The Marsili Seamount (Tyrrhenian Sea, Italy). Geoscience Frontiers, 15(1), 101723. https://doi.org/10.1016/j.gsf.2023.101723
- Passaro, S., Milano, G., D'Isanto, C., Ruggieri, S., Tonielli, R., Bruno, P., Sprovieri, M., & Marsella, E. (2010). DTM-based morphometry of the Palinuro seamount (Eastern Tyrrhenian Sea): Geomorphological and volcanological implications. Geomorphology, 115(1–2), 129–140. https://doi.org/10.1016/j.geomorph.2009.09.041
- Petersen, S., Monecke, T., Westhues, A., Hannington, M. D., Bruce Gemmell, J., Sharpe, R., Peters, M., Strauss, H., Lackschewitz, K., Augustin, N., Gibson, H., & Kleeberg, R. (2014). Drilling Shallow-water massive sulfides at the Palinuro volcanic complex, Aeolian Island Arc, Italy. Economic Geology, 109(8), 2129–2158. https://doi.org/10.2113/econgeo.109.8.2129
- Robin, C., Colantoni, P., Gennesseaux, M., & Rehault, J. P. (1987). Vavilov seamount: A mildly alkaline Quaternary volcano in the Tyrrhenian basin. Marine geology, 78(1), 125–136. doi:10.1016/0025-3227(87)90071-5
- Sartori, R. (1990). The main results of ODP Leg 107 in the frame of neogene to recent geology of peri-Tyrrhenian areas. In K. A. Kastens, J. Mascle, et al. (Eds.), Proceedings of the ODP, scientific results (Vol. 107, pp. 715–730).
- Savelli, C., & Ligi, M. (2017). An updated reconstruction of basaltic crust emplacement in Tyrrhenian sea, Italy. Scientific Reports, 7(1), 18024. https://doi.org/10.1038/s41598-017-17625-2
- Trua, T., & Marani, M. P. (2021). Clinopyroxene crystals in basic lavas of the Marsili Volcano chronicle early magmatic stages in a back-arc transcrustal mush system. Geosciences, 11(4), 159. https://doi.org/10.3390/geosciences11040159
- Trua, T., Marani, M. P., & Gamberi, F. (2018). Magma plumbing system at a young back-arc spreading center: The Marsili Volcano, southern Tyrrhenian Sea. Geochemistry, Geophysics, Geosystems, 19(1), 43–59. https://doi.org/10.1002/2017GC007151
- Trua, T., Serri, G., Renzulli, A., Marani, M., & Gamberi, F. (2002). Volcanological and petrological evolution of Marsili seamount (southern Tyrrhenian Sea). Journal of Volcanology and Geothermal Research, 114(3–4), 441–464. doi:10.1016/S0377-0273(01)00300-6
- Ventura, G., Milano, G., Passaro, S., & Sprovieri, M. (2013). The Marsili ridge (southern Tyrrhenian Sea, Italy): An island-arc volcanic complex emplaced on a ‘relict’ back-arc basin. Earth-Science Reviews, 116, 85–94. https://doi.org/10.1016/j.earscirev.2012.11.005
- Ward, S. N. (1994). Constraints on the seismotectonics of the central Mediterranean from very long baseline interferometry. Geophysical Journal International, 117(2), 441–452. doi:10.1111/j.1365-246X.1994.tb03943.x
- Zitellini, N., Trincardi, F., Marani, M., & Fabbri, A. (1986). Neogene tectonics of the northern Tyrrhenian Sea. Giornale di Geologia, 48(1/2), 25–40.