ABSTRACT
Introduction: De novo donor-specific antibodies (dnDSA) directed against HLA are a major contributing factor to the chronic deterioration of renal allograft function. Several factors, including the degree of HLA matching, younger recipient age, and past sensitization events have been shown to increase the risk for the development of dnDSA. The development of dnDSA is also strongly associated with modifications in the immunosuppressive regimen, non-adherence, and under-immunosuppression.
Areas covered: Tacrolimus is widely used after solid organ transplantation (SOT) and in recent years, both a high intra-patient variability in tacrolimus exposure and low tacrolimus exposure have been found to be associated with a higher risk of dnDSA development in kidney transplant recipients. This article provides an overview of current findings published in the recent 5 years regarding the relationship between tacrolimus exposure and variation therein and the development of dnDSA.
Expert opinion: In this review, we describe how combining data on tacrolimus intra-patient variability and mean pre-dose concentration may be an effective tool to identify kidney transplant recipients who are at higher risk of developing dnDSA.
1. Introduction
Renal transplantation is the preferred treatment for patients with end-stage renal disease, generally leading to improved quality of life and longer survival [Citation1,Citation2]. Despite advances in the field of transplantation, long-term renal allograft loss remains a significant problem. Early acute rejection rates have been reduced to less than 15% and 1-year graft survival rates are over 90% [Citation1,Citation3]. However, in the long term, there is a gradual decline of graft survival rates. Recently, Coemans et al. published 1- and 5-year renal graft survival rates of patients transplanted in Europe between 2006 and 2015 of 92.0% and 84.4%, respectively [Citation4]. The introduction of more potent immunosuppressive drugs such as cyclosporine A (CsA) in the 1980s and mycophenolate mofetil (MPA) and tacrolimus in the 1990s may explain the significant decrease in acute rejection rates and the improvement of short-term allograft survival.
Long-term renal allograft survival is significantly affected by a large number of variables including donor and recipient age, donor and recipient ethnicity, recipient gender, duration of dialysis treatment, cold ischemia time, comorbidities, human leukocyte antigen (HLA) mismatches, and sensitization against HLA antigens [Citation5,Citation6]. One of the most challenging complications following kidney transplantation is antibody-mediated rejection (ABMR) [Citation7–Citation9]. Sellares et al. 2012 found ABMR to be the most common cause of late kidney allograft failure in their patient cohort, with non-adherence being present in almost half of the patients who experienced rejection-related failures [Citation10]. De novo donor-specific antibodies (dnDSA) directed against HLA are highly associated with the development of ABMR [Citation2,Citation11–Citation14]. Although up to 45% of the patients with DSA do not develop ABMR [Citation15], there is strong evidence indicating that DSAs and particularly dnDSA are associated with worse long-term outcomes and higher rates of allograft loss [Citation13,Citation16]. Besides allograft failure, the development of dnDSA results in an increased challenge to find an acceptably matched kidney in patients who require a re-transplantation.
In the past, chronic allograft dysfunction was commonly attributed to chronic calcineurin inhibitor (CNI)-mediated nephrotoxicity while much of the pathological effects of dnDSA and the process of antibody-mediated rejection (ABMR) went unrecognized. Nowadays, more sensitive techniques for measuring anti-HLA antibodies and a wider classification of ABMR subtypes have increased our understanding of chronic deterioration of renal allograft function [Citation2,Citation17].
Research into the clinical impact of dnDSA on allograft function has identified a number of variables that are associated with the development of dnDSA. These include the degree of HLA matching, younger recipient age, African American ethnicity, the preexistence of non-DSA anti-HLA antibodies at the time of transplantation and past sensitization events. Several studies have shown that the incidence of dnDSA is strongly associated with modifications in the immunosuppressive regimen, non-adherence, and under-immunosuppression [Citation6,Citation18].
CNI-based immunosuppression is the most commonly used therapeutic regimen in SOT, with tacrolimus being recommended as the first-choice CNI [Citation19]. Tacrolimus is a critical dose drug and therapeutic drug monitoring (TDM) is routinely performed to avoid both under- and overexposure [Citation20–Citation22]. Tacrolimus has a high inter-patient variability in its pharmacokinetics. Tacrolimus also has a high intra-patient variability (IPV), which means that despite the tacrolimus dose remaining unchanged, concentrations can vary greatly within an individual over time, which may result in episodes of sub-therapeutic or supra-therapeutic exposure. While environmental factors [Citation23] and genetic variability [Citation24,Citation25] can influence tacrolimus IPV, non-adherence has been presented as the most common cause for a high IPV [Citation26,Citation27]. In 2010, Borra et al. showed that a high tacrolimus IPV was associated with poor outcome after kidney transplantation [Citation28]. Using a better statistical framework, Sapir Pichhadze et al. also demonstrated that a higher standard deviation in tacrolimus levels resulted in a higher hazard ratio for a composite endpoint that included late allograft rejection, transplant glomerulopathy or total graft loss [Citation29]. Several other studies have confirmed that a high IPV is associated with poor long-term outcomes after kidney transplantation, late allograft rejection, and reduced renal allograft survival [Citation30–Citation33]. In a recent study by Sablik et al. it was shown that a high tacrolimus IPV was not associated with the incidence of chronic active ABMR. However, they did show that a high tacrolimus IPV was associated with an inferior graft survival in patients with chronic active ABMR with a high IPV versus those with a low IPV [Citation34]. In order to further improve long-term outcomes after transplantation, it is important to gain more insight into the role of tacrolimus underexposure and IPV in the development of dnDSA. The aim of this review is to describe the current knowledge on the association between tacrolimus exposure and variability therein and the development of dnDSA after renal transplantation.
2. Cellular mechanism of action of tacrolimus in dnDSA developments
Long-lived antibody-producing B cells can be formed through T cell-dependent antigen presentation in B cell follicles, known as germinal centers (GC), in secondary lymphoid organs [Citation35,Citation36]. Follicular T helper (Tfh) cells are a CD4+ T cell subset. Tfh cells can migrate to the GC [Citation37,Citation38] where they can provide help to naïve B cells in the process of differentiating into antibody-producing plasma cells and high-affinity memory B cells [Citation39–Citation41]. The GC response is regulated by follicular regulatory T (Tfr) cells which are a subset of regulatory T cells (Tregs) [Citation42,Citation43]. Tfr cells share markers with both Tregs and Tfh cells [Citation44,Citation45] and recent studies suggest that they might perform their suppressive function not only inside the GC but also from the outside in [Citation46]. Both pre-transplant and dnDSA of the IgG class are strongly associated with acute and chronic allograft injury which suggests that GC reactions are crucial in the pathophysiology of ABMR in kidney transplant patients [Citation47,Citation48].
Tacrolimus performs its immunosuppressive function by binding and inhibiting calcineurin, which results in a blockade of dephosphorylation of the nuclear factor of activated T cells (NFAT) [Citation49]. This blockade of NFAT prevents it from translocating into the DNA promoter region in the nucleus, thereby inhibiting the transcription of the inflammatory cytokine interleukin-2 (IL-2) [Citation50]. Ultimately, this leads to abolished T cell development and proliferation [Citation51]. In a previous study from our group, we showed that the IL-21-producing capacity of circulating (c)Tfh cells decreased in kidney transplant recipients receiving tacrolimus-based immunosuppression [Citation52]. Despite their reduced IL-21 production, cTfh cells still had the capacity to induce B cell differentiation into IgM and IgG-producing plasmablasts in vitro [Citation41,Citation52]. Recently, Wallin et al. compared the effect of tacrolimus treatment in paired blood and lymph node samples obtained from transplant recipients. Living-donor kidney transplant recipients were treated with tacrolimus for a week prior to transplantation while the deceased-donor recipients received no pre-transplantation tacrolimus treatment [Citation53]. One week of tacrolimus treatment was sufficient to reduce the frequency of both circulating and lymph node Tfh cells in the transplant recipients. At the same time, both Treg and Tfr numbers and activation remained the same in the tacrolimus treated and untreated recipients. Furthermore, they found that tacrolimus prevents B cell maturation and antibody production in B cell/Tfh co-cultures. Similarly, Iwasaki et al. performed a series of co-cultures with CD45RA−CXCR5+ cTfh and CD19 + B cells where they showed that tacrolimus is able to suppress B cell activation in a dose-dependent manner [Citation54]. Together these data suggest that optimization of tacrolimus immunosuppression might be an important factor in the prevention of Tfh-mediated dnDSA development. Heidt et al. demonstrated that tacrolimus is able to inhibit IgG and IgM production when B cells were cultured with non-pre-activated T cells [Citation55]. However, tacrolimus failed to inhibit immunoglobulin production when B cells were cultured with pre-activated T cells. Pre-activated T cells were stimulated with anti-CD3 monoclonal antibody (mAb)/anti-CD28 mAb and IL-2 for 2 days prior to co-culturing with B cells. Their findings suggest that tacrolimus is capable of suppressing T cell-dependent humoral immune responses by interfering with T cell function. De Bruyne et al. showed that tacrolimus treatment resulted in decreased cell division and plasmablast differentiation in naïve B cells treated with human recombinant transforming growth factor (TGF)-b1 [Citation56]. Additionally, tacrolimus was able to suppress IgA class-switching and IgA secretion in naïve B cells in a dose-dependent manner [Citation56]. While tacrolimus seems to exert an immunosuppressive effect on naïve B cells, the deleterious effects of dnDSA result from the presence of bone marrow-derived class-switched IgG secreting B cells [Citation40,Citation57]. Currently, there is little evidence to suggest that tacrolimus might have a direct effect on the proliferation and function of bone marrow-derived plasma cells and memory B cells [Citation53,Citation58,Citation59]. In contrast, in vitro treatment with MPA and sirolimus result in inhibition of B cell proliferation and immunoglobulin production [Citation58,Citation60].
3. De novo DSA development in kidney transplant recipients on tacrolimus-based immunosuppression regimens
In recent years, several studies have provided evidence that low tacrolimus exposure and/or high tacrolimus IPV can lead to dnDSA formation in renal transplant patients (). The IPV is generally assessed by the coefficient of variation (CV) which is calculated by dividing the standard deviation (SD) of a number of serial pre-dose concentrations by the mean of these tacrolimus measurements [Citation23]. The tacrolimus pre-dose concentrations may be dose corrected or not. And while some authors only report the SD others use the mean absolute deviation to describe IPV (). For a comprehensive overview of methods to determine tacrolimus IPV see Shuker et al. [Citation23]. The time in the therapeutic range calculates the percentage of time a patient is within a predefined drug target range. The time in therapeutic range is commonly used as a quality measure for anticoagulation therapy with vitamin-K antagonists, but this parameter can also be used to calculate the proportion of time during which the tacrolimus concentrations are on target [Citation61].
Figure 1. Tacrolimus intra-patient variability. An example showing the difference between non-dose corrected (A) and dose-corrected (B).
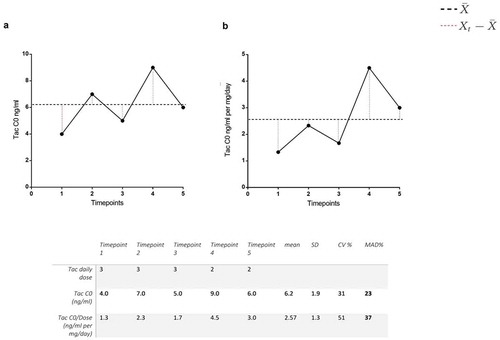
Table 1. Studies in kidney transplantation correlating low tacrolimus exposure to de novo donor-specific antibody development.
Pizzo et al. studied 37 biopsies from 23 pediatric renal transplant patients on combined sirolimus and low-dose tacrolimus immunosuppression [Citation62]. A high IPV of tacrolimus was associated with the formation of dnDSAs, graft rejection, and medication non-adherence. Of the biopsies with both high sirolimus and tacrolimus CV, no less than 67% developed dnDSA.
Rodrigo et al. analyzed dnDSA formation at 1, 3, 5 years and 6 months prior to the last follow-up visit or graft loss in 310 adult renal transplant patients [Citation63]. Formation of dnDSA was also correlated to tacrolimus IPV in their patient cohort. A total of 12.6% of the patients developed dnDSA. A high CV % was found to be one of the few independent risk factors for the development of dnDSA. At a CV cutoff of 30%, the odds ratio for developing dnDSA was 2.48. Additionally, they found that a high IPV was a predictor of death censored graft loss.
Gatault et al. studied the efficacy and safety of two different target concentrations of extended-release tacrolimus (TacER) in 186 low-immunologic risk adult renal transplant patients [Citation64]. Patients were randomized to a 50% reduction in the daily dose of TacER (targeted C0 > 3 ng/mL) or continuation of the prerandomization dose (targeted C0 7–12 ng/mL). The low-dose tacrolimus patients experienced more biopsy-proven rejections and their TCMR grade was more severe. Furthermore, 6/87 patients in the low-dose tacrolimus group developed dnDSAs in contrast to 0/99 of the patients in the regular dose tacrolimus patient group.
Wiebe et al. determined the class II HLA-DR and -DQ eplet mismatches of 596 patients and studied the relationship between tacrolimus exposure and the development of dnDSA in these patients [Citation65]. An eplet is a portion of the epitope that is based on the amino acid mismatching between donor and recipient [Citation66]. This type of mismatching is becoming increasingly popular in SOT in view of the growing knowledge on epitope risk stratification and antibody cross-reactivity [Citation67–Citation69]. Patients with a significantly higher tacrolimus CV were more likely to develop HLA-DR/DQ dnDSA than patients with a lower tacrolimus CV (39.6 ± 13.5 versus 33.7 ± 13.3). Additionally, the lowest recorded tacrolimus pre-dose concentration (ng/mL) in patients who developed dnDSA was significantly lower than in patients who did not develop dnDSA (2.76 ± 1.71 versus 3.42 ± 1.45). Recipients with a high immunological risk, defined as having >11 HLA-DR or DQ eplet mismatches, who developed dnDSA had significantly higher percentages of tacrolimus pre-dose levels under 5 ng/mL. For recipients with a low immunological risk, defined as having <11 HLA-DR or DQ eplet mismatches, an increased risk of developing dnDSA could not be shown for tacrolimus pre-dose concentrations below 5 ng/mL. In this study, after correcting for HLA DR/DQ eplet mismatches, the lowest recorded tacrolimus pre-dose concentration was correlated with developing dnDSA at an odds ratio of 0.71 per 1 ng/mL. A higher tacrolimus CV was also correlated with developing dnDSA after correcting for HLA-DR/DQ eplet mismatch (HR 1.34).
Davis et al. evaluated the mean tacrolimus pre-dose concentration and tacrolimus time in therapeutic range in 538 kidney transplant patients in the first year after transplantation for the risk of developing dnDSAs [Citation61]. A mean tacrolimus pre-dose concentration <8 ng/mL within 6 months significantly increased the risk of developing dnDSAs at 6 months (OR 2.51). Similarly, the odds ratio of developing dnDSA by 12 months was 2.32 with a mean tacrolimus pre-dose concentration <8 ng/mL within 12 months. Additionally, tacrolimus time in therapeutic range <60% by 12 months, using a range of 5–10 ng/mL, was also strongly associated with dnDSA development by 12 months (OR 2.05).
Jung et al. prospectively screened 167 adult kidney transplant patients for dnDSA and correlated these findings to tacrolimus pre-dose concentration and ABMR [Citation70]. A total of 16 patients developed dnDSA. Lower tacrolimus levels in the first year after transplantation were associated with the development of dnDSAs. More specifically, tacrolimus pre-dose levels <7 ng/mL in the first 0–2 months after transplantation were a significant predictor of dnDSA development (HR 0.70). In contrast to other studies, no association was found between tacrolimus levels measured 2 or more months after transplantation and the development of dnDSA.
Girerd et al. assessed the impact of reduced exposure to either CsA or tacrolimus on the development of dnDSA in 247 low-immunological risk patients [Citation71]. Reduced exposure to CNI was defined as a pre-dose concentration below the lower limit of the drug targets for ≥50% of time intervals. Patients with reduced exposure to CNI had an increased risk of developing dnDSA (HR 9.77).
More recently, Kaya Aksoy et al. described that a tacrolimus CV cutoff value of 32% was most accurate for identifying dnDSA development in their patient cohort of 67 pediatric kidney transplant recipients (AUC 0.713) [Citation72]. De novo DSA development during the course of the follow- up period was associated with a higher percentage of patients with a tacrolimus CV >32% between 6 and 12 months and over 1 year after transplantation (67% vs 31% and 83% vs 47%, respectively).
Information about patients who have already developed dnDSA and the effect of tacrolimus pre-dose levels and tacrolimus variability on graft outcomes is limited. Beland et al. found that higher tacrolimus levels in patients with dnDSA were associated with better kidney graft survival. In 42 unsensitized patients who later developed dnDSA during their screening period, a positive association was found between higher mean tacrolimus concentrations and lower risk of graft loss (HR, 0.49; 95% CI, 0.33–0.75; p = 0.001) [Citation73]. This effect persisted after adjusting for known predictors of graft loss such as delayed graft function, non-adherence, transplant glomerulopathy, interstitial fibrosis, tubular atrophy, and arteriolar hyaline thickening scores. A mean tacrolimus level below 5.3 ng/mL in the first 2 years post-dnDSA detection was a strong, independent predictor of graft loss.
Shuker et al. found that an increase in tacrolimus IPV in combination with decreased tacrolimus pre-dose concentrations at 12 months was associated with an increased hazard of a composite endpoint consisting of biopsy-proven graft rejection, doubling of serum creatinine concentrations and graft loss [Citation30]. Similarly, Rozen-Zvi et al. have previously combined the time-weighted variability of tacrolimus with high or low drug levels to identify patients at risk for graft loss [Citation74]. In their study, patients with a combination of high tacrolimus time-weighed variability and low drug level exposure had a lower graft survival when compared to patients with all other tacrolimus variability and exposure combinations (HR 3.14; p < 0.001). Graft function and graft rejection have also been shown to be associated with the tacrolimus concentration-to-dose (C/D) ratio [Citation75,Citation76]. Recently, Jouve et al. found that time-dependent tacrolimus C/D ratio and early tacrolimus C/D ratio predict kidney graft survival even after correcting for other predictors including tacrolimus pre-dose levels and tacrolimus IPV [Citation77]. Possibly, combining different tacrolimus monitoring strategies can more accurately identify kidney transplant recipients at risk for developing dnDSA.
4. De novo DSA development in liver transplant recipients on tacrolimus-based immunosuppression regimens
Although the focus of this paper is on kidney transplant, it is fair to mention that also in patients transplanted with other organs, associations between dnDSA development and tacrolimus exposure have been reported. Kaneku et al. analyzed factors associated with dnDSA formation in 749 liver transplant patients and they found that patients with low CNI pre-dose concentrations (tacrolimus <3 ng/mL or CsA <75 ng/mL) were at increased risk of developing dnDSA (OR 2.66; 95% CI, 1.2–5.84; p = 0.015) [Citation78]. More recently, Del Bello et al. described that at a cutoff CV of >35% the odds ratio for developing dnDSA within the first 2 years post-transplantation was 4.83 (p = 0.01) and at a cutoff CV of >40% the odds ratio increased to 9.73 (p = 0.01) [Citation79]. However, dnDSA and a high tacrolimus IPV seem to have a limited overall impact on graft and patient outcome after liver transplantation [Citation80,Citation81].
5. Co-medication
CNI-based immunosuppression has been shown to be more protective against dnDSA formation in comparison to mammalian target of rapamycin-based immunosuppression in both kidney and liver transplant patients [Citation82]. Studies comparing everolimus to the CNI inhibitor CsA found that recipients receiving everolimus were at higher risk of acute rejection and had a higher incidence of dnDSA development [Citation83–Citation86]. Conversely, a study comparing CNI-free everolimus regimen to CNI-based (CsA or tacrolimus) regimen found no difference in the occurrence of dnDSA at last follow up [Citation87]. Nonetheless, not all CNI inhibitors seem to be equally efficient at preventing the formation of dnDSA [Citation78,Citation88]. Wiebe et al. reported a 2.7 fold higher incidence of dnDSA development in recipients treated with CsA versus those treated with tacrolimus [Citation65]. Despite these findings, dnDSA is still commonly found in SOT patients treated with standard-dose tacrolimus [Citation61,Citation63,Citation78,Citation79,Citation82]. It is likely that differences in induction therapy and overall immunosuppression regimen contribute to the individual and/or patient cohort risk profile for developing dnDSA.
Jung et al. found that tacrolimus levels <7 ng/mL between 2 and 12 months and a high tacrolimus IPV between 0 and 12 months were not associated to the development of dnDSA while other studies found a positive association with similar tacrolimus pre-dose concentrations and high tacrolimus IPV [Citation61,Citation63–Citation65,Citation70]. One possible explanation for this difference in outcome could be the difference in the induction therapy regimen in these patient cohorts. All recipients in the study by Jung et al. received induction therapy in the form of an IL-2 receptor blocker [Citation70]. In contrast, the patient cohorts in the studies by Rodrigo et al., Davis et al. and Wiebe et al. received anti-thymocyte globulin or IL-2 blocker at lower percentages ranging from 28% to 56% [Citation61,Citation63,Citation65].
Steroid-free maintenance therapy may also be a risk factor for the development of dnDSA. Pizzo et al. found that a high tacrolimus IPV (CV >31%) was weakly correlated with a high sirolimus IPV (CV >25%) [Citation62], suggesting that the deleterious effects of under-immunosuppression due to a high tacrolimus IPV might be offset by other immunosuppressive medication. This may be especially relevant in the case of patients treated with standard triple immunosuppression therapy with high levels of prednisone and/or MPA. Recently, Shuker et al. found that a high tacrolimus IPV was not associated with the incidence and progression of cardiac allograft vasculopathy in heart transplant recipients [Citation89]. The authors hypothesized that the effect of tacrolimus IPV on heart allograft outcomes may be considerably less than on kidney allograft outcomes due to the continuous use of prednisone and a higher number of immunosuppressive drugs after heart transplantation [Citation89]. In 2019, the results from the TRANSFORM study demonstrated that the use of reduced tacrolimus + everolimus versus tacrolimus + MPA resulted in comparable two-year outcomes and dnDSA incidence (22% versus 18%, respectively) [Citation90]. So, while reduced tacrolimus in combination with MPA seems to be associated with higher dnDSA development [Citation64], it seems that everolimus is better able to protect against the deleterious effects of a lower tacrolimus exposure. Based on these studies, we think that the type of induction therapy, co-medication, and overall immunosuppressive load may need to be considered when assessing the risk of tacrolimus underexposure in the development of dnDSA.
6. Conclusion
In this review, we discuss several studies that have investigated the relationship between tacrolimus exposure and the development of dnDSA in patients with kidney transplantations. Most of these studies found an increased risk of dnDSA development in patients with a high tacrolimus IPV [Citation62,Citation63,Citation65], lower tacrolimus exposure (as assessed by mean pre-dose concentrations) [Citation61,Citation64,Citation65,Citation70,Citation71] and a shorter time in therapeutic range [Citation61]. This association was found for tacrolimus exposure in the first months after kidney transplantation and up to the time point of dnDSA detection. Wiebe et al. found that mean tacrolimus pre-dose concentrations in the 6 months leading up to dnDSA development were significantly lower in comparison to mean tacrolimus pre-dose concentration in these same patients at earlier time points [Citation65]. It is likely that prolonged periods of tacrolimus underexposure at any time point after transplantation can lead to dnDSA formation [Citation91,Citation92]. This may be relevant in patients who have initially been adherent to their medication and become non-adherent several years after transplantation. To our knowledge, only one study has reported a negative association between a high tacrolimus IPV and the development of dnDSA [Citation93]. However, we cannot exclude the possibility of a publication bias toward studies that have found positive results.
In summary, underexposure to tacrolimus is associated with the development of dnDSA and increased graft loss. The underexposure can be demonstrated by calculating mean tacrolimus concentrations over a period of time, by time-in-therapeutic range and by calculating IPV. Probably, in a substantial proportion of patients non-adherence contributes to the low tacrolimus levels and/or variability, but evidence for this association is weak at best.
7. Expert opinion
There is no consensus on the best target tacrolimus therapeutic range to prevent the development of dnDSA and the optimal tacrolimus C0 is likely to be different based on the induction therapy used or co-medication regimen. It is also unknown whether a higher tacrolimus therapeutic range might be able to attenuate ongoing immunological damage in patients who already have formed dnDSA. Beland et al. found that higher tacrolimus levels in patients with dnDSA resulted in improved kidney graft survival [Citation73]. Furthermore, Sablik et al. showed that while a high tacrolimus IPV was not associated with the incidence of chronic active ABMR, it was associated to inferior graft survival in patients with chronic active ABMR [Citation34]. Together these findings suggest that patients might benefit from higher immunosuppressive maintenance therapy following the development of dnDSA and that a high tacrolimus IPV is particularly dangerous in patients with ABMR. It remains unclear whether the benefit of longer graft survival outweighs the risks of higher tacrolimus exposure.
While tacrolimus IPV may be a valuable tool for identifying patient non-adherence, it cannot discriminate between periods of under-immunosuppression or over-immunosuppression. The adverse outcomes associated with a high tacrolimus IPV may, on the one hand, be related to immune activation leading to rejection episodes and, on the other hand, to the effects of CNI toxicity, cancer, and infections [Citation29]. The same can be said for tacrolimus monitoring by time in the therapeutic range. The tacrolimus C/D ratio may serve to identify patients who are genetically predisposed to more rapid fluctuations in tacrolimus blood levels. In contrast to tacrolimus IPV and time in therapeutic range, the C/D ratio is less a reflection of possible medication non-adherence. Ideally, we would like to identify patients who are chronically under-immunosuppressed or experience recurrent episodes of sub-therapeutic tacrolimus exposure. Especially patients who have a relatively low mean tacrolimus exposure in combination with high variability may have periods of extremely low immunosuppression leading to induction of alloreactivity. In contrast, in case of a high mean exposure such fluctuations may not put the patients at increased risk. This may also explain why Borra et al. could not find an association between outcome and IPV in a population with high mean mycophenolic acid levels [Citation28]. A similar interplay between tacrolimus C/D ratio and pre-dose concentrations may occur where fast metabolizers who have low mean tacrolimus exposure may be experiencing regular periods of under-immunosuppression. Alternatively, due to the high dose also high tacrolimus peak concentrations may lead to nephrotoxicity and long-term graft dysfunction. The study described by Rozen-Zvi et al. shows that combining tacrolimus IPV with mean pre-dose concentration may be an effective tool to identify patients who are regularly under-immunosuppressed [Citation74]. Despite its nephrotoxic effects, tacrolimus-based immunosuppression is likely to remain the main immunosuppressive regimen for years to come due to its effectiveness in preventing acute rejection episodes. This is all the more reason to incorporate algorithms that calculate tacrolimus IPV and C/D ratio in combination with pre-dose concentrations into electronic patient files as a cheap and noninvasive monitoring strategy which can aid clinicians in the identification of patients who are most at risk for developing dnDSA.
Article highlights
De novo donor-specific antibodies (DSA) directed against HLA are highly associated with the development of antibody-mediated rejection and higher rates of allograft loss in kidney transplant recipients.
The presence of de novo DSA results in an increased challenge to find an acceptably matched kidney in patients who require a re-transplantation.
A high tacrolimus intra-patient variability and overall lower tacrolimus exposure are associated with the development of de novo DSA.
The induction therapy regimen, co-medication, and overall immunosuppressive load need to be considered when assessing the risk of de novo DSA formation in patients with tacrolimus under-exposure.
Combining data on tacrolimus intra-patient variability, C/D ratio and mean pre-dose concentration can potentially become an effective tool to identify transplant recipients who are at higher risk of developing de novo DSA.
Declaration of interest
DA Hesselink has received lecture and consulting fees from Astellas Pharma and Chiesi Farmaceutici SpA, as well as grant support from Astellas Pharma, Chiesi Farmaceutici SpA and Bristol-Myers Squibb. T van Gelder reports study grants from Astellas Pharma and Chiesi Farmaceutici SpA, and honoraria for lectures or consulting from Astellas Pharma and Novartis. The authors have no other relevant affiliations or financial involvement with any organization or entity with a financial interest in or financial conflict with the subject matter or materials discussed in the manuscript apart from those disclosed.
Reviewer disclosures
Peer reviewers on this manuscript have no relevant financial or other relationships to disclose.
Additional information
Funding
References
- Nankivell BJ, Kuypers DR. Diagnosis and prevention of chronic kidney allograft loss. Lancet. 2011 Oct 15;378(9800):1428–1437. PubMed PMID: 22000139.
- Loupy A, Hill GS, Jordan SC. The impact of donor-specific anti-HLA antibodies on late kidney allograft failure. Nat Rev Nephrol. 2012 Apr 17;8(6):348–357. PubMed PMID: 22508180.
- Ojo AO, Morales JM, González-Molina M, et al. Comparison of the long-term outcomes of kidney transplantation: USA versus Spain. Nephrol Dialysis Transplantation. 2012;28(1):213–220.
- Coemans M, Süsal C, Döhler B, et al. Analyses of the short- and long-term graft survival after kidney transplantation in Europe between 1986 and 2015. Kidney Int. 2018;94:964–973.
- Meier-Kriesche HU, Schold JD, Kaplan B. Long-term renal allograft survival: have we made significant progress or is it time to rethink our analytic and therapeutic strategies? Am J Transplant. 2004 Aug;4(8):1289–1295. PubMed PMID: 15268730.
- Neuberger JM, Bechstein WO, Kuypers DR, et al. Practical recommendations for long-term management of modifiable risks in kidney and liver transplant recipients: a Guidance report and clinical checklist by the Consensus On Managing Modifiable Risk In Transplantation (COMMIT) Group. Transplantation. 2017 Apr;101(4S Suppl 2):S1–S56. PubMed PMID: 28328734.
- Djamali A, Kaufman DB, Ellis TM, et al. Diagnosis and management of antibody-mediated rejection: current status and novel approaches. Am J Transplant. 2014 Feb;14(2):255–271. PubMed PMID: 24401076.
- Vo AA, Sinha A, Haas M, et al. Factors predicting risk for antibody-mediated rejection and graft loss in highly human leukocyte antigen sensitized patients transplanted after desensitization. Transplantation. 2015 Jul;99(7):1423–1430. PubMed PMID: 25606792.
- Loupy A, Lefaucheur C. Antibody-mediated rejection of solid-organ allografts. N Engl J Med. 2018 Sep 20;379(12):1150–1160. PubMed PMID: 30231232.
- Sellares J, de Freitas DG, Mengel M, et al. Understanding the causes of kidney transplant failure: the dominant role of antibody-mediated rejection and nonadherence. Am J Transplant. 2012 Feb;12(2):388–399. PubMed PMID: 22081892.
- Loupy A, Suberbielle-Boissel C, Hill GS, et al. Outcome of subclinical antibody-mediated rejection in kidney transplant recipients with preformed donor-specific antibodies. Am J Transplant. 2009 Nov;9(11):2561–2570. PubMed PMID: 19775320.
- Jordan SC, Vo AA. Donor-specific antibodies in allograft recipients: etiology, impact and therapeutic approaches. Curr Opin Organ Transplant. 2014 Dec;19(6):591–597. PubMed PMID: 25304815.
- Butler CL, Valenzuela NM, Thomas KA, et al. Not all antibodies are created equal: factors that influence antibody mediated rejection. J Immunol Res. 2017;2017:7903471. PubMed PMID: 28373996.
- Aubert O, Loupy A, Hidalgo L, et al. Antibody-mediated rejection due to preexisting versus De Novo donor-specific antibodies in kidney allograft recipients. J Am Soc Nephrol. 2017 Jun;28(6):1912–1923. PubMed PMID: 28255002.
- Amico P, Honger G, Mayr M, et al. Clinical relevance of pretransplant donor-specific HLA antibodies detected by single-antigen flow-beads. Transplantation. 2009 Jun 15;87(11):1681–1688. PubMed PMID: 19502960.
- Wiebe C, Gibson IW, Blydt-Hansen TD, et al. Rates and determinants of progression to graft failure in kidney allograft recipients with de novo donor-specific antibody. Am J Transplant. 2015 Nov;15(11):2921–2930. PubMed PMID: 26096305.
- Lionaki S, Panagiotellis K, Iniotaki A, et al. Incidence and clinical significance of de novo donor specific antibodies after kidney transplantation. Clin Dev Immunol. 2013;2013:849835. PubMed PMID: 24348683.
- Monfa E, San Segundo D, San Millan JCR, et al. Intermediate steroid withdrawal after renal transplantation and anti-HLA antibodies (HLA-Abs) development. Nefrologia. 2017 Jul - Aug;37(4):415–422. PubMed PMID: 28330741.
- Kidney Disease: improving Global Outcomes Transplant Work G. KDIGO clinical practice guideline for the care of kidney transplant recipients. Am J Transplant. 2009 Nov;9(Suppl 3):S1–155. PubMed PMID: 19845597.
- Hesselink DA, Bouamar R, Elens L, et al. The role of pharmacogenetics in the disposition of and response to tacrolimus in solid organ transplantation. Clin Pharmacokinet. 2014 Feb;53(2):123–139. PubMed PMID: 24249597.
- Andrews LM, Li Y, De Winter BCM, et al. Pharmacokinetic considerations related to therapeutic drug monitoring of tacrolimus in kidney transplant patients. Expert Opin Drug Metab Toxicol. 2017 Dec;13(12):1225–1236. PubMed PMID: 29084469.
- Brunet M, van Gelder T, Asberg A, et al. Therapeutic drug monitoring of tacrolimus-personalized therapy: second consensus report. Ther Drug Monit. 2019 Jun;41(3):261–307. PubMed PMID: 31045868.
- Shuker N, van Gelder T, Hesselink DA. Intra-patient variability in tacrolimus exposure: causes, consequences for clinical management. Transplantation Rev. 2015 Apr;29(2):78–84. PubMed PMID: 25687818.
- Gijsen VM, Madadi P, Dube MP, et al. Tacrolimus-induced nephrotoxicity and genetic variability: a review. Ann Transplant. 2012 Apr-Jun;17(2):111–121. PubMed PMID: 22743729.
- Seibert SR, Schladt DP, Wu B, et al. Tacrolimus trough and dose intra-patient variability and CYP3A5 genotype: effects on acute rejection and graft failure in European American and African American kidney transplant recipients. Clin Transplant. 2018 Dec;32(12):e13424. PubMed PMID: 30318646.
- Whalen HR, Glen JA, Harkins V, et al. High intrapatient tacrolimus variability is associated with worse outcomes in renal transplantation using a low-dose tacrolimus immunosuppressive regime. Transplantation. 2017 Feb;101(2):430–436. PubMed PMID: 26950724.
- Leino AD, King EC, Jiang W, et al. Assessment of tacrolimus intrapatient variability in stable adherent transplant recipients: establishing baseline values. Am J Transplant. 2018 Dec 1;19(5):1410-1420. PubMed PMID: 30506623.
- Borra LC, Roodnat JI, Kal JA, et al. High within-patient variability in the clearance of tacrolimus is a risk factor for poor long-term outcome after kidney transplantation. Nephrol Dial Transplant. 2010 Aug;25(8):2757–2763. PubMed PMID: 20190242.
- Sapir-Pichhadze R, Wang Y, Famure O, et al. Time-dependent variability in tacrolimus trough blood levels is a risk factor for late kidney transplant failure. Kidney Int. 2014 Jun;85(6):1404–1411. PubMed PMID: 24336032.
- Shuker N, Shuker L, van Rosmalen J, et al. A high intrapatient variability in tacrolimus exposure is associated with poor long-term outcome of kidney transplantation. Transpl Int. 2016 Nov;29(11):1158–1167. PubMed PMID: 27188932.
- van Gelder T. Within-patient variability in immunosuppressive drug exposure as a predictor for poor outcome after transplantation. Kidney Int. 2014 Jun;85(6):1267–1268. PubMed PMID: 24875549.
- O’Regan JA, Canney M, Connaughton DM, et al. Tacrolimus trough-level variability predicts long-term allograft survival following kidney transplantation [journal article]. J Nephrol. 2016 April 01;29(2):269–276.
- Süsal C, Döhler B. Late intra-patient tacrolimus trough level variability as a major problem in kidney transplantation: a collaborative transplant study report. Am J Transplant. 2019;19(10):2805–2813.
- Sablik KA, Clahsen-van Groningen MC, Hesselink DA, et al. Tacrolimus intra-patient variability is not associated with chronic active antibody mediated rejection. PLoS One. 2018;13(5):e0196552. PubMed PMID: 29746495.
- Stebegg M, Kumar SD, Silva-Cayetano A, et al. Regulation of the Germinal Center Response. Front Immunol. 2018;9:2469. PubMed PMID: 30410492.
- Mesin L, Ersching J, Victora GD. Germinal center B cell dynamics. Immunity. 2016 Sep 20;45(3):471–482. PubMed PMID: 27653600.
- Yan L, de Leur K, Hendriks RW, et al. T follicular helper cells as a new target for immunosuppressive therapies. Front Immunol. 2017;8:1510. PubMed PMID: 29163552.
- Baan CC, de Graav GN, Boer K. T follicular helper cells in transplantation: the target to attenuate antibody-mediated allogeneic responses? Curr Transplant Rep. 2014;1(3):166–172. PubMed PMID: 25927019.
- Weinstein JS, Bertino SA, Hernandez SG, et al. B cells in T follicular helper cell development and function: separable roles in delivery of ICOS ligand and antigen. J Immunol. 2014 Apr 1;192(7):3166–3179. PubMed PMID: 24610013.
- Ionescu L, Urschel S. Memory B-cells and long-lived plasma cells. Transplantation. 2019 Feb 4;103:890–898. PubMed PMID: 30747835.
- de Leur K, Dor FJ, Dieterich M, et al. IL-21 receptor antagonist inhibits differentiation of B cells toward plasmablasts upon alloantigen stimulation. Front Immunol. 2017;8:306. PubMed PMID: 28373876.
- Chung Y, Tanaka S, Chu F, et al. Follicular regulatory T cells expressing Foxp3 and Bcl-6 suppress germinal center reactions. Nat Med. 2011 Jul 24;17(8):983–988. PubMed PMID: 21785430.
- Wollenberg I, Agua-Doce A, Hernandez A, et al. Regulation of the germinal center reaction by Foxp3+ follicular regulatory T cells. J Immunol. 2011 Nov 1;187(9):4553–4560. PubMed PMID: 21984700.
- Linterman MA, Pierson W, Lee SK, et al. Foxp3+ follicular regulatory T cells control the germinal center response. Nat Med. 2011 Jul 24;17(8):975–982. PubMed PMID: 21785433.
- Wallin EF. T follicular regulatory cells and antibody responses in transplantation. Transplantation. 2018 Oct;102(10):1614–1623. PubMed PMID: 29757907.
- Sayin I, Radtke AJ, Vella LA, et al. Spatial distribution and function of T follicular regulatory cells in human lymph nodes. J Exp Med. 2018 Jun 4;215(6):1531–1542. PubMed PMID: 29769249.
- Karahan GE, Claas FH, Heidt S. B cell immunity in solid organ transplantation. Front Immunol. 2016;7:686. PubMed PMID: 28119695.
- Chhabra M, Alsughayyir J, Qureshi MS, et al. Germinal center alloantibody responses mediate progression of chronic allograft injury. Front Immunol. 2018;9:3038. PubMed PMID: 30728823.
- Shrestha BM. Two decades of tacrolimus in renal transplant: basic science and clinical evidences. Exp Clin Transplant. 2017 Feb;15(1):1–9. PubMed PMID: 27938316.
- Vafadari R, Kraaijeveld R, Weimar W, et al. Tacrolimus inhibits NF-kappaB activation in peripheral human T cells. PLoS One. 2013;8(4):e60784. PubMed PMID: 23573283.
- Laskin BL, Jiao J, Baluarte HJ, et al. The effects of tacrolimus on T-cell proliferation are short-lived: a pilot analysis of immune function testing. Transplant Direct. 2017;3(8):e199–e199. PubMed PMID: 28795150; eng.
- de Graav GN, Dieterich M, Hesselink DA, et al. Follicular T helper cells and humoral reactivity in kidney transplant patients. Clin Exp Immunol. 2015 May;180(2):329–340. PubMed PMID: 25557528.
- Wallin EF, Hill DL, Linterman MA, et al. The calcineurin inhibitor tacrolimus specifically suppresses human T follicular helper cells. Front Immunol. 2018;9:1184. PubMed PMID: 29904381.
- Iwasaki K, Kitahata N, Hiramitsu T, et al. Increased CD40L+PD-1+ follicular helper T cells (Tfh) as a biomarker for predicting calcineurin inhibitor sensitivity against Tfh-mediated B-cell activation/antibody production after kidney transplantation. Int Immunol. 2018 Jul 24;30(8):345–355. PubMed PMID: 29878122.
- Heidt S, Roelen DL, Eijsink C, et al. Calcineurin inhibitors affect B cell antibody responses indirectly by interfering with T cell help. Clin Exp Immunol. 2010 Feb;159(2):199–207. PubMed PMID: 19922499.
- De Bruyne R, Bogaert D, De Ruyck N, et al. Calcineurin inhibitors dampen humoral immunity by acting directly on naive B cells. Clin Exp Immunol. 2015 Jun;180(3):542–550. PubMed PMID: 25682989.
- Clatworthy MR. B cell modulation in transplantation. Clin Exp Immunol. 2014 Dec;178(Suppl 1):61–63. PubMed PMID: 25546764.
- Heidt S, Roelen DL, Eijsink C, et al. Effects of immunosuppressive drugs on purified human B cells: evidence supporting the use of MMF and rapamycin. Transplantation. 2008 Nov 15;86(9):1292–1300. PubMed PMID: 19005412.
- Thaunat O, Koenig A, Leibler C, et al. Effect of immunosuppressive drugs on humoral allosensitization after kidney transplant. J Am Soc Nephrol. 2016 Jul;27(7):1890–1900. PubMed PMID: 26872489.
- Traitanon O, Mathew JM, La Monica G, et al. Differential effects of tacrolimus versus sirolimus on the proliferation, activation and differentiation of human B cells. PLoS One. 2015;10(6):e0129658. PubMed PMID: 26087255.
- Davis S, Gralla J, Klem P, et al. Lower tacrolimus exposure and time in therapeutic range increase the risk of de novo donor-specific antibodies in the first year of kidney transplantation. Am J Transplant. 2018 Apr;18(4):907–915. PubMed PMID: 28925597.
- Pizzo HP, Ettenger RB, Gjertson DW, et al. Sirolimus and tacrolimus coefficient of variation is associated with rejection, donor-specific antibodies, and nonadherence. Pediatr Nephrol. 2016 Dec;31(12):2345–2352. PubMed PMID: 27286686.
- Rodrigo E, Segundo DS, Fernandez-Fresnedo G, et al. Within-patient variability in tacrolimus blood levels predicts kidney graft loss and donor-specific antibody development. Transplantation. 2016 Nov;100(11):2479–2485. PubMed PMID: 26703349.
- Gatault P, Kamar N, Buchler M, et al. Reduction of extended-release tacrolimus dose in low-immunological-risk kidney transplant recipients increases risk of rejection and appearance of donor-specific antibodies: a randomized study. Am J Transplant. 2017 May;17(5):1370–1379. PubMed PMID: 27862923.
- Wiebe C, Rush DN, Nevins TE, et al. Class II eplet mismatch modulates tacrolimus trough levels required to prevent donor-specific antibody development. J Am Soc Nephrol. 2017;28(11):3353–3362. PubMed PMID: 28729289.
- Tambur AR, Campbell P, Claas FH, et al. Sensitization in transplantation: assessment of risk (STAR) 2017 working group meeting report. Am J Transplant. 2018 Jul;18(7):1604–1614. PubMed PMID: 29603613.
- Montgomery RA, Lonze BE, King KE, et al. Desensitization in HLA-incompatible kidney recipients and survival. N Engl J Med. 2011 Jul 28;365(4):318–326. PubMed PMID: 21793744.
- Kosmoliaptsis V, Mallon DH, Chen Y, et al. Alloantibody responses after renal transplant failure can be better predicted by donor-recipient HLA amino acid sequence and physicochemical disparities than conventional HLA matching. Am J Transplant. 2016 Jul;16(7):2139–2147. PubMed PMID: 26755448.
- Wiebe C, Nickerson P. Strategic use of epitope matching to improve outcomes. Transplantation. 2016 Oct;100(10):2048–2052. PubMed PMID: 27362311.
- Jung H-Y, Kim S-H, Seo M-Y, et al. Characteristics and clinical significance of De Novo donor-specific anti-HLA antibodies after kidney transplantation. J Korean Med Sci. 2018;33(34):e217–e217. PubMed PMID: 30127706.
- Girerd S, Schikowski J, Girerd N, et al. Impact of reduced exposure to calcineurin inhibitors on the development of de novo DSA: a cohort of non-immunized first kidney graft recipients between 2007 and 2014. BMC Nephrol. 2018;19(1): 232–232. PubMed PMID: 30219043.
- Kaya Aksoy G, Comak E, Koyun M, et al. Tacrolimus variability: a cause of donor-specific anti-HLA antibody formation in children. Eur J Drug Metab Pharmacokinet. 2019 Feb 8;44:539–548. PubMed PMID: 30737655.
- Beland MA, Lapointe I, Noel R, et al. Higher calcineurin inhibitor levels predict better kidney graft survival in patients with de novo donor-specific anti-HLA antibodies: a cohort study. Transpl Int. 2017 May;30(5):502–509. PubMed PMID: 28186642.
- Rozen-Zvi B, Schneider S, Lichtenberg S, et al. Association of the combination of time-weighted variability of tacrolimus blood level and exposure to low drug levels with graft survival after kidney transplantation. Nephrol Dial Transplant. 2017 Feb 1;32(2):393–399. PubMed PMID: 28025383.
- Tholking G, Fortmann C, Koch R, et al. The tacrolimus metabolism rate influences renal function after kidney transplantation. PLoS One. 2014;9(10):e111128. PubMed PMID: 25340655.
- Schutte-Nutgen K, Tholking G, Steinke J, et al. Fast Tac metabolizers at risk (-) it is time for a C/D ratio calculation. J Clin Med. 2019 Apr 28;8(5):587. PubMed PMID: 31035422.
- Jouve T, Fonrose X, Noble J, et al. The TOMATO study (TacrOlimus MetabolizAtion in kidney TransplantatiOn): impact of the concentration-dose ratio on death-censored graft survival. Transplantation. 2019 Aug 12:1. PubMed PMID: 31415035.
- Kaneku H, O’Leary JG, Banuelos N, et al. De novo donor-specific HLA antibodies decrease patient and graft survival in liver transplant recipients. Am J Transplant. 2013 Jun;13(6):1541–1548. PubMed PMID: 23721554.
- Del Bello A, Congy-Jolivet N, Danjoux M, et al. High tacrolimus intra-patient variability is associated with graft rejection, and de novo donor-specific antibodies occurrence after liver transplantation. World J Gastroenterol. 2018 Apr 28;24(16):1795–1802. PubMed PMID: 29713132.
- Vandevoorde K, Ducreux S, Bosch A, et al. Prevalence, risk factors, and impact of donor-specific alloantibodies after adult liver transplantation. Liver Transpl. 2018 Aug;24(8):1091–1100. PubMed PMID: 29665189.
- Van Der Veer MAA, Nangrahary N, Hesselink DA, et al. High intra-patient variability in tacrolimus exposure is not associated with immune-mediated graft injury after liver transplantation. Transplantation. 2019 Feb 19;103:2329–2337. PubMed PMID: 30801539.
- O’Leary JG, Samaniego M, Barrio MC, et al. The influence of immunosuppressive agents on the risk of De Novo donor-specific HLA antibody production in solid organ transplant recipients. Transplantation. 2016 Jan;100(1):39–53. PubMed PMID: 26680372.
- Budde K, Zeier M, Witzke O, et al. Everolimus with cyclosporine withdrawal or low-exposure cyclosporine in kidney transplantation from month 3: a multicentre, randomized trial. Nephrol Dial Transplant. 2017 Jun 1;32(6):1060–1070. PubMed PMID: 28605781.
- Liefeldt L, Brakemeier S, Glander P, et al. Donor-specific HLA antibodies in a cohort comparing everolimus with cyclosporine after kidney transplantation. Am J Transplant. 2012 May;12(5):1192–1198. PubMed PMID: 22300538.
- Rostaing L, Hertig A, Albano L, et al. Fibrosis progression according to epithelial-mesenchymal transition profile: a randomized trial of everolimus versus CsA. Am J Transplant. 2015 May;15(5):1303–1312. PubMed PMID: 25808994.
- Huang Y, Ramon D, Luan FL, et al. Incidences of preformed and de novo donor-specific HLA antibodies and their clinicohistological correlates in the early course of kidney transplantation. Clin Transplant. 2012:247–256. PubMed PMID: 23721029.
- Kamar N, Del Bello A, Congy-Jolivet N, et al. Incidence of donor-specific antibodies in kidney transplant patients following conversion to an everolimus-based calcineurin inhibitor-free regimen. Clin Transplant. 2013 May-Jun;27(3):455–462. PubMed PMID: 23621682.
- Jouve T, Noble J, Rostaing L, et al. Tailoring tacrolimus therapy in kidney transplantation. Expert Rev Clin Pharmacol. 2018 Jun;11(6):581–588. PubMed PMID: 29779413.
- Shuker N, Bouamar R, Hesselink DA, et al. Intrapatient variability in tacrolimus exposure does not predict the development of cardiac allograft vasculopathy after heart transplant. Exp Clin Transplant. 2018 Jun;16(3):326–332. PubMed PMID: 28969528.
- Berger SP, Sommerer C, Witzke O, et al. Two-year outcomes in de novo renal transplant recipients receiving everolimus-facilitated calcineurin inhibitor reduction regimen from the TRANSFORM study. Am J Transplant. 2019;19(11):3018–3034.
- Hricik DE, Formica RN, Nickerson P, et al. Adverse outcomes of tacrolimus withdrawal in immune-quiescent kidney transplant recipients. J Am Soc Nephrol. 2015;26(12):3114–3122. PubMed PMID: 25925687.
- Dugast E, Soulillou JP, Foucher Y, et al. Failure of calcineurin inhibitor (Tacrolimus) weaning randomized trial in long-term stable kidney transplant recipients. Am J Transplant. 2016 Nov;16(11):3255–3261. PubMed PMID: 27367750.
- Hage V, Ferrandiz I, Belliere J, et al. Incidence of donor-specific anti-HLA antibodies in non-HLA-sensitized patients given tacrolimus once or twice daily during the first 2 years after kidney transplant. Exp Clin Transplant. 2018 Dec 31;17(3):313-319. PubMed PMID: 30602364.