It is well known in clinical practice that patients apparently suffering from the same illness do not necessarily respond the same way to the same medicine, both in terms of the effectiveness and side effects [Citation1]. Among the causes for this interindividual variability in drug response are physiological (age, sex, and BMI) and pathological (renal or liver diseases) conditions, as well as lifestyle-related factors (smoking, use of drugs or alcohol, etc.) and, most importantly, the genetic diversity among people, whose understanding may help explain this phenomenon [Citation2,Citation3]. In this last respect, pharmacogenetics represents a core element of biomedical research aimed at investigating the genetic basis of individual variation of drug treatment [Citation4,Citation5]. By studying interindividual variations in DNA sequence related to drug response, pharmacogenetics has the potential to provide a personalized therapy approach, based on an individual’s genetic makeup, thereby improving therapeutic efficacy and safety. In this Editorial, we discuss the main determinants of the interindividual variability in response to antidiabetic drugs in patients with type 2 diabetes mellitus (T2D).
T2D identifies a group of complex heterogeneous disorders, in which peripheral insulin resistance and pancreatic beta-cell impairment lead to hyperglycemia [Citation6]. In the recent years, T2D has emerged as a global epidemic, becoming a public health problem worldwide, with serious health-related and socioeconomic consequences [Citation7], making urgent the need for more effective therapeutic approaches. Many antidiabetic agents are currently available for treating hyperglycemia and diabetes. However, despite the recent advancement in medical therapy for T2D, clinical management of affected patients with antihyperglycemic drugs is still largely empirical and less evidence-based, with a substantial number of patients with diabetes not reaching the targets of treatment. Based on these premises, the need for clinical-based pharmacogenetic investigations oriented to the development of personalized medicine approaches in T2D is being increasingly considered [Citation8,Citation9].
Phenotypic variability among individuals, including variability in disease susceptibility and drug response, is, in part, the result of genetic polymorphisms (or variants) in the human genome. In most cases, they are single nucleotide polymorphisms (SNPs), consisting in the exchange of single nucleotides in DNA. The ability of the SNP in influencing drug response and therapeutic efficacy relies on its capacity to induce changes in gene expression, proteins, or other molecules involved in the pharmacokinetic and/or pharmacodynamic profile, hence altering the clinical efficacy of the drug. Since the introduction, in 2007, of the genome-wide association approach, many phenotypes have been associated with SNPs, which may affect disease pathogenesis, disease morbidity and severity, and therapeutic intervention [Citation10]. To date, over 80 SNPs have been identified in different loci that might be associated with the pathogenesis of T2D [Citation11–Citation14], thereby supporting the notion that, from a genetic perspective, T2D actually encompasses a heterogeneous group of distinct disorders [Citation11–Citation14], each one associated with a specific phenotype only apparently identical to one another, in which interindividual variability in terms of drug efficacy or drug-related toxicity can occur.
Thanks to the next-generation sequencing (NGS), a huge amount of sequence data has been obtained in the last years, which are contributing to a better understanding of not only the genetics of diabetes, but also the variability in response to drug exposures. Many pharmacogenetic studies of antidiabetic drugs have been performed in the recent years, identifying numerous SNPs affecting both the pharmacokinetics and pharmacodynamics of these drugs [Citation15–Citation19] (see ).
Figure 1. Gene polymorphisms that may influence therapeutic management of diabetic patients undergoing anti-hyperglycemic agent treatment.
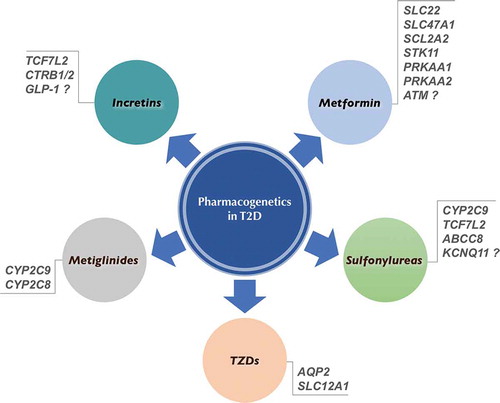
Unlike monogenic forms of diabetes, where pharmacogenetic information has led to more effective treatments [Citation20–Citation23], DNA sequence polymorphisms as genotypic markers for common T2D are still far from routine clinical application. Until now, pharmacogenetic studies of antihyperglycemic drugs in T2D have mainly concerned the organic cation transporters (OCTs) of the SLC22 gene family, which are involved in tissue distribution and urine elimination of metformin [Citation24,Citation25], and the CYP2C9 gene, which produces the CYP2C9 enzyme of the hepatic cytochrome P450 (CYP450) system, which is involved in drug metabolism.
Metformin is the first-line oral antihyperglycemic drug for people with T2D. However, individual response to metformin is highly variable, with less than two-third of treated patients reaching glycemic control [Citation8,Citation25], thus indicating that identification of SNPs associated with this variability would greatly contribute to ameliorate therapeutic treatment of these patients. The pharmacogenetic information available at present about metformin, supported by in vitro and animal studies [Citation26], indicates that polymorphisms of OCTs can alter the antihyperglycemic response to this drug, contributing to the interindividual variation in metformin response [Citation27–Citation30]. Also, recent studies in this context indicate that patients with reduced function variants in OCT1 are at increased risk for metformin intolerance and gastrointestinal side effects, which lead to the discontinuation of therapy in ~5% of cases [Citation31,Citation32]. In addition to the SLC22 variants encoding OCTs, other SNPs have been linked to the variable response to metformin, such as the SLC47A1 gene, encoding the multidrug and toxin extrusion 1 (MATE1) protein [Citation30,Citation33], the glucose transporter SCL2A2 gene [Citation34], and the STK11, PRKAA1, and PRKAA2 genes, involved in the AMP-activated protein kinase (AMPK) pathway [Citation35], while the association of a variant at the locus of the ataxia telangiectasia-mutated gene is still debated [Citation36–Citation39].
Among oral antidiabetic drugs, sulfonylureas help to lower blood glucose by closing the ATP-sensitive potassium (K-ATP) channel on pancreatic β-cells, leading to insulin secretion. As with metformin, pharmacogenetic studies of sulfonylureas in patients with common T2D have provided some important information also for this class of medications, which may help explaining heterogeneity in drug responses. In Caucasians, sulfonylureas are metabolized in the liver, primarily via the cytochrome P450 isoenzyme CYP2C9, and excreted in the urine as inactive metabolites. Polymorphisms of the CYP2C9 gene have been reported, which, by reducing the metabolism of these compounds, hence increasing drug bioavailability, can significantly influence the response of diabetic patients to sulfonylureas and the risk of hypoglycemia [Citation40,Citation41]. The risk of hypoglycemia in patients carrying mutations in CYP2C9 is supported by population-based studies, in which genetic variations of CYP2C9 are associated with the improvement in glycemic control parameters, including HbA1c [Citation42]. Interesting findings in this area come from studies of the TCF7L2 gene, which plays a role in β-cell function. Variation in the therapeutic efficacy of sulfonylureas (either in terms of improvement or deterioration in glycemic control) has been reported among diabetic patients carrying variants of this gene [Citation43,Citation44]. In relation to the important role of the K-ATP channel/sulfonylurea receptor for these antidiabetic compounds, a protective variant of the ABCC8 sulfonylurea receptor gene has been described in Chinese diabetic patients [Citation45], whereas conflicting results have been reported for the KCNQ11 gene, encoding for the pore-forming subunits kir.6.2 [Citation46,Citation47]. Therefore, at present, genotyping of CYP2C9 and TCF7L2 may provide useful information in predicting the effects and risks of sulfonylureas, thereby assisting physicians in clinical and therapeutic management of diabetic patients undergoing antihyperglycemic agent treatment.
As agonists of PPAR-γ, the thiazolidinediones (TZDs), pioglitazone, and rosiglitazone, act as insulin-sensitizing drugs, thus improving insulin resistance in patients with T2D. Pharmacogenetic studies with TZDs have reported equivocal results, probably because of the small sample size and the low levels of statistical power [Citation48]. It is important to note, in this context, the identification of genetic variants in AQP2 and SLC12A1 genes, whose protein products are involved in kidney’s water and sodium reabsorption, respectively. As adverse effects of TZDs may include fluid retention and peripheral edema (worsening heart failure) [Citation49], variants of AQP2 and SLC12A1 may represent a risk factor in TZD-treated patients.
Metiglinides, such as repaglinide and nateglinide, are recently developed shorter-acting insulin secretagogues. Like sulfonylureas, they act by inhibiting the K-ATP channel on β-cells, thus forcing pancreas to make more insulin, and are metabolized through the CYP450 enzyme system. Also in this case, genetic polymorphisms of the P450 enzyme system, specifically CYP2C9 and CYP2C8, have been associated with variability in glucose-lowering effect of repaglinide and nateglinide, respectively [Citation50–Citation52].
Incretin-based treatments include GLP-1 analogs (liraglutide and exenatide) and DPP-4 inhibitors (gliptins), both of which potentiate meal-stimulated insulin secretion. Variants in GLP-1 receptor gene have been reported, which may alter insulin secretion in response to glucose [Citation53]. However, whether these variations are responsible for the interindividual variability in response to these medications is still not certain. Despite this, also in this context variants in TCF7L2 and CTRB1/2 (a known diabetes risk locus) appear to reduce the efficacy of exogenous GLP-1 on insulin release [Citation54].
Recently, new scenarios are emerging by studies on the joint effects of multiple type 2 diabetes-related loci on the efficacy of antidiabetic drugs, which may be potentially useful to predict drug response [Citation55].
Globally, the above findings well support the concept that genetic variations associated with T2D can affect the therapeutic efficacy of antihyperglycemic drugs in patients with T2D. Nevertheless, up to date, drug therapy in diabetic subjects has not taken into consideration the genetic diversity of patients, and pharmacogenetics in diabetes is still in a preliminary stage. Consequently, the possibility for individualized therapy is yet far from being actualized, and interindividual variability in diabetic patients’ response to antihyperglycemic agents is still a common concern. Among the reasons for this, it can be cited the lack, at this time, of standard clinical indications for the use of pharmacogenetic testing in clinical practice, as well as the lack of robust evidence demonstrating clinical usefulness of pharmacogenetics in diabetic patients under antidiabetic drugs. It is desirable that the recent advances in the omics-related technologies (transcriptomics, proteomics, metabolomics, and epigenenomics), together with better-designed studies with standardized assays and a larger number of patients, will soon allow to overcome these limitations.
We hope this editorial can help clinicians practicing diabetology to better understand the interindividual variability in diabetic patients’ response to antihyperglycemic agents and to establish corrective actions to optimize therapeutic management of these patients.
Declaration of interest
The authors have no relevant affiliations or financial involvement with any organization or entity with a financial interest in or financial conflict with the subject matter or materials discussed in the manuscript. This includes employment, consultancies, honoraria, stock ownership or options, expert testimony, grants or patents received or pending, or royalties.
Additional information
Funding
References
- Eichelbaum M, Evert B. Influence of pharmacogenetics on drug disposition and response. Clin Exp Pharmacol Physiol. 1996;23(10–11):983–985.
- Prescott LF. Pathological and physiological factors affecting drug absorbtion, distribution, elimination, and response in man. In: Gillette JR, Mitchell JR, eds., From: concepts in biochemical pharmacology. Berlin - Heidelberg: Springer-Verlag; 1975. p. 234–257.
- Madian AG, Wheeler HE, Baker Jones R, et al. Relating human genetic variation in drug responses. Trends Genet. 2012;28(10):487–495.
- Roses AD. Pharmacogenetics and the practice of medicine. Nature. 2000;405(6788):857–865.
- Meyer UA. Pharmacogenetics and adverse drug reactions. Lancet. 2000;356(9242):1667–1671.
- American Diabetes Association. Classification and diagnosis of diabetes. Diabetes Care. 2017;40(Suppl. 1):S11–S24.
- IDF Diabetes Atlas – 7th Edition. [cited 2017 Apr 1]. Available from www.diabetesatlas.org
- Brunetti A, Brunetti FS, Chiefari E. Pharmacogenetics of type 2 diabetes mellitus: an example of success in clinical and translational medicine. World J Transl Med. 2014;12(3):141–149.
- Klonoff DC. Precision medicine for managing diabetes. J Diabetes Sci Technol. 2015;9(1):3–7.
- Motsinger-Reif AA, Jorgenson E, Relling MV, et al. Genome-wide association studies in pharmacogenomics: successes and lessons. Pharmacogenet Genomics. 2013;23(8):383–394.
- Brunetti A, Chiefari E, Foti D. Perspectives on the contribution of genetics to the pathogenesis of type 2 diabetes mellitus. Recenti Prog Med. 2011;102(12):468–475.
- Fuchsberger C, Flannick J, Teslovich TM, et al. The genetic architecture of type 2 diabetes. Nature. 2016;536(7614):41–47.
- Mohlke KL, Boehnke M. Recent advances in understanding the genetic architecture of type 2 diabetes. Hum Mol Gen. 2015;24(R1):R85–92.
- Chiefari E, Tanyolaç S, Paonessa F, et al. Functional variants of the HMGA1 gene and type 2 diabetes mellitus. Jama. 2011;305(9):903–912.
- Maruthur NM, Gribble MO, Bennett WL, et al. The pharmacogenetics of type 2 diabetes: a systematic review. Diabetes Care. 2014;37(3):876–886.
- Manolopoulos VG, Ragia G, Tavridou A.Pharmacogenomics of oral antidiabetic medications: current data and pharmacoepigenomic perspective. Pharmacogenomics. 2011;12(8):1161–1191.
- Pearson ER. Pharmacogenetics in diabetes. Curr Diab Rep. 2009;9(2):172–181.
- Huang C, Florez JC. Pharmacogenetics in type 2 diabetes: potential implications for clinical practice. Genome Med. 2011;3(11):76.
- Kleinberger JW, Pollin TI. Personalized medicine in diabetes mellitus: current opportunities and future prospects. Ann N Y Acad Sci. 2015;1346(1):45–56.
- Flanagan SE, Clauin S, Bellanne-Chantelot C, et al. Update of mutations in the genes encoding the pancreatic beta-cell K(ATP) channel subunits Kir6.2 (KCNJ11) and sulfonylurea receptor 1 (ABCC8) in diabetes mellitus and hyperinsulinism. Hum Mutat. 2009;30(2):170–180.
- Pearson ER, Flechtner I, Njolstad PR, et al. Switching from insulin to oral sulfonylureas in patients with diabetes due to Kir6.2 mutations. N Engl J Med. 2006;355(5):467–477.
- Pearson ER, Liddell WG, Shepherd M, et al. Sensitivity to sulphonylureas in patients with hepatocyte nuclear factor-1alpha gene mutations: evidence for pharmacogenetics in diabetes. Diabet Med. 2000;17(7):543–545.
- Jesic MD, Sajic S, Jesic MM, et al. A case of new mutation in maturity-onset diabetes of the young type 3 (MODY 3) responsive to a low dose of sulphonylurea. Diabetes Res Clin Pract. 2008;81(1):e1–3.
- Viollet B, Guigas B, Sanz Garcia N, et al. Cellular and molecular mechanisms of metformin: an overview. Clin Sci (Lond). 2012;122(6):253–270.
- Todd JN, Florez JC. An update on the pharmacogenomics of metformin: progress, problems and potential. Pharmacogenomics. 2014;15(4):529–539.
- Shu Y, Sheardown SA, Brown C, et al. Effect of genetic variation in the organic cationic transporter 1 (OCT1) on metformin action. J Clin Invest. 2007;117(5):1422–1431.
- Zhou K, Donnelly LA, Kimber CH, et al. Reduced-function SLC22A1 polymorphisms encoding organic cation transporter 1 and glycemic response to metformin: a GoDARTS study. Diabetes. 2009;58(6):1434–1439.
- Shu Y, Brown C, Castro RA, et al. Effect of genetic variation in the organic cationic transporter 1, OCT1, on metformin parmacokinetics. Clin Pharmacol Ther. 2008;83(2):273–280.
- Becker ML, Visser LE, Van Schalk RH, et al. Genetic variation in the organic cation transporter 1 is associated with metformin response in patients with diabetes mellitus. Pharmacogenomics J. 2009;9(4):242–247.
- Tkac I, Klimcakova L, Javorsky M, et al. Pharmacogenomic association between a variant in SLC47A1 gene and therapeutic type response to metformin in 2 diabetes. Diabetes Obes Metab. 2013;15:189–191.
- Dujic T, Zhou K, Donnelly LA, et al. Association of organic cation transporter 1 with intolerance to metformin in type 2 diabetes: a GoDARTS study. Diabetes. 2015;64(5):1786–1793.
- Dujic T, Causevic A, Bego T, et al. Organic cation transporter 1 variants and gastrointestinal side effects of metformin in patients with Type 2 diabetes. Diabet Med. 2016;33(4):511–514.
- Becker ML, Visser LE, Van Schaik RH, et al. Genetic variation in the multidrug and toxin extrusion 1 transporter protein influences the glucose-lowering effect of metformin in patients with diabetes: a preliminary study. Diabetes. 2009;58(3):745–749.
- Zhou K, Yee SW, Seiser EL, et al. Variation in the glucose transporter gene SLC2A2 is associated with glycemic response to metformin. Nat Genet. 2016;48(9):1055–1059.
- Jablonski KA, McAteer JB, De Bakker PI, et al. Common variants in 40 genes assessed for diabetes incidence and response to metformin and lifestyle intervention in the diabetes prevention program. Diabetes. 2010;59(10):2672–2681.
- Zhou K, Bellenguez C, Spenser CCA, et al. Common variants near ATM are associated with glycemic response to metformin in type 2 diabetes. Nat Genet. 2011;43(2):117–120.
- Florez JC, Jablonski KA, Taylor A, et al. The C allele of ATM rs11212617 does not associate with metformin response in the diabetes prevention program. Diabetes Care. 2012;35(9):1864–1867.
- van Leeuwen N, Nijpels G, Becker ML, et al. A gene variant near ATM is significantly associated with metformin treatment response in type 2 diabetes: a replication and meta-analysis of 5 cohorts. Diabetologia. 2012;55(7):1971–1977.
- Tkac I. Replication of the association of gene variant near ATM and response to metformin. Pharmacogenomics. 2012;13(12):1331–1332.
- Ragia G, Petridis I, Tavridou A, et al. Presence of CYP2C9*3 allele increases risk for hypoglycemia in Type 2 diabetic patients treated with sulfonylureas. Pharmacogenomics. 2009;10(11):1781–1787.
- Holstein A, Hahn M, Patzer O, et al. Impact of clinical factors and CYP2C9 variants for the risk of severe sulfonylurea-induced hypoglycemia. Eur J Clin Pharmacol. 2011;67(5):471–476.
- Zhou K, Donnelly L, Burch L, et al. Loss-of-function CYP2C9 variants improve therapeutic response to sulfonylureas in type 2 diabetes: a Go-DARTS study. Clin Pharmacol Ther. 2010;87(1):52–56.
- Schroner Z, Javorsky M, Tkacova R, et al. Effect of sulphonylurea treatment on glycaemic control is related to TCF7L2 genotype in patients with type 2 diabetes. Diabetes Obes Metab. 2011;13(1):89–91.
- Holstein A, Hahn M, Körner A, et al. TCF7L2 and therapeutic response to sulfonylureas in patients with type 2 diabetes. BMC Med Genet. 2011;12:30.
- Feng Y, Mao G, Ren X, et al. Ser1369Ala variant in sulphonylurea receptor gene ABCC8 is associated with antidiabetic efficacy of gliclazide in Chinese type e diabetic patients. Diabetes Care. 2008;31(10):1939–1944.
- Schroner Z, Dobrikova M, Klimcakova L, et al. Variation in KCNQ11 is associated with therapeutic response to sulphonylureas. Med Sci Monit. 2011;17(7):CR392–6.
- Gloyn AL, Hashim Y, Ashcroft SJ, et al. Association studies of variants in promoter and coding regions of beta-cell ATP-sensitive K-channel genes SUR1 and Kir6.2 with type 2 diabetes mellitus (UKPDS 53). Diabetes Med. 2001;18(3):206–212.
- Becker ML, Pearson ER, Tkáč I. Pharmacogenetics of oral antidiabetic drugs. Int J Endocrinol. 2013;2013:686315.
- Karalliedde J, Buckingham RE. Thiazolidinediones and their fluid-related adverse effects: facts, fiction and putative management strategies. Drug Saf. 2007;30(9):741–753.
- Kirchheiner J, Roots I, Goldammer M, et al. Effect of genetic polymorphisms in cytochrome p450 (CYP) 2C9 and CYP2C8 on the pharmacokinetics of oral antidiabetic drugs: clinical relevance. Clin Pharmacokinet. 2005;44(12):1209–1225.
- Tomalik-Scharte D, Fuhr U, Hellmich M, et al. Effect of the CYP2C8 genotype on the pharmacokinetics and pharmacodynamics of repaglinide. Drug Metab Dispos. 2011;39(5):927–932.
- Chen M, Hu C, Jia W. Pharmacogenomics of glinides. Pharmacogenomics. 2015;16(1):45–60.
- Sathananthan A, Man CD, Micheletto F, et al. Common genetic variation in GLP1R and insulin secretion in response to exogenous GLP-1 in nondiabetic subjects: a pilot study. Diabetes Care. 2010;33(9):2074–2076.
- ‘t Hart LM, Fritsche A, Nijpels G, et al. The CTRB1/2 locus affects diabetes susceptibility and treatment via the incretin pathway. Diabetes. 2013;62(9):3275–3281.
- Chen M, Zhang R, Jiang F, et al. Joint effects of diabetic-related genomic loci on the therapeutic efficacy of oral anti-diabetic drugs in Chinese type 2 diabetes patients. Sci Rep. 2016;6:23266.