ABSTRACT
Objective: Effective treatment for primary Sjögren’s syndrome (pSS) is not available. pSS immunopathology involves a variety of immune-cells and dysregulated pathways; targeting several pathways instead of only one could therefore be effective. Treatment with leflunomide (LEF) and hydroxychloroquine (HCQ) might be successful given their unique immunosuppressive properties. We aimed to study the in vitro effects of LEF, HCQ and their combination on T- and B-cell proliferation, cytokine and immunoglobulin production by activated PBMCs.
Methods: PBMCs of six healthy individuals and nine pSS patients were stimulated with superantigen and TLR9 agonist to mimic the hallmark features. LEF, HCQ and their combinations were tested at clinically observed concentrations and proliferation, cytokine and immunoglobulin production were measured.
Results: TCR/TLR9 activation of PBMCs induced strong proliferation of T and B-cells and production of CXCL13, IFN-α, IFN-γ, IgG and IgM. LEF dose-dependently inhibited all measured parameters, where HCQ potently and dose-dependently decreased B cell proliferation, CXCL13, IFN-α, IgG and IgM production. At different concentration combinations, HCQ and LEF inhibited several immune hallmark features more potently than each single compound.
Conclusion: A combination of LEF and HCQ at clinically applicable concentrations additively inhibits immune activation, supporting a potential implementation of this drug combination in pSS treatment.
1. Introduction
Despite an extensive search for a clinically effective treatment for primary Sjögren’s syndrome (pSS), to this day standard care comprises only symptomatic treatment with saliva- and tear substitutes to relieve the hallmark symptoms of pSS, severe dryness of eyes and mouth. Even though the precise immunopathological processes of pSS remain to be elucidated, evolving knowledge in recent years has prompted many efforts to target one or more of these processes specifically, either by biological DMARDs (bDMARDs), small drug molecules or repurposing existing drugs like classical synthetic DMARDs (csDMARDs) [Citation1].
The salivary glands of patients with pSS are infiltrated by a range of immune cells, mainly CD4 and CD8 T-cells, B-cells, and to a lesser extent dendritic cells (DCs), monocytes/macrophages and NK-cells [Citation2]. A complex interplay between these cells and their effector molecules results in chronic inflammation with B cell hyperactivity, auto-antibody production and ultimately formation of ectopic germinal centers [Citation3,Citation4]. Reflecting B cell hyperactivity, ~30% of pSS patients develop extraglandular manifestations and 5–10% of patients with pSS develop B-cell lymphoma, in particular those patients with high numbers of lymphocytic foci or germinal centers [Citation2,Citation5–Citation7].
A clear role for the interferon pathway has been shown in pSS [Citation8–Citation11]. The presence of an interferon signature is associated with B cell hyperactivity and clinical parameters [Citation12]. Systemically, an interferon type I is present in approximately 55% of pSS patients. This type I IFN signature has been attributed to production of type I IFNs (e.g. IFN-α) by plasmacytoid DCs (pDCs). In target organs, a predominant upregulation of interferon type II inducible genes was demonstrated [Citation13], which is associated with the abundant presence of IFN-γ-producing T cells [Citation14]. IFN-α induces Tfh cells expressing Bcl6 and triggers B cell activation [Citation15]. Interestingly, recent studies demonstrate that also IFN-γ is a critical inducer of germinal center formation and autoimmunity. Furthermore, pathogenic Tfh cells co-express IL-21 and IFN-γ [Citation16–Citation18].
Ectopic germinal centers that develop in 25–30% of pSS patients [Citation3–Citation5], exhibit functional characteristics of lymphoid germinal centers, including B cell affinity maturation and clonal selection [Citation3]. Presence of GCs in pSS is associated with high disease activity and strongly increased risk of lymphoma [Citation5,Citation19]. CXCL13 and its unique receptor CXCR5 are crucial in formation, function, and maintenance of GCs by regulating homing and tissue localization of CXCR5-expressing Tfh cells and B cells. In pSS patients, CXCR5 Tfh cells in peripheral blood and labial salivary gland (LSG) are increased and in LSG CXCR5 Tfh cells correlate with percentages of memory B cells and plasma cells. In line with this observation, the degree of cellular organization of the lymphocytic aggregate is positively associated with the CXLC13 expression. These data implicate a critical role for the CXCL13/CXCR5 axis in B cell hyperactivity and GC formation in pSS patients [Citation4].
The involvement of different immune cells and effector mechanisms in pSS pathology indicates that targeting multiple immunopathological pathways could lead to an improved inhibition of disease activity. Combining csDMARDs with overlapping but also distinct anti-inflammatory activities has proven to be a successful strategy in for instance rheumatoid arthritis. In pSS, the csDMARDs leflunomide (LEF) and hydroxychloroquine (HCQ), when given as monotherapy, inhibited immunological parameters in clinical trials [Citation20,Citation21], although suboptimal clinical effects were seen for these monotherapies [Citation20–Citation22]. Considering their complementary mechanisms of action, a combination of LEF and HCQ could have beneficial effects on immunological and clinical parameters in patients with pSS.
Leflunomide (LEF) is an isoxazole derivate that, after in vivo conversion to its active form (A77 1726), becomes biologically active. It inhibits dihydro-orotate dehydrogenase (DHODH), which is the rate-limiting enzyme of de novo synthesis of pyrimidines causing inhibition of (CD4) T cell proliferation [Citation23]. In mice, LEF inhibits both T cell-dependent and T cell-independent B cell antibody production by blocking cell cycle transition, thereby limiting the expansion of antibody-producing cells [Citation24].
Hydroxychloroquine (HCQ), initially used as an anti-malarial drug, also exhibits immunomodulatory effects. HCQ mainly targets antigen-presenting cells such as dendritic cells and monocytes, illustrated by the fact that after exposure these cells have higher intracellular concentrations of the drug [Citation25]. Antigen presentation is decreased by HCQ, as MHC class II molecules are moved to the endocytic compartments after synthesis. This mainly affects binding of low-affinity self-peptides instead of antigenic pathogen-derived peptides [Citation25]. HCQ inhibits activity of pDCs, which are the strongest producers of type I IFN, and of B cells, by inhibiting Toll-Like receptor (TLR)-mediated responses [Citation25,Citation26]. This is due to the HCQ-mediated raise of endosomal pH leading to dysfunction of endosomal TLRs. By raising endosomal pH, HCQ interferes with the functional transformation of the TLRs needed for their activation [Citation27]. Also, HCQ directly interferes with nucleic acid TLR ligands, resulting in structural alterations of the nucleic acid and prevention of its binding to TLRs [Citation28]. In addition, HCQ inhibits autophagy, resulting in less cell proliferation [Citation29].
Considering the distinct immune cells and effector mechanisms targeted by LEF and HCQ, and the potential for their combined use, we here assessed the in vitro effects of a combination of these drugs on TCR/TLR-activated PBMCs. We show that the combined use of LEF and HCQ leads to strong additive inhibition of T and B cell proliferation, resulting in decreased production of T follicular helper (Tfh)-related cytokine CXCL13, type I and type II IFNs and immunoglobulins.
2. Materials and methods
2.1. Patients
Nine pSS patients who fulfilled the American-European Consensus Group classification criteria for primary Sjögren’s syndrome [Citation30], as well as six healthy individuals, were included. Characteristics of the patients and healthy controls are given in . The study was performed according to the regulations of the medical ethical committee of the University Medical Centre Utrecht. All patients and healthy individuals gave their written informed consent.
Table 1. Characteristics of the controls (n = 6) and patients (n = 9).
2.2. Cell culture
Mononuclear cells (MCs) from patients and controls were isolated from heparinized peripheral blood (PB) by density centrifugation using Ficoll-Paque Plus (GE Healthcare, Uppsala, Sweden). Prior to MC isolation, PB was diluted 1:1 in PBS. Peripheral blood mononuclear cells (PBMCs) were stimulated with superantigen Staphylococcal enterotoxin B (SEB) (Sigma–Aldrich, Zwijndrecht, the Netherlands), CPG-C ODN-M362 (Invivogen, San Diego, USA) and their combination, in the presence or absence of clinically relevant concentrations of HCQ (Sigma–Aldrich) and A77 1726 (MedChemExpress, Monmouth Junction, USA), the active metabolite of LEF. For analyses of cytokine production and cell proliferation, supernatant and cells were harvested after four days of culture. For analyses of immunoglobulin production, supernatants were harvested after 10 days of culture. Culture supernatants were rendered cell-free and stored at −80 C prior to analysis.
2.3. Flow cytometry
Proliferation of T cells and B cells was assessed, using the Cell Trace Violet technique (Life technologies, Carlsbad, California, USA). This intracellular dye dilutes out following every cell division and is measured by Fluorescence-activated cell sorting (FACS). For analysis of cytokine production and proliferation, a viability dye staining (Fixable Viability Dye eFluor©780, Thermo Fisher, Waltham, USA) was added to exclude cell death as an explanation for inhibition of read-outs. To distinguish cell subsets in flow-cytometry analyses, the following antibody panel was used: CD3 V500 (BD Biosciences), CD4 PerCP-Cy5.5 (Sony Biotechnology), CD45ROPE-Cy7 (BD Bioschiences), CD19 AF700 (eBioscience), CD14 FITC (Miltenyi) and CD25 BV711 (BD Biosciences). Data were analyzed using FlowJo (version 10).
2.4. Measurement of cytokines and immunoglobulins
Cytokine production was measured using an in-house developed and validated multiplex immunoassay as described previously [Citation31]. IgG and IgM levels in the supernatants taken after 10 days of culture were determined by ELISA according to manufacturer’s instructions (Bethyl Laboratories, Montgomery, USA).
2.5. Statistics
To compare groups and conditions, the paired T-test was used. To test the additive effects of LEF and HCQ, paired T-tests were applied. Differences were considered to be statistically significant at p < 0.05.
3. Results
Stimulation of PBMCs with SEB and TLR-9L induces strong proliferation of T and B cells, as well as production of cytokines and immunoglobulins
To study the combined effects of LEF and HCQ in vitro, we sought to setup a culture system that reflects activation of multiple immune cell types, and effector molecules as observed in patients with pSS. As SEB strongly induces T cell receptor-mediated T cell activation in a large proportion of T cells and TLR9 ligand CPG-C is a robust activator of B cells and pDCs, we investigated whether the combination of these stimuli resulted in activation of these three cell subsets. After four days of culturing PBMCs in the presence of both SEB and TLR9 ligand, we observed strong proliferation of both T cells and B cells ()). This was true for cells from both healthy controls and patients with pSS, and there were no apparent differences between patients and controls ()). In line with the robust T and B cell proliferation after four days, high levels of Tfh-related cytokine CXCL13 and type I and II interferons IFN-α and IFN-γ, were measured upon activation. After 10 days of culture, we observed strongly increased levels of IgG and IgM production upon stimulation ()). Judging from the strongly enhanced proliferation and production of IFNs and immunoglobulins, this culture system was used as a model to study the inhibitory effect of LEF and HCQ on activation of T cells, B cells and pDCs.
Figure 1. In vitro model to assess efficacy of csDMARDs to inhibit lymphocyte proliferation, cytokine- and immunoglobulin production. (a) Induction of both T- and B-cell proliferation upon TCR stimulation with SEB and TLR9 stimulation with CpG-C. % proliferating T cells and B cells are indicated in light grey and dark grey, respectively. (b) Induction of T and B cell proliferation and (c) cytokine and immunoglobulin production upon stimulation with SEB and TLR9 in healthy controls (HC) and pSS patients.
*, **, *** indicate statistical significant differences of p < 0.05, 0.01 and 0.001.
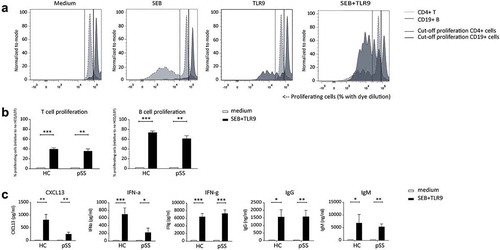
3.1. HCQ and LEF distinctly inhibit lymphocyte proliferation, cytokine and Ig production
We next investigated the dose dependency of LEF or HCQ to inhibit activation of PBMCs cultured with SEB and TLR-9L. Several concentrations of either cDMARD were tested on cells from both patients and controls. For both drugs, the highest condition was chosen based on mean serum levels that are seen in patients treated with clinically usual dosages. For LEF, mean serum levels are approximately 30–40 mg/l [Citation21,Citation32] (this corresponds to 111–148 μM) and a minimum concentration of 13–16 mg/l (48–59 μM) is considered minimally required for clinical response [Citation32]. Therefore, 100 μM was chosen as the highest concentration. Studies in SLE patients suggest a minimum effective blood concentration of 500 ng/ml (1.15 μM) of HCQ, however others claim that a minimum of 1000 ng/ml (2.3 μM) is required for therapeutic efficacy [Citation33,Citation34]. Therefore, the optimal therapeutic range of HCQ remains to be determined [Citation33]. However, a great variability is seen in serum HCQ concentration among individuals, more than a 10-fold range of drug concentrations was found in patients all receiving similar doses [Citation34]. Therefore, a concentration of 4300 ng/ml (this corresponds to 10 μM) was chosen as the highest condition.
HCQ by itself potently inhibited B cell proliferation ()), IFN-α, CXCL13 ()), and immunoglobulin production ()) in a dose-dependent manner. T cell proliferation was only inhibited at the highest concentration of HCQ. LEF alone dose-dependently inhibited proliferation of T and B cells, production of cytokines CXCL13, IFN-α and IFN-γ and production of IgG and IgM.
Figure 2. Inhibition of proliferation, cytokine and immunoglobulin production by HCQ and LEF in HC and pSS-patients. HCQ alone potently inhibited B cell proliferation (A), IFN-α, CXCL13 (B), and immunoglobulin production (C) in a dose-dependent manner. T cell proliferation was only inhibited at the highest concentration of HCQ. LEF alone dose-dependently inhibited proliferation of T and B cells (a), production of cytokines CXCL13, IFN-α and IFN-γ (b) and production of IgG and IgM (c).
*, **, *** indicate statistical significant differences of p < 0.05, 0.01 and 0.001.
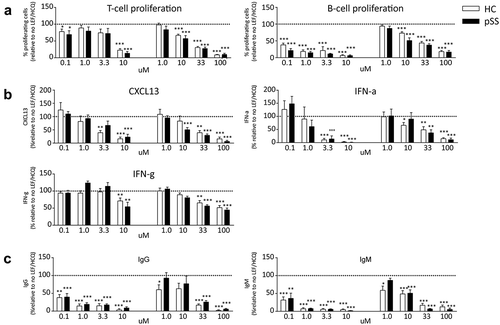
3.2 Suboptimal concentrations of the HCQ/LEF combination additively inhibit T cell proliferation, B cell proliferation and CXCL13 production (in pSS patients) and inhibit IFN-α and immunoglobulins
Next, we investigated the effects of the combination of csDMARDs in this culture system. We tested different combinations of LEF and HCQ in a checkerboard setup and determined the concentration at which optimal additive inhibition of T and B cell proliferation was observed. At a concentration of 3.3 μM HCQ and 10 μM LEF, which are submaximal dosages of each drug, T cell and B cell proliferation were additively inhibited in HCs and pSS patients, compared to the addition of either HCQ or LEF alone ()).
Figure 3. Additive inhibition of proliferation and CXCL13 production and robust inhibition of Igs and IFN-? Additive inhibition of T- and B-cell proliferation and production of CXCL13 and robust inhibition of IgG, IgM, and IFN-? is achieved by suboptimal concentrations of HCQ and LEF in HC and pSS patients. (a): T cell proliferation is additively inhibited by HCQ and LEF both in HC and in pSS patients. Combination of HCQ and LEF leads to profound inhibition of B cell proliferation (b): Robust inhibition of CXCL13 and IFN-? production in HC and pSS patients. IFN-? production remains unchainged (c): IgG and IgM are strongly inhibited by HCQ and LEF in HC and pSS patients.
*, **, *** indicate statistical significant differences of p < 0.05, 0.01 and 0.001.
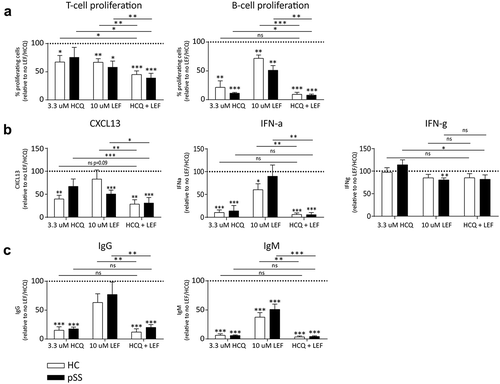
In pSS patients, CXCL13 production was also strongly and additively inhibited by the combination of 3.3 μM HCQ and 10 μM LEF. A trend towards additive inhibition was seen in HCs, but statistical significance was not reached. The combination of 3.3 μM HCQ and 10 μM LEF induced robust inhibition of IFN-α, but no additive effect was observed since HCQ alone already induced strong almost complete inhibition.
Inhibition of IFN-γ was seen at a concentration of 10 μM HCQ (clinically relevant concentration) and 33 and 100 μM LEF (suboptimal and clinically relevant concentration, respectively) (, )). At 3.3 μM HCQ and 10 μM LEF inhibition of IFN-γ production was absent or only minor. For both IgG and IgM, the combination of 3.3 μM HCQ and 10 μM LEF strongly reduced production, but this was not significantly different from HCQ alone since this by itself strongly diminished Ig production (, )).
Concentrations of LEF and HCQ that optimally inhibited lymphocyte proliferation did not show significant additive effects on cytokine and immunoglobulin production, because HCQ had a very strong effect by itself. As such, we investigated whether suboptimal concentrations of HCQ would show more pronounced additive effects on Ig production. Using a concentration of 0.1 μM HCQ and 33 μM LEF significant additive inhibition of IgG for both healthy individuals and pSS patients was observed. This was also seen in pSS patients for IgM production, although in healthy individuals only a trend towards additive inhibition was seen. Similar results were seen for combinations of LEF with 1.0 μM HCQ (data not shown).
3.3. Optimal concentrations of LEF and HCQ additively inhibit production of IFN-γ and immunoglobulins
The combination of the highest concentrations of the two drugs, 10 μM HCQ and 100 μM LEF, resulted in significant additive and profound inhibition of IFN-γ (). Cell death was measured in all conditions in patients and healthy donors and did not significantly differ between donors and patients. In none of the conditions, cell death exceeded 8% on average (see Supplementary figure 1) not correlating with immune inhibitory effects, thus excluding cell death as a cause for inhibition of proliferation, cytokine and immunoglobulin production.
Figure 4. Additive inhibition of IFN-γ and B cell activity (IgG and IgM). Additive inhibition of IFN-γ and immunoglobulins is achieved using either higher (IFN-γ) or suboptimal (IgG and IgM) concentrations of HCQ and LEF.
*, **, *** indicate statistical significant differences of p < 0.05, 0.01 and 0.001.
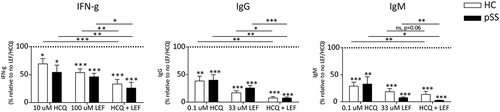
4. Discussion
Both HCQ and LEF separately inhibit T and B cell activation, with HCQ extremely potently inhibiting B cell activation and LEF more potently inhibiting T cell activation. At different combinations of HCQ and LEF, additive inhibition of in vitro proliferation of T and B cells, production of Tfh-associated cytokine CXCL-13, IFN-y, and B cell activity was observed.
Dosages of HCQ and LEF were chosen based on serum levels seen in patients treated with the usual dosage of these drugs, with the highest concentration in vitro set as the highest concentration observed in vivo and subsequent testing of lower concentrations. With respect to HCQ, studies in SLE patients suggest a minimum effective blood concentration of 500 ng/ml (1.15 μM) [Citation33], others claim that a minimum of 1000 ng/ml (2.3 μM) is required for therapeutic efficacy [Citation33,Citation34]. Our data show that at 3.3 μM HCQ in vitro robustly inhibits B cell activity, IFN-α and to a lesser extent CXCL13 production. Also at the lower clinically suggested concentration (1 μM) HCQ in vitro extremely well inhibits B cell activation. This is line with the observed effect of HCQ [Citation20] on sIgG levels in pSS patients that resembles those of the biologicals Rituximab [Citation35], Abatacept [Citation36,Citation37] and Belimumab [Citation38]. Interestingly, our study shows that HCQ in much lower concentrations, even up to 0.1 μM, is capable of inhibiting B cell activation. Since at this concentration no inhibition of T cell proliferation, CXCL13, IFNγ or IFNα production was observed HCQ seems to target B cells independent of these processes.
We demonstrate that both LEF and HCQ inhibit T and B cell proliferation and production of CXCL13, IFN-α, IFN-γ and immunoglobulins IgG and IgM, all of which are important contributors to pSS pathology, albeit with different potencies. LEF dose-dependently inhibits proliferation of T- and B cells, CXCL13, IFN-α, IFN-γ, and immunoglobulins IgG and IgM. HCQ in a dose-dependent manner inhibits proliferation of B cells, production of CXCL13, IFN-α and immunoglobulins, however T cell proliferation and IFNγ were inhibited much less potent, only at the highest HCQ concentration.
For the first time, we showed that a combination of LEF and HCQ induced complementary and additive inhibition of TCR/TLR9 activated immune activation. We observed the most robust additive effects on B and T cell proliferation and CXCL13 using suboptimal dosages of LEF and HCQ. In addition, optimal inhibition of IFN-α and B cell activity reflected by IgG and IgM production was achieved at these concentrations. Hence, the key pathways involved in pSS pathology are successfully targeted by this combination.
Recently it was shown that IFN-γ plays a critical role in GC formation and induction of autoimmunity. IFN-γ was one of the more persistent cytokines in our in vitro model. Significant inhibition of IFN-γ production required higher concentrations of HCQ (10 μM) and LEF (100 μM), concentrations that in vivo can be easily reached for LEF, but less easy for HCQ when patients are treated with the usual dosage. A combination of 10 μM HCQ and 100 μM LEF did result in significant and additive inhibition of IFN-γ production. Trends for additive inhibition were observed when using a lower concentration of HCQ, but did not reach statistical significance. This suggests that the local IFN-γ production in pSS could persist despite broad immune inhibition with HCQ and LEF in clinically relevant concentrations, requiring higher concentrations of HCQ and LEF.
Despite huge efforts to successfully inhibit disease activity in pSS, results of numerous clinical studies, including those testing biologicals such as rituximab, have been rather disappointing [Citation1,Citation39], and new promising drugs require confirmation in larger studies. Notwithstanding, treatment with, for example, Rituximab has been demonstrated to lead to biological effects, including mitigation of T and B cell activation, glandular inflammation, formation of ectopic lymphoid structures and B cell hyperactivity (reflected by reduction of serum IgG and RF) [Citation40–Citation43]. Still, inhibition of B cell hyperactivity may only be partially, insufficient to fully tackle immunopathology and disease activity [Citation44] [Citation35,Citation45]. Interestingly, upon investigation of the biological effects of LEF/HCQ combination therapy in a double-blind randomized controlled clinical trial we recently performed (EudraCT 2014-003140-12) we found an unprecedented strong inhibition of B cell hyperactivity as reflected by reduction in serum IgG [Citation46]. This was associated by significant inhibition of several clinical measures, including the primary outcome, the ESSDAI score. Whether LEF/HCQ combination therapy truly culminates into higher clinical efficacy remains to be confirmed in larger clinical trials.
5. Conclusion
Our data show that the combination of LEF and HCQ potently inhibits several hallmark immune responses in vitro that are indicated to play key roles in immunopathology of pSS. To confirm these favorable in vitro results, we are currently investigating the clinical and immunological effects of combination therapy with HCQ and LEF in pSS patients. Publication of the results of this double-blind, randomized controlled clinical trial is eagerly awaited.
Article highlights
Stimulation of PBMCs with SEB and TLR-9L induces strong proliferation of T and B cells, as well as production of cytokines and immunoglobulins.
Leflunomide inhibits in vitro proliferation of lymphocytes, most strongly T lymphocytes and halts production of CXCL13, IFN-α, IFN-γ, and immunoglobulins.
Hydroxychloroquine in vitro most strongly inhibits proliferation of B-cells, production of CXCL13, IFN-α, and immunoglobulins.
Their combination additively decreases lymphocyte proliferation and cytokine- and immunoglobulin production.
Since leflunomide and hydroxychloroquine combination therapy in vitro potently inhibits several immune responses that are key to immunopathology in pSS this drug combination holds promise for treatment of pSS.
This box summarizes key points contained in the article.
Declaration of interest
The authors have no relevant affiliations or financial involvement with any organization or entity with a financial interest in or financial conflict with the subject matter or materials discussed in the manuscript. This includes employment, consultancies, honoraria, stock ownership or options, expert testimony, grants or patents received or pending, or royalties.
Reviewer disclosures
Peer reviewers on this manuscript have no relevant financial or other relationships to disclose.
Supplemental Material
Download TIFF Image (47.5 KB)Supplementary material
Supplemental data for this article can be accessed here.
Additional information
Funding
References
- Nocturne G, Cornec D, Seror R, et al. Use of biologics in Sjögren’s syndrome. Rheum Dis Clin North Am. 2016;42:407–417.
- Mavragani CP, Moutsopoulos HM. Sjögren’s syndrome. Annu Rev Pathol Mech Dis. 2014;9:273–285.
- Bombardieri M, Lewis M, Pitzalis C. Ectopic lymphoid neogenesis in rheumatic autoimmune diseases. Nat Rev Rheumatol. 2017;13:141–154.
- Barone F, Bombardieri M, Manzo A, et al. Association of CXCL13 and CCL21 expression with the progressive organization of lymphoid-like structures in Sjögren’s syndrome. Arthritis Rheum. 2005;52:1773–1784.
- Theander E, Vasaitis L, Baecklund E, et al. Lymphoid organisation in labial salivary gland biopsies is a possible predictor for the development of malignant lymphoma in primary Sjogren’s syndrome. Ann Rheum Dis. 2011;70:1363–1368.
- Risselada AP, Kruize AA, Goldschmeding R, et al. The prognostic value of routinely performed minor salivary gland assessments in primary Sjögren’s syndrome. Ann Rheum Dis. 2014;73:1537–1540.
- Risselada AP, de Hair M, Kruize AA, et al. Lymphocytic focus score as a prognostic tool. Ann Rheum Dis. 2015;74:e31.
- Gottenberg J-E, Cagnard N, Lucchesi C, et al. Activation of IFN pathways and plasmacytoid dendritic cell recruitment in target organs of primary Sjögren’s syndrome. Pnas. 2006;103:2770–2775.
- Båve U, Nordmark G, Lövgren T, et al. Activation of the type I interferon system in primary Sjögren’s syndrome: A possible etiopathogenic mechanism. Arthritis Rheum. 2005;52:1185–1195.
- Hjelmervik TOR, Petersen K, Jonassen I, et al. Gene expression profiling of minor salivary glands clearly distinguishes primary Sjögren’s syndrome patients from healthy control subjects. Arthritis Rheum. 2005;52:1534–1544.
- Nordmark G. Mechanisms of disease: primary Sjögren’s syndrome and the type I interferon system. Nat Clin Pract Rheumatol. 2006;2:262–269.
- Brkic Z, Maria NI, Van Helden-Meeuwsen CG, et al. Prevalence of interferon type I signature in CD14 monocytes of patients with Sjögren’s syndrome and association with disease activity and BAFF gene expression. Ann Rheum Dis. 2013;72:728–735.
- Nezos A, Gravani F, Tassidou A, et al. Type I and II interferon signatures in Sjogren’s syndrome pathogenesis: contributions in distinct clinical phenotypes and Sjogren’s related lymphomagenesis. J Autoimmun. 2015;63:47–58.
- Woerkom JM, Van, Kruize AA, Wijk MJGW, et al. Salivary gland and peripheral blood T helper 1 and 2 cell activity in Sjögren’s sicca syndrome. Ann Rheum Dis. 2005;64:1474–1479.
- Nakayamada S, Poholek A, Lu K, et al. Type I interferon induces binding of STAT1 to Bcl6: divergent roles of STAT-family transcription factors in the Tfh cell genetic program. J Immunol. 2014;192:2156–2166.
- Domeier PP, Chodisetti SB, Soni C, et al. IFN- γ receptor and STAT1 signaling in B cells are central to spontaneous germinal center formation and autoimmunity. J Exp Med. 2016;213:715–732.
- Velu V, Mylvaganam GH, Hong JJ, et al. Induction of Th1-biased T follicular helper (Tfh) cells in lymphoid tissues during chronic simian immunodeficiency virus infection defines functionally distinct germinal center Tfh cells. J Immunol. 2016;197:1832–1842.
- Yu S, Jia L, Zhang Y, et al. IL-12 induced the generation of IL-21- and IFN- g -co-expressing poly-functional CD4+ T cells from human naive CD4+ T cells. Cell Cycle. 2015;14:3362–3372.
- Risselada AP, Looije MF, Kruize AA, et al. The role of ectopic germinal centers in the immunopathology of primary Sjögren’s syndrome: a systematic review. Semin Arthritis Rheum. 2013;42:368–376.
- Kruize A, Hene R, Kallenberg C, et al. Hydroxychloroquine treatment for primary Sjögren’s syndrome : a two year double blind cross over trial. Ann Rheum Dis. 1993;52:360–364.
- Van Woerkom JM, Kruize AA, Geenen R, et al. Safety and efficacy of leflunomide in primary Sjögren’s syndrome: a phase II pilot study. Ann Rheum Dis. 2007;66:1026–1032.
- Gottenberg J-E, Ravaud P, Puéchal X, et al. Effects of hydroxychloroquine on symptomatic improvement in primary Sjögren syndrome the JOQUER randomized clinical trial. Jama. 2014;312:249–258.
- Bruneau J-M, Yea CM, Spinella-Jaegle S, et al. Purification of human dihydro-orotate dehydrogenase and its inhibition by A77 1726, the active metabolite of leflunomide. Biochem J. 1998;336:299–303.
- Siemasko K, Chong A, Williams J, et al. Regulation of B cell function by the immunosuppressive agent leflunomide. Transplantation. 1996;61:635–642.
- Abarientos C, Sperber K, Shapiro DL, et al. Hydroxychloroquine in systemic lupus erythematosus and rheumatoid arthritis and its safety in pregnancy. Expert Opin Drug Saf. 2011;10:705–714.
- Lau CM, Broughton C, Tabor AS, et al. RNA-associated autoantigens activate B cells by combined B cell antigen receptor/Toll-like receptor 7 engagement. J Exp Med. 2005;202:1171–1177.
- Wallace D, Gudsoorkar V, Weisman S, et al. New insights into mechanisms of therapeutic effects of antimalarial agents in SLE. Nat Rev Rheumatol. 2012;8:522–533.
- Kužnik A, Benčina M, Švajger U, et al. Mechanism of endosomal TLR inhibition by antimalarial drugs and imidazoquinolines. J Immunol. 2011;186:4794–4804.
- Pasquier B. Autophagy inhibitors. Cell Mol Life Sci. 2016;73:985–1001.
- Vitali C, Bombardieri S, Jonsson R, et al. Classification criteria for Sjögren’s syndrome: a revised version of the European criteria proposed by the American-European Consensus Group. Ann Rheum Dis. 2002;61:554–558.
- Jager WD, Velthuis H, Prakken BJ, et al. Simultaneous detection of 15 human cytokines in a single sample of stimulated peripheral blood mononuclear cells simultaneous detection of 15 human cytokines in a single sample of stimulated peripheral blood mononuclear cells. Clin Diagn Lab Immunol. 2003;10:133–139.
- van Roon E, Jansen T, van de Laar M, et al. Therapeutic drug monitoring of A77 1726, the active metabolite of leflunomide: serum concentrations predict response to treatment in patients with rheumatoid arthritis. Ann Rheum Dis. 2005;64:569–574.
- Mok CC. Therapeutic monitoring of the immuno-modulating drugs in systemic lupus erythematosus. Expert Rev Clin Immunol. 2016;13:1–7.
- Costedoat-Chalumeau N, Guern le V, Piette JC. Routine hydroxychloroquine blood concentration measurement in systemic lupus erythematosus reaches adulthood. J Rheumatol. 2015;42:1997–1999.
- Devauchelle-Pensec V, Mariette X, Jousse-Joulin S, et al. Treatment of primary Sjögren syndrome with rituximab: a randomized trial. Ann Intern Med. 2014;160:233–242.
- Meiners PM, Vissink A, Kroese GM, et al. Abatacept treatment reduces disease activity in early primary Sjögren’s syndrome (open-label proof of concept ASAP study). Ann Rheum Dis. 2014;73:1–4.
- Adler S, Körner M, Förger F, et al. Evaluation of histologic, serologic, and clinical changes in response to abatacept treatment of primary sjögren’s syndrome: A pilot study. Arthritis Care Res (Hoboken). 2013;65:1862–1868.
- Mariette X, Seror R, Quartuccio L, et al. Efficacy and safety of belimumab in primary Sjögren’s syndrome: results of the BELISS open-label phase II study. Ann Rheum Dis. 2013;74:1–6.
- van der Heijden EHM, Kruize AA, Radstake TRDJ, et al. Optimizing conventional DMARD therapy for Sjögren’s syndrome. Autoimmun Rev. 2018;17:480–492.
- Carubbi. Efficacy and safety of rituximab treatment in early primary Sjögren’s syndrome: a prospective, multi-center, follow-up study. Arthritis Res Ther. 2013;15:R172.
- Alunno A, Carubbi F, Bistoni O, et al. Interleukin (IL)-17-producing pathogenic T lymphocytes co-express CD20 and are depleted by rituximab in primary Sjögren’s syndrome: a pilot study. Clin Exp Rheumatol. 2016;184:284–292.
- Abdulahad WH, Meijer JM, Kroese FGM, et al. B cell reconstitution and T helper cell balance after rituximab treatment of active primary Sjögren’s syndrome. Arthritis Rheum. 2011;63:1116–1123.
- Verstappen GM, Kroese FGM, Meiners PM, et al. B cell depletion therapy normalizes circulating follicular th cells in primary Sjögren syndrome. J Rheumatol. 2017;44:49–58.
- Hamza N, Bootsma H, Yuvaraj S, et al. Persistence of immunoglobulin-producing cells in parotid salivary glands of patients with primary Sjögren’s syndrome after B cell depletion therapy. Ann Rheum Dis. 2012;71:1881–1887.
- St Clair E, Levesque M, Prak E, et al. Rituximab therapy for primary Sjögren’s syndrome: an open-label clinical trial and mechanistic analysis. Arthritis Rheum. 2013;65:1097–1106.
- Radstake T, van der Heijden E, Moret F, et al. Clinical efficacy of leflunomide/hydroxychloroquine combination therapy in patients with primary Sjogren’s syndrome: results of a placebo-controlled double-blind randomized clinical trial [abstract]. Arthritis Rheumatol. 2018;70(suppl 10).