ABSTRACT
Introduction: The gold standard for diagnosis of coronavirus disease 2019 (COVID-19) is detecting severe acute respiratory syndrome coronavirus 2 (SARS-CoV-2) by reverse transcription polymerase chain reaction (RT-PCR), which is expensive, time-consuming and may result in false-negative results. Serological tests can be employed for RT-PCR negative patients, contact tracing, determining the probability of protection against re-infection, and seroepidemiological studies.
Areas covered: The main methodologies of serology-based tests for the detection of SARS-CoV-2 including enzyme-linked immunosorbent assays (ELISAs), chemiluminescent immunoassays (CLIAs) and lateral flow immunoassays (LFIAs) were reviewed and their diagnostic performances were compared. Herein, a literature review on the databases of PubMed, Scopus and Google Scholar between January 1, 2020 and June 30, 2020 based on the main serological methods for COVID-19 detection with the focus on comparative experiments was performed. The review was updated on December 31, 2020.
Expert opinion: Serology testing could be considered as a part of diagnostic panel two-week post symptom onset. Higher sensitivity for serology-based tests could be achieved by determining combined IgG/IgM titers. Furthermore, higher sensitive serological test detecting neutralization antibody could be developed by targeting spike (S) antigen. It was also demonstrated that the sensitivity of ELISA/CLIA-based methods are higher than LFIA devices.
1. Introduction
Severe Acute Respiratory Syndrome Coronavirus 2 (SARS-CoV-2) is a single-stranded, positive-sense RNA virus and causes Coronavirus Disease 2019 (COVID-19). The appearance of the disease started in China and then spread worldwide. The high rate of transmissibility, environmental stability of SARS-CoV-2, and the severity of illness in high-risk populations led to the pandemic level of COVID-19 [Citation1]. In March 2020, World Health Organization (WHO) announced COVID-19 as the first pandemic caused by a coronavirus [Citation2]. The clinical appearance of COVID-19 ranges from being asymptomatic to fever with mild respiratory illness, to critically acute respiratory distress syndrome and death from respiratory failure or related difficulties [Citation3–5]. One of the pivotal interventions to control SARS-CoV-2 transmission is its early detection [Citation4].
As stated in WHO guideline, COVID-19 cases are confirmed by detection of unique sequences of the virus RNA using nucleic acid amplification tests (NAAT) such as real-time reverse transcription-polymerase chain reaction (rRT-PCR) in sputum, throat swabs, and secretions of the lower respiratory tract [Citation6,Citation7]. rRT-PCR, the reference standard method, diagnoses SARS-CoV-2 infection with high sensitivity and accuracy in the acute phase of COVID-19 illness [Citation4]. However, rRT-PCR is time-consuming and needs 4–6 h to be performed. It also needs costly specialist equipment, skillful laboratory staff for sample preparation and testing [Citation7]. Furthermore, false-negative results due to low viral load in the upper respiratory may occur using RT-PCR analysis.
Additionally, the peak of viral load in upper respiratory tract secretions is within the first week of symptoms and can become undetectable by 14 days post-symptom onset [Citation4,Citation7]. Furthermore, problems in different steps of real-time RT-PCR, including sampling, RNA extraction, and PCR amplification, may result in failure in detecting COVID-19 patients by real-time RT-PCR. However, many of these problems do not happen in detecting antibodies in serum samples [Citation8]. The time point of infection and pre-analytical factors (samples type, samples collection, storage conditions) affect viral load and, subsequently, the results of NAATs [Citation9]. In comparison to molecular methods, serological tests can be performed faster with lower cost, high-throughput, and less workload [Citation4,Citation9]. When the viral load of patients is below the detection limit of RT-PCR assays, serological diagnosis becomes very valuable and can be used complementary to NAATs [Citation9,Citation10]. However, as seroconversion usually occurred at 3–14 days post-symptom onsets, early diagnosis of COVID-19 using only serological tests may not be possible. Moreover, the specificity and sensitivity of serological tests are affected by infection time course [Citation11]. Overall, serology tests can be suitable in the following settings: 1) diagnosis of patients more than 7 days after the onset of symptoms, 2) diagnosis of patients with negative RT-PCR test and strong epidemiological and clinical evidences suggesting COVID-19 infection, 3) contact tracing, 4) determining potential immunity and the probability of protection against a re-infection, and 5) seroepidemiological studies to understand the vastness of COVID-19 circulation in the community [Citation12–14].
Different serological tests are accessible to determine the antibody response elicited by SARS-CoV-2 infection. The main methodologies include enzyme-linked immunosorbent assays (ELISAs), chemiluminescent immunoassays (CLIAs), lateral flow immunoassays (LFIAs) ()). Furthermore, neutralization (NT) assays measure neutralizing antibodies that have the ability to effectively bind to virus and block its replication [Citation15]. In this review, we aimed to gather the results of different validation studies related to serological tests of SARS-CoV-2 infection. Furthermore, we also compared the diagnostic performance of different methods. Accordingly, the main serological methods were described. Then, comparisons between cases in each method and comparison between different methods were explained ()).
Figure 1. The main serological methods for detection of SARS‑CoV‑2 infection
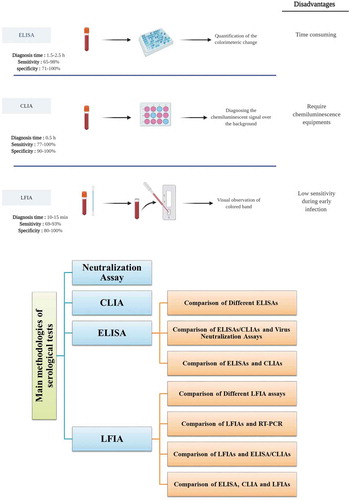
2. Method
The literature review was performed on the databases of Pubmed, Scopus and Google Scholar from January 1, 2020 to June 30, 2020 and updated on December 31, 2020. The keywords of (‘COVID-19’ or ‘SARS-CoV-2’) and (‘Serological test’ or ‘Serological assay’ or ‘CLIA’ or ‘ELISA’ or ‘LFIA’) were used. Furthermore, the search is limited to the English language. To identify publications of interest, the articles mostly focused on the comparison of different serological tests were selected.
3. Anti-SARS-CoV-2 antibody level and disease severity
It was found that irrespective of the applied immunoassays, serum antibody was detected with lower rate in post-positive RT-PCR individual without any symptoms (asymptomatic individual) [Citation9]. For example, in a study of Zhou et al., the difficulty of antibodies detection in asymptomatic cases by both gold immunochromatographic (IC) assay and CLIA may be related to low viral loads in asymptomatic cases [Citation17]. In another study, SARS-CoV-2 antibodies were detected in only 20% of asymptomatic cases 3 weeks after the diagnosis [Citation18]. Also, COVID-19 patients suffering from pneumonia showed shorter seroconversion time than those without pneumonia, as detected by point-of-care (POC) tests [Citation19]. Therefore, the stage of the disease strongly affects the detection rates of immunoassays [Citation9].
Some studies have shown a strong correlation between disease severity and anti-SARS-CoV-2 antibody titers [Citation20,Citation21]. For example, it was demonstrated that disease severity is positively correlated with serum receptor-binding domain (RBD)-specific IgA concentrations [Citation22,Citation23]. Perera et al. also revealed that significant higher levels of IgG are detected by RBD-based ELISA in severe/critical cases [Citation24]. On the other hand, some studies demonstrated no correlation between clinical severity and antibody levels [Citation9,Citation20,Citation25]. GeurtsvanKessel and colleagues also demonstrated that the severity of the disease does not influence the range of detected neutralizing titers or sensitivity of the examined assays [Citation13]. Altogether, it can be concluded that there is not any agreement about the correlation between disease severity and antibody titers at the present time [Citation20]. The association of disease severity and antibody titers should be examined in a larger patient cohort [Citation24].
4. Antibody profiling in SARS-CoV-2 infection
Knowing antibody profile is very important to request serological assays at the proper time and to properly interpret antibody test results [Citation10]. Initial studies demonstrated that similar to typical viral infection, IgM antibodies against SARS-Cov-2 might be developed earlier, and IgG level rises when IgM comes down [Citation26]. Zhou et al. found no general principle for the sequential order of IgM and IgG seroconversion for a specific COVID-19 patient [Citation17]. However, some studies demonstrated earlier IgG seroconversion than IgM [Citation9,Citation10]. Long et al. reported 3 types of seroconversion: simultaneous seroconversion of IgG and IgM, earlier and later IgM seroconversion than IgG. They demonstrated 100% IgG seroconversion within 19 days after symptom onset [Citation27].
These inconsistent results in terms of the chronological order of IgM and IgG emergence as a result of SARS-CoV-2 infection could be described by different sensitivity of immunoassays. This conflicting results support the detection of both antibodies, simultaneously [Citation9]. The initial infection of SARS-CoV-2 at the respiratory system highly containing mucosal immune cells may be the cause of the early development of IgA in the sera of COVID-19 patients. Addition of IgA to an ordinary serological test containing IgM and IgG increased the sensitivity of SARS-CoV-2 diagnosis in the early stage of infection, and higher diagnostic accuracy for COVID-19 may be provided [Citation22]. However, Coste et al. revealed that tests detecting only IgM and IgA or IgM+IgA demonstrate insufficient performances for the early diagnostic of COVID-19 [Citation28].
In the study of Lou and colleagues, the total antibody was reported to be detected as the first serology marker followed by IgM and IgG, with a median seroconversion time of 9, 10 and 12 days post-disease onset (d.p.o), respectively. Therefore, the highest sensitivity in the early stage of illness (0–7 d.p.o) was total antibody [Citation29]. It was also reported that in the early stage of the illness (0–10 d.p.o.), the positive rate of IgM and IgG ELISAs is less than 60%. Liu et al. demonstrated that the positive rate of IgM and IgG detected by ELISA method is increased when the number of d.p.o. is increased. However, the positive rate of IgM declines after 35 d.p.o [Citation30]. Adams et al. demonstrated that IgG titers rises over the first 3 weeks of infection, and no additional case could be recognized by the detection of IgM using ELISA [Citation31]. Xia et al. also demonstrated that the diagnostic sensitivity could be enhanced when the total antibody is detected while specificity remains similar. Therefore, detecting the total antibody is a better serological diagnostic indicator, especially for early diagnosis [Citation32].
5. Neutralizing antibodies in COVID-19 patients
Wu et al. demonstrated long duration of viral shedding with a median period of up to 14 days after seroconversion [Citation19]. Torres and Rinder stated that a positive antibody result does not ensure lack of viral shedding, probably due to the presence of non-neutralizing antibodies [Citation33]. It was found that significantly higher neutralizing antibody (nAb) against SARS-CoV-2 is elicited in the elderly (31–84) than younger patients (16–30). Furthermore, convalescent patients demonstrated a higher nAb level than inpatients. Moreover, higher nAb titer was detected in patients with severe clinical condition [Citation34]. It was found that the titer of nAb is lower in asymptomatic cases compared to pneumonic COVID-19 patients. However, nAb was found in most of the mild patients infected by SARS-CoV-2 after the third week of illness, even in asymptomatic individuals [Citation35]. Besides, it was assumed that other immune responses, including T cells or cytokines along with nAbs, may contribute to the recovery. The presence of nAbs may or may not correlate with protection. Additionally, WHO has recently warned that positive serological tests are not similar to protective immunity [Citation30]. Further studies are required to examine whether high level of nAbs keeps the COVID-19 patients safe from progression into severe or critical conditions [Citation34].
6. Comparison of nucleocapsid and spike proteins of SARS-CoV-2
It is very important to choose a proper recombinant SARS-CoV-2 protein for reliable serological assays [Citation36]. Four major structural proteins, including membrane (M), envelope (E), nucleocapsid (N), and spike (S), as well as non-structural proteins, are encoded by coronavirus RNA [Citation22] (). N and RBD of S protein are the most common antigens that can be used as an immobilized antigen for creating a serological COVID-19 diagnostic test [1]. The S glycoprotein is highly glycosylated and has 66 N-linked glycosylation sites per trimer, and a few of them are the target of nAbs [Citation37]. The N-terminal S1 domain includes the N-terminal domain (NTD) and RBD for the host cell receptor angiotensin-converting enzyme-2 (ACE2). The S2 domain mediates viral cell membrane fusion. Upon binding RBD to ACE2, the S protein undergoes a conformational change and rearranges from a pre-fusion to a post-fusion state, allowing the fusion of viral and target cell membranes [Citation38]. S protein is the most specific structural proteins for generating protective nAbs. Antibodies against RBD are strongly related to virus neutralization [Citation22,Citation39]. It was found that the probability of predicting protective antibody responses will be enhanced if S1 antigens or RBD is used in the assay [Citation40]. Cell-mediated immunity is elicited by two predominant CD8+ T epitopes of N protein [Citation22,Citation39].
Ma et al. revealed that diagnostic accuracy of RBD-based test is better than that of N-based. The outperforming of the RBD-based test may be due to its expression in a human cell line providing critical glycosylation for high-affinity interaction with antibodies generated in COVID-19 patients [Citation22]. In the study of Zhang et al., 3 prokaryotically expressed N proteins (full-length recombinant N protein (rN), NH2 (rN1), and COOH terminal (rN2) fragments of N protein) and 3 eukaryotically expressed spike proteins including rS1, rS-RBD, rS-RBD-mFc (S-RBD ligated to the Fc mouse fragment) were prepared. Initial ELISA evaluation of these recombinant proteins demonstrated that the highest coronavirus-specific IgM and IgG titers are obtained by rS1 and rS-RBD-mFc. The better performance of rS-RBD-mFc compared to rS-RBD may be due to the enhancement of half-life and stability of RBD protein by Fc fragments [Citation36]. It was also demonstrated by Liu et al. that rS-based ELISA is significantly more sensitive than rN-based ELISA for IgM detection. Relatively high, and early antibody response targeted to the S antigen was elicited in patients with COVID-19 compared to the N antigen [Citation30]. The higher sensitivity for S-based immunoassays may be related to the earlier immune response to the S antigen compared to the N antigen in COVID-19 patients [Citation9]. Therefore, for early screening of negative RT-PCR suspected COVID-19 patients, rS-based immunoassays are suggested [Citation30]. However, Burbelo et al. found that the sensitivity of luciferase immunoprecipitation system (LIPS) assay for detecting antibody targeted to the N protein of SARS-CoV-2 in plasma samples of COVID-19 patients obtained ≥14 days after symptom onset is more than those targeted to S protein [Citation41]. In addition, Schnurra et al. revealed that N- and S-based SARS CoV-2 antibody tests had a comparable range of sensitivities, and both of these antigens are proper for serological diagnostics. As negative sera in N-based SARS-CoV-2 antibody tests are different from that in S-based tests, combining N-and S-based tests may enhance true positivity and can be beneficial when extremely sensitive antibody tests are not accessible. However, due to increasing cost and labor, these successive test algorithms should be considered carefully [Citation42].
7. Appraisal of test performance
True positive (TP), true negative (TN), false negative (FN), and false-positive (FP) are general terms used to explain sensitivity and specificity. Sensitivity is the chance that a SARS-CoV-2-infected person obtains a positive test result. Specificity is the chance that a non-infected person obtains a negative result. Sensitivity (true positive rate) and specificity (true negative rate) are calculated as TP/(TP + FN) and = TN/(TN + FP). Positive predictive values (PPV) is the chance that a person whose serological test is positive truly has antibodies to SARS-CoV-2. Negative predictive values (NPV) demonstrated how likely it is that a person who has a negative result from a test truly does not have antibodies to SARS-CoV-2. PPV and NPV depend on the prevalence of SARS-CoV-2 infection and personal risk assessment and clinical decision making [Citation43].
The required performance of an antibody test is related to its particular aim, which can be either population screening (in the general population or at-risk populations) or diagnostic support [Citation13]. Therefore, the aim of a serological test is very important for determining proper thresholds for sensitivity and specificity. High sensitivity (generally ≥90%) is necessary for diagnosing asymptomatic carriers. Specificity is less pivotal because some false-positive results can be endured. However, in performing antibody tests to notify release from quarantine, high specificity is critical, as false-positivity can enhance the risk of exposure for nonimmune individuals [Citation31].
The effect of population prevalence should also be considered when test performance is evaluated. It was found that even a highly specific test can produce many false-positive results, particularly in case of low population prevalence [Citation31,Citation44]. This can be considered as the number of false-positive tests per 1000 people tested or as the ratio of wrong positive tests to all positive tests. For example, when a test with 99% sensitivity and specificity is used in a population with a 5% prevalence of seropositivity, 16% of positive results will be false. Therefore, 950 of 100,000 people will be false-positive and wrongly be informed that they have had a previous infection [Citation45]. Personal or societal decisions based on wrong reassurance can be damaging and promote further outbreaks [Citation31]. Consequently, similar to HIV testing in low prevalence settings, it may be required to perform a ‘two-step’ approach for SARS-CoV-2 serological testing to simplify seroepidemiological studies. In this approach, a sensitive high-throughput screening assay is followed by a high specific assay for confirmation (e.g., neutralization testing or Western blot) [Citation46].
8. Neutralization assays
Most of the current large-scale serological assays detect binding antibodies and do not detect nAbs. At present, virus NT assay is the gold standard for the evaluation of protective immunity against SARS-CoV-2. This method is used to answer whether or not the measured antibody is correlated with the functional antibodies that can keep a population safe during a further exposure [Citation13].
In plaque reduction NT test (PRNT), the gold standard for detecting protective immunity, nAbs targeted against viruses is measured. The method is based on the cytopathic effect (CPE) that damaged virus-infected target cells are visually observed under a microscope. Serum dilutions causing plaque reductions of 90% (PRNT90) and 50% (PRNT50) are noted as titers [Citation47]. PRNT is a time‐consuming technique, which can only be carried out by skillful staff, and only in a biosafety level 3 (BSL3) laboratory for live SARS-CoV-2 virus handling [Citation48]. Live virus-based microneutralization (MN) is a highly sensitive and specific technique and measures virus-specific nAbs in patients’ blood [Citation30,Citation47,Citation49]. In the MN assay conducted by Perera et al., the CPE effect was read after adding serum- SARS-CoV-2 virus mixture to Vero E6 cell in 96-well plates [Citation24]. It was demonstrated that the sensitivity of PRNT90 test was higher than MN tests when conventional 100 tissue culture infectious dose (TCID50) was used in the MN test. MN assays with decreased virus challenge dose (50 or 25 TCID50) resulted in increased sensitivity but might compromise specificity [Citation24]. Four- to sixteen-fold higher titer was achieved by MN assay using plasma sample rather than concurrent serum. This difference was due to the contribution of heparin in reducing the infectivity of the virus challenge dose, and thus heparinized plasma should be avoided in NT assay [Citation24]. Amanat et al. developed an in vitro MN assay to quantitatively measure nAbs or drugs, which can block entrance and/or replication of SARS-CoV-2. In this medium-throughput method, which could be performed in a 96-well cell culture plate, the readout was not based on visual inspection of CPE and relied on immunostaining of the virus nucleoprotein [Citation50]. Because of the low throughput of PRNT, it cannot be used for large-scale serodiagnosis and vaccine evaluation. Therefore, a high-throughput fluorescence-based nAb assay was developed for COVID-19 diagnosis. In this nAb assay, a stable mNeonGreen SARS-CoV-2 in which mNeonGreen gene was genetically modified at the viral genome, was neutralized with COVID-19 patient sera. Vero cells were infected with reporter virus/serum mixture and then the fluorescence of infected cells were measured to determine the NT50 value of each serum sample. A strong correlation between the reporter virus (NT50) and PRNT (PRNT50) results was demonstrated. This platform can be used for rapid large-scale screening of people for detection of protective antibodies and can advise policymakers for reopening local communities [Citation51].
Furthermore, pseudovirus-based neutralizing assays (PBNAs) are applied as alternative methods. In PBNA, viruses are without virulent parts of the virus and involve a single round of replication. Therefore, they are much safer to handle and require BSL2 laboratory [Citation52]. Since an authentic virus is not used in this test, the results always demonstrate an approximation of ‘real’ virus NT [Citation50].
In another approach, a surrogate virus neutralization test (sVNT), which detects nAbs was developed by Tan et al. (). This method can be performed within 1–2 hours in a BSL2 lab and does not need any live virus. In this assay, the interaction between RBD and ACE2 receptor is inhibited by highly specific nAbs in patient or animal sera in an ELISA plate well. Advantages of sVNT include its ability to detect total SARS-CoV-2 nAbs in an isotype-independent and species-independent manner [Citation53]. In the validation study of the developed sVNT with two COVID-19 patient cohorts, the sensitivity of 95–100% and specificity of 100% were reported [Citation53]. However, Bond and colleague reported sensitivity of 60.9% rising to 91.2% for samples collected >14 days post-symptom onset, along with a specificity of 94.4% for sVNT assay. Specificity can be enhanced by using higher inhibition cutoff or performing the test in triplicate in samples within an arbitrary range. For example, in a low-prevalence setting when the inhibition cutoff for sVNT assay was raised to 25%, the specificity was increased to 99.3%, and thus clinical utility could be improved. Overall, determining specific cutoffs for populations with different ethnic or geographic background and introduction of an equivocal range with repeated testing for samples within this range may reduce potential variation in sVNT assay [Citation46].
Figure 3. Mechanism of surrogate virus neutralization test (sVNT)
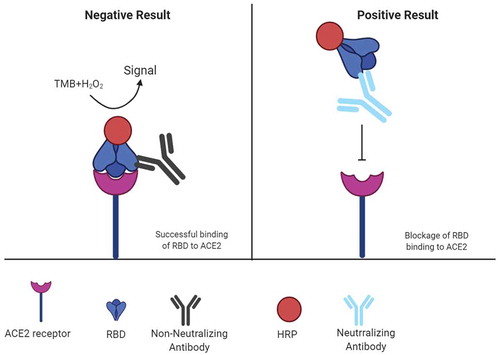
9. Chemiluminescent immunoassays (CLIAs)
When an electron transits from an excited state to the ground state, visible or near-visible radiation is emitted, which is called luminescence. Chemiluminescence is a light-emitting process in which chemical reactions are the energy source for generating the electronically excited state [Citation54]. Immunoassay technique in which the ‘indicator’ of the analytic reaction is a luminescent molecule called chemiluminescent immunoassay [Citation54]. This laboratory technique benefits from the specificity of the immune response and also the sensitivity of the luminescence reaction. It can be automated and quickly used on immunochemical analyzers for a high throughput of samples [Citation1,Citation55]. The principle of a CLIA is the same as an ELISA with shorter incubation steps and no need for a reagent to stop the enzymatic reaction [Citation15].
In the first step of the chemiluminescence-based method, SARS-CoV-2 specific IgA, IgM and IgG in sera were captured via SARS-CoV-2 antigen (N or RBD)-coated magnetic particles. Then, a second acridinium-conjugated antibody that detects human IgA, IgM or IgG was applied. Reaction of acridinium with its substrates caused strong chemiluminescence. Afterward, the diagnosed chemiluminescent signal over the background was measured as relative light units (RLU) [Citation55]. Requiring supporting chemiluminescence instruments [Citation16] and qualified personnel [Citation56] are the disadvantages of CLIA assays. One of the usual obstacles in chemiluminescence immunoassay is false-positive results. It was found that CLIA against multi-antigens (N + S proteins) has higher specificity than single antigen proteins, but still reducing false positives is required [Citation17].
It was demonstrated that the performance of CLIA for detecting IgM is not suitable enough. This could be due to its low concentration and that IgM titer rises then declines rapidly in early and mid-infection. Therefore, measuring total antibodies instead of measuring IgM and IgG alone is suggested for defining the progress of infection and diagnosis [Citation17]. In the study of Wan et al., among the examined CLIAs, the test performance of the one detected total antibody was the best and could be applied as an alternative method for nucleic acid testing [Citation55].
9.1. Examples of CLIAs
9.1.1. LIAISON SARS-CoV-2 S1/S2 IgG (DiaSorin, Saluggia, Italy)
LIAISON SARS-CoV-2 S1/S2 IgG assay, a standardized automated chemiluminescent assay, measured antibodies against the SARS-CoV-2 native S1/S2 proteins. The automated LIAISON SARS-CoV-2 S1/S2 IgG assay was examined in >1500 samples and its performance was sensitive, specific and precise with a high throughput capacity. The first results by automated LIAISON SARS-CoV-2 S1/S2 IgG assay are accessible within 35 minutes with a throughput of 170 tests/hour. The sensitivity of the LIAISON SARS-CoV-2 S1/S2 IgG assay was significantly enhanced as the immune response matures. As S1 and S2 proteins are targets for nAbs, the probability of agreement between LIAISON and NT assay is enhanced. Furthermore, LIAISON assay could separate NT assay-negative samples from NT assay-positive samples with good sensitivity and specificity. When using LIAISON in a semi-quantitative manner, higher units indicated higher NT assay titers. Therefore, large numbers of samples can be screened via LIAISON to detect recovering plasma for treatment of patients with acute SARS-CoV-2 [Citation39]. In the study of Herroelen et al., significant lot-to-lot alternation in the raw signals of the two LIAISON SARS-CoV-2 S1/S2 IgG kits were reported. As a result, optimizing cutoffs are suggested to properly evaluate different lots [Citation57]. Plebani et al. also demonstrated that the best negative likelihood ratio can be achieved by redefining cutoffs for LIAISON and iFlash [Citation58].
9.1.2. Atellica IM SARS-CoV-2 total (COV2T) (Siemens Healthcare, Erlangen, Germany)
The COV2T assay is a chemiluminescent microparticle immunoassay (CMIA), which quantitatively measures total antibodies (including IgG and IgM) targeted to RBD SARS-CoV-2 in human serum and plasma. The results are obtained in 10 min on the Atellica IM Analyzer with a capacity to process up to 440 assays per hour [Citation42].
9.1.3. Abbott ARCHITECT SARS-CoV-2 IgG immunoassay (Abbott Diagnostics, Chicago, US)
Abbott SARS-CoV-2 IgG assay is a fully automated indirect CMIA to detect IgG antibodies directed to SARS-CoV-2 rN antigen [Citation59,Citation60]. Chew et al. demonstrated that clinical sensitivity of the Abbott SARS-CoV-2 IgG depends on the sampling time from the onset of symptoms. The clinical sensitivity of samples taken ≥14 days after the onset of clinical illness was significantly higher than those taken <14 days. However, no considerable differences were detected between 14 and 20 days and ≥21 days from the onset of illness. Therefore, taking samples 14 days from onset of illness may be considered as an adequate threshold for retrospective identification of COVID-19 patients [Citation59]. Schnurra et al. revealed that the sensitivity of Abbott is a bit less than that determined by the manufacturer [Citation42].
9.1.4. iFlash1800 (YHLO Biotechnology Company, Ltd, Shenzhen, China)
iFlash-SARS-CoV-2 is a paramagnetic particle CLIA for the detection of IgM and IgG antibodies against SARS-CoV-2 N and S proteins [Citation58]. The sensitivity of iFlash-SARS-CoV-2 was reported to be less than 50% before day 8 post-symptom onset, and it was increased to 81.8% 9–10 days after symptom onset for both IgM and IgG. A sensitivity of 100% was obtained 15 days or later after symptom onset when IgG was measured [Citation61].
9.1.5. MAGLUMI (SNIBE – Shenzhen New industries biomedical engineering company, Ltd, Shenzhen, China)
The MAGLUMI 2019-nCoV IgG and IgM, indirect CLIAs on the fully automated MAGLUMI analyzers, determine IgG and IgM antibodies against SARS-Cov-2 S and N proteins in human sera [Citation62].
9.1.6. Elecsys Anti-SARS-CoV-2 diagnostics (Rotkreuz, Switzerland)
Elecsys anti-SARS-CoV-2, a new electrochemiluminescence immunoassay (ECLIA) () for in vitro qualitative detection of total antibodies (IgA, IgM, and IgG) directed against the SARS-CoV-2 N antigen, is performed on the cobasVR e801 immunoassay analyzers. Excellent clinical performance could be obtained 14 days after RT-PCR positivity or the beginning of COVID-19 symptoms by optimal cutoff provided by the receiver operating characteristic (ROC) curve [Citation63].
Figure 4. Electrochemiluminescence-based anti-SARS‑CoV‑2 immunoassay
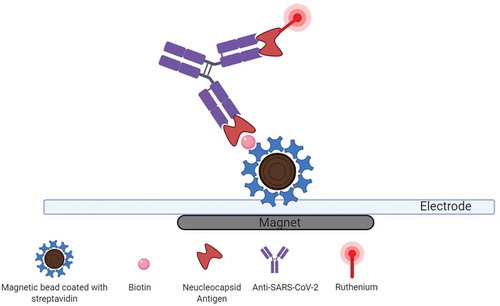
9.1.7. Synthetic peptide-based Magnetic Chemiluminescence Enzyme Immunoassay (MCLIA)
In the study of Cai et al., twenty peptides from the orf1a/b, S, and N proteins were synthesized as candidate antigens and were labeled with biotin. Then, the biotinylated peptides were purified and bound to streptavidin-coated magnetic beads. Afterward, serum samples were mixed with peptide-containing beads. Finally, after reaction with antibody conjugate and substrate, reactivity was determined by a luminescence reader. Overall, a MCLIA based on the synthetic peptide from the S protein was developed for determining IgG and IgM against SARS-CoV-2. In the study conducted on sera of 276 patients with confirmed SARS-CoV-2 infection, the positive rate of IgG and IgM was 71.4% and 57.2%, respectively. The relatively low homology of synthetic peptide and other coronaviruses may lead to good specificity of this assay. The stability of this assay based on the coefficient of variation (CV) of IgG and IgM detection in different concentration of samples was also perfect. Therefore, the diagnostic accuracy of COVID-19 may be enhanced by combining peptide-based MCLIA with real-time RT-PCR [Citation8].
10. Enzyme-Linked Immunosorbent Assay (ELISA)
Generally, ELISA, the gold standard of immunoassay, detects and quantifies substances including antibodies, antigens, proteins, glycoproteins, and hormones. To detect patient antibodies, a known capture antigen is immobilized on the plate and then the patient’s antibodies in a sample bind to the immobilized capture antigen. After that, an enzyme-labeled detection antibody specific to any antibody isotypes (i.e., IgG, IgM, etc.) forms a complex with the captured patient antibodies. Next, the interaction of enzyme (usually horseradish peroxidase (HRP)) and its substrate causes a colorimetric change that can be quantified and correlated to the presence and/or concentration of the antibody [Citation15]. Most of ELISA validation studies focused on comparing different antigens and antibody isotypes for achieving the best sensitivity and specificity [Citation1]. ELISA is not a very expensive method. It can be simply operated and made high throughput with an automated work station. However, this technique is time‐consuming and at risk of contamination. It also needs infrastructure and qualified personnel [Citation16].
10.1. Examples of ELISA
10.1.1 Wantai SARS-CoV-2 total Ig and IgM ELISAs (Beijing Wantai Biological Pharmacy Enterprise Co., Ltd., China)
Total antibodies against RBD of SARS-CoV-2 including IgM, IgG and IgA are measured using a well-validated Wantai ELISA kit. The sensitivity of Wantai SARS-CoV-2 Ab ELISA was increased to 79% in patients with at least 7 days of symptoms [Citation64]. Marlet and colleagues demonstrated that the sensitivity for Wantai Ab 14 days after onset of the symptom ranges 98–100% and 98.0–99.1%, respectively [Citation65].
10.1.2. Euroimmun Anti-SARS-CoV-2 IgG and IgA ELISA assays (EUROIMMUN Medizinische Labordiagnostika AG, Lübeck, Germany)
IgA and IgG antibodies targeted against the recombinant S1 domain of the SARS-CoV-2 are semi-quantitatively measured by Euroimmun ELISA technique. Beavis et al. reported 90.5 and 100% agreement for IgA and IgG detection by Euroimmun anti-SARS-CoV-2 ELISA assay in samples collected ≥4 days after COVID-19 confirmation by RT-PCR, respectively. They showed that both sensitivity and specificity for detection of IgA by Euroimmun anti-SARS-CoV-2 ELISA assay in samples collected ≥4 days after COVID-19 diagnosis by PCR is good and for detection of IgG is excellent [Citation66]. It was found that anti-SARS- CoV-2 IgA detection by Euroimmun was earlier than IgG detection [Citation57,Citation67]. However, poor specificity is the disadvantage of this method. The manufacturer confirmed low specificity for IgA ELISA, and thus it is not recommended to perform IgA ELISA for screening of asymptomatic persons [Citation26]. It was also found that specificity was low (72.8%) in case of considering both IgA and IgG components [Citation46]. Additionally, specificity of below 90% was reported by Tuaillon and colleagues for Euroimmun IgG and IgA assays [Citation67]. In case of achieving low or moderate positive Signal/cutoff (S/CO) ratio by Euroimmun tests, Tuaillon et al. recommended performing a second IgA/IgG test to verify the rise of the signal [Citation67]. Bond et al. revealed that specificity is increased to 97.8% when IgG alone is considered [Citation46].
10.2. Comparison of different ELISAs
Both Wantai and Euroimmun ELISA assays measure antibodies targeted to the S1 subunit of the SARS-CoV-2 spike protein; however, the Wantai Total Ab ELISA only detects the RBD within the S1, which is approximately 33% of the S1 subunit and is highly diverse between SARS-CoV-2 and other beta-coronaviruses. Therefore, the probability of cross-reaction with epitopes outside of this domain and other circulating coronaviruses may be reduced [Citation12]. Lassaunière et al., reported that the ELISA assays detecting total SARS-CoV-2 RBD-specific antibodies are more sensitive than that detecting spike-specific IgA or IgG only. The differences between the assays may be attributed to the targeted SARS-CoV-2 antigen and the used ELISA format [Citation12]. Herroelen et al. [Citation57] also reported superior diagnostic performance of the Wantai SARS-COV-2 Ab ELISA compared to Euroimmun anti-SARS-CoV-2 IgG and IgA ELISA assays. Tuaillon et al. reported that the N-based ELISA (ID.Vet test) is more specific than S1-based ELISA (Euroimmun). However, the sensitivities obtained by these ELISA assays were comparable [Citation67].
10.3. Comparison of ELISAs/CLIAs and virus neutralization assays
Prior infection with SARS-CoV-2 could be evidenced by a positive result using RBD-based ELISA; however, the presence of nAbs would provide greater assurance of protective immunity [Citation24]. The correlation between S-based ELISA and the neutralizing assay was reported in many studies (). For example, ELISA reactivity using the mammalian cell-expressed S proteins and neutralizing activity of sera against the USA-WA1/2020 isolate was correlated. It was also found that ELISA and MN titers are correlated significantly [Citation68]. Ni and colleagues found significant correlation between nAb titers and area under the curve (AUC) of anti-S-RBD IgG, but not of anti-N IgG. Therefore, anti-S-RBD IgG might be used for predicting the capability of serum neutralization in COVID-19 patients [Citation69]. Peterhoff et al. also reported strong correlation between RBD-based ELISA and virus neutralization [Citation70]. In a cohort of RT-PCR confirmed COVID-19 patients, all sera were positive by IgG and IgM RBD-based ELISA, MN, and PRNT90 assays 29 days post-onset of symptoms [Citation24]. Furthermore, detectable levels of nAbs were found in all positive samples with an OD ratio>10 evaluated by Wantai Ig ELISA in different stages and severity of the disease. Therefore, it can be suggested that the existence of nAbs using a cutoff in Wantai Ig ELISA is predictable [Citation13]. Additionally, among the examined ELISAs and CLIAs, Wantai was the most effective method for identifying donors with high NT titers [Citation71]. In the study of Patel et al., among the evaluated assays, the results of Euroimmun and Elecsys assays (Roche) demonstrated the highest and the weakest correlation with nAb titers, respectively [Citation72]. Padoan et al. also reported the weak correlation between N-based Elecsys Anti-SARS-CoV-2 Ab Tot levels and PRNT50 titers [Citation73].
Table 1. Comparison of COVID-19 ELISA/CLIA and neutralization assays
Perera et al. suggested that a valid strategy for large-scale seroepidemiological studies can be established by employing RBD-base IgG ELISA as a screening assay followed by confirmation using PRNT90 [Citation24]. However, due to the agreement between Abbott SARS-CoV-2 IgG immunoassay and virus MN test conducted by Meschi et al., neutralization activity may be predictable from the strong humoral response, regardless of the target antigens [Citation74].
10.4. Comparison of ELISAs and CLIAs
As described below, some studies demonstrated that the sensitivity of ELISAs is higher than that of CLIAs (). For example, among 5 different automated SARS-CoV-2 serology assays (Euroimmun, Wantai, Elecsys, LIAISON and Atellica), the most sensitive assay was S-protein based ELISA by Wantai followed by the N-protein based Elecsys assay. The specificity of over 99.5% was reported only for Elecsys and Atellica [Citation75]. Furthermore, among eight commercial assays based on CLIA, ELISA and enzyme-linked fluorescent assay (ELFA) technologies, ELISA from Wantai was the most and LIAISON assay was the least sensitive methods [Citation76]. In another study by Wolff et al., the highest overall sensitivity among the examined methods (Elecsys, LIAISON and Euroimmun) was achieved using Euroimmun with a combined detection of IgG/IgA, Euroimmun IgA and Elecsys, respectively [Citation9].
Table 2. Comparison of COVID-19 ELISAs and CLIAs
In contrast, some studies demonstrate the superior performance of CLIA-based technique over ELISA-based one. For example, comparing seven commercial antibody tests applying different techniques including ELISA (Euroimmun), bridge immunoassays such as microparticle immunoassay (Siemens), ECLIA (Roche) and CMIA (Abbott), the highest sensitivity was obtained by Siemens antibody test followed by the Roche, Euroimmun and Abbott tests. Detecting all immunoglobulin classes by Siemens and Roche could explain their high sensitivity [Citation42]. Furthermore, the largest agreement among the examined tests was reported between the Roche (detecting antibodies against N protein) and Siemens assays (recognizing antibodies against S protein) [Citation42]. Lin et al. also demonstrated significant higher sensitivity level for N-based CLIA compared to N-based ELISA. They attributed the sensitivity difference to the serum amount for the first incubation step and intrinsic methods [Citation78].
Many studies revealed that the diagnostic performance of CLIA is comparable to that of ELISA. Padoan et al. reported that the values of IgG measured by MAGLUMI CLIA and Euroimmun ELISA assay are comparable and the clinical agreement between these methods is 90.8% [Citation37]. It was also shown that Euroimmun Anti-SARS-CoV-2 IgA and IgG, and MAGLUMI IgM and IgG have 90% agreement at the respective cutoffs, especially for IgG and the cumulative immunoglobulin profile [Citation62]. In the study of Egger et al., Elecsys was compared with the EDI ELISA for the detection of SARS-CoV-2 antibodies in human plasma. The percentage of positive results was 100% for the Elecsys and 94% for EDI ELISA 15–22 days after symptom onset. The overall agreement between Elecsys assay and EDI ELISA (IgM or IgG) was acceptable in the confirmed COVID-19 patients. False positivity rates in the healthy blood donors and the ICU patients were <1% and <3% for Elecsys and EDI ELISA, respectively [Citation79]. Good agreement between LIAISON and Euroimmun ELISA IgG tests was reported in two studies as described in the following section. In the study of Tré-Hardy et al., no difference between these two techniques in terms of sensitivities and specificities for IgG detection was demonstrated [Citation80]. In another study conducted by Pflüger et al., among 5 different automated SARS-CoV-2 serology assays, the strongest correlation of quantitative results was reported between the LIAISON and Euroimmun IgG tests [Citation75].
Many studies recommended optimizing diagnostic cutoffs to obtain better diagnostic performances [Citation58,Citation62]. Adaptation of the cutoff for LIAISON and the Euroimmun ELISA tests showed a sensitivity of 100% for both tests and a specificity of 99% for LIAISON as well as 96% for Euroimmun ELISA test. Although the analytical and clinical performances of LIAISON are excellent for detecting IgG antibodies specifically directed against SARS-CoV-2, it is suggested to reestablish the cutoff in each center for preventing false-negative results [Citation80]. In the study of Plebani et al., the overall agreement between CLIA assays can be improved by adopting the threshold. The optimized thresholds were different from manufacturers’ suggested cutoffs, especially for MAGLUMI and LIAISON. The highest agreement and the best performance was obtained by adopting the threshold for MAGLUMI and LIAISON. Reestablishment of thresholds led to higher values for specificity, classification accuracy and positive likelihood ratio for MAGLUMI; sensitivity, accuracy and negative likelihood ratio for LIAISON; sensitivity and negative likelihood ratio for iFlash [Citation58].
11. Lateral flow immunoassays (LFIAs)
Qualitative (yes/no) results can be obtained by serological rapid diagnostic tests (RDTs) [Citation13]. Most of the rapid POC immunoassays are based on lateral flow immunoassay (LFIA) methodology [Citation44]. LFIA can be used as an easily mass-producible test platform and inexpensive scalable solution for population-scale diagnosis of SARS-CoV-2 in high-, and also low- and middle-income countries [Citation81]. The principle behind LFIAs, a paper-based platform, is migrating liquid sample containing the target analyte without the help of external forces (capillary action) through various zones of polymeric strips and interaction of immobilized capture antibody with the target analyte (). The result of LFIA-based tests are displayed within 5–30 min. In the first step, the liquid sample that contained the target analyte is put on an adsorbent sample pad, which generally standing on one part of the strip. The buffer salts and surfactants in sample pad treat sample matrix for better interaction of the analyte present in the sample with the dry reagents of detection system. Then, treated samples are moved toward another part of the strip named conjugate release pad, which contains conjugated target analyte-specific antibodies or conjugated-virus antigen. Generally, antibodies or virus antigens are conjugated to colored or fluorescent particles (e.g., colloidal gold or latex microspheres). After the interaction, the conjugated antibody/antigen along with the target migrates toward the detection zone of a strip, a porous membrane (usually composed of nitrocellulose) consists of capture antibodies (anti-human IgG and IgM antibodies) immobilized in lines. An absorbent pad is placed at the end of the strip, which absorbs the excess reagents, maintains liquid flows across the device and avoids the backflow of the liquid. An appropriate response on the test line demonstrates the presence of target analyte in sample, while a response on the control line indicates the proper liquid flow through the strip and therefore the validity of the test. Multiple analytes can be detected by different test lines on a device of LFIA [Citation82,Citation83]. The most common lateral flow immunochromatographic tests (ICTs) tests, which are usually known as dipstick tests, are over-the-counter pregnancy test kits [Citation83]. Simple operation, low cost and high speed are the advantages of LFIAs. However, the drawbacks of LFIAs are their low specificity and sensitivity [Citation16,Citation56].
Figure 5. Lateral flow immunoassays (LFIAs) for diagnosis of COVID-19
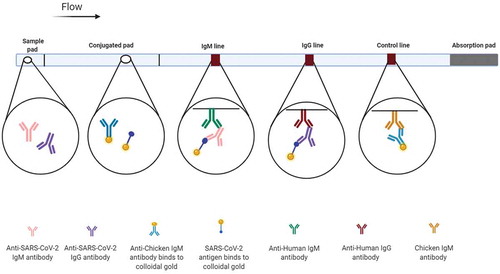
11.1. Different sample types for LFIAs
Different biological samples including urine, saliva, sweat, serum, plasma, whole blood and other fluids can be examined using LFIAs [Citation82]. Most of the studies detecting antibodies against SARS-CoV-2 antigens using RDT tests revealed no significant change in results when different samples are used [Citation85]. Due to lack of deviation between fingerstick blood, serum and plasma of venous blood, the developed LFIA kit can be applied for out‐of‐clinic screening using fingerstick blood or heel blood instead of vein blood [Citation86]. For example, Pan et al. reported excellent agreement between whole blood and plasma samples for the detection of antibody against SARS-CoV-2 antigens using IC strip [Citation85]. Bond et al. showed that the concordance between whole blood (finger prick) and plasma testing using OnSite and VivaDiag IgM/IgG rapid tests ranges from 81.8% to 88.1% [Citation87]. Prazuck et al. demonstrated that the sensitivity of direct antibody typing using RDTs with whole blood is the same as with serum in a retrospective way [Citation88].
11.2. Duration between symptom onset and performing LFIAs
It was found that the sensitivity of LFIAs are enhanced by increasing the time between sample acquisition and initial of the infection [Citation87]. The sensitivity of an IC assay with IgM and IgG combinatorial detection was 11.1, 92.9, and 96.8% at the early (1–7 days after onset), intermediate (8–14 days after onset), and late stage (more than 15 days), respectively [Citation85]. It was found that the detection sensitivity of the developed gold immunochromatography assay (GICA) by Zhang et al. can be increased by excluding the sera from patients within 5 days of symptoms onset [Citation36]. The sensitivity of 4 different IC tests examined by Demey et al. ranged between 60 and 80% on day 10 and was 100% on day 15 post-infection. Therefore, reliable results could be achieved by these IC tests at 14–15 days post-infection [Citation89]. The sensitivity of over 90% was achieved by 3 out of 6 POCs examined in the study of Tuaillon et al. when the samples were collected 15 days after the onset of symptoms [Citation67]. In a retrospective study, the diagnostic sensitivity was enhanced to over 87% between 15 and 21 days after symptom onset and attained to 100% three weeks post-symptom onset for all 4 examined POC antibody rapid tests [Citation19]. Elslande et al. reported that the sensitivity of LFIAs is not very good and is <60% at the time of hospital admission [Citation26]. It was also found that the sensitivity of LFIAs was limited in admitted patients. However, it was increased in patients with more than 7 days from symptom onset to sample collection and in patients with C-reactive protein (CRP) levels >100 mg/L upon presentation [Citation64].
11.3. IgM and/or IgG detection by LFIAs
It was found that no early and overall diagnostic value is added by POC rapid test detecting IgM separately from IgG antibodies against SARS-CoV-2 compared to POC rapid test detecting combined IgG and IgM antibodies [Citation19]. Tuaillon et al. reported that the detection of IgM before IgG by most of the POC tests used in their study is disappointing [Citation67]. Van Elslande et al. also revealed that the average time for IgM seropositivity detected by different LFIAs is not shorter than IgG seropositivity [Citation26]. In the study of Lee et al., day 5 and 42 were the earliest detection and longest persistence of SARS-CoV-2 IgM using rN-based IgG/IgM LFIA. In case of SARS-CoV-2 IgG, the earliest detection time was on day 5 and most cases persistently had positive IgG [Citation90].
Furthermore, in the study of Fujigaki et al. the IgM antibodies examined by 3 different IC kits were positive in few cases in the early stage of the disease (0–6 days post-onset symptoms). Additionally, IgM detection by these IC kits was low over the entire period and was improper for diagnosing acute phase of COVID-19. However, IgG was detected in almost all samples 13 days post-symptom onset. Therefore, IgG detection by the examined IC kits could be effective for determining COVID-19 infection in the recent past [Citation91]. It was also demonstrated that the sensitivity of POC test detecting IgM alone is not more than POC test detecting IgM/IgG POC test on samples collected during the second week of COVID-19 [Citation67].
Additionally, the specificity of the examined LFIAs in the study of Van Elslande et al. was decreased when detection of IgM antibodies was included in these tests. Altogether, the diagnostic performance of LFIAs was not improved when the results of IgG LFIAs and IgM LFIAs were combined. Therefore, to prevent false-positive results for IgM detection, it is suggested to develop LFIAs that detect only IgG [Citation26]. Candel et al. found that rapid serology tests (mainly IgG detection) could be useful for diagnosis and clinical management of COVID-19 patients in late stage (after more than 15 days of symptoms). However, they revealed that IgM response remained longer in severe critical cases than moderate cases. Therefore, they concluded that IgM detection by LFIAs may be correlated with the severity of the infection [Citation92].
11.4. Examples of LFIAs
11.4.1. COVID-PRESTO and COVID-DUO (AAZ-LMB, Boulogne-Billancourt, France)
COVID-PRESTO and COVID-DUO detect N-protein targeted IgG and IgM antibodies in fingertip whole blood samples. Anti-human IgM and IgG antibodies (test lines IgM and IgG) and rabbit IgG (control line) were immobilized on a nitrocellulose strip. Recombinant COVID-19 antigens labeled with colloidal gold were also incorporated into the strip [Citation88]. In 238 RT-PCR positive COVID-19 patients, it was shown that the specificity of both COVID-PRESTO and DUO RDTs are 100% (none false positive). The sensitivity of both of these RDTs is increased over time from the onset of symptoms. The sensitivity of both RDTs reached 100% after 15 days from the onset of symptom [Citation88]. It was found that the majority of COVID-PRESTO test users can correctly read the results. Therefore, it can be applied as a suitable self-test by general population [Citation84].
11.4.2. VivaDiag (VivaChek Biotech (Hangzhou) Co., Ltd., China)
The VivaDiag COVID-19 IgM/IgG rapid test determines COVID-19’s IgM and IgG antibodies, qualitatively. The manufacturer suggested that clinical findings should also be considered when the test result is interpreted. Bond et al. assumed that the performance characteristics of VivaDiag are below than stated by the manufacturer [Citation87]. Cassaniti et al. stated that VivaDiag COVID‐19 IgM/IgG rapid test is not advisable for triage of patients with suspected COVID‐19 due to obtaining negative results by this test in RT-PCR confirmed patients [Citation84]. However, Capello et al. intended to use VivaDiag COVID‐19 IgM/IgG rapid test for screening of SARS-CoV-2 infection in high-risk patients with no clinical signs of the disease [Citation93].
11.4.3. Nanozyme-based chemiluminescence
Liu et al. combined the high sensitivity of chemiluminescence, the high-specificity of immunoassay and the short testing time of lateral flow chromatography technique. Consequently, they developed a nanozyme-based chemiluminescence paper test for rapid, portable and ultra-sensitive detection of SARS-CoV-2 RBD antigen. They used Co-Fe@hemin nanozyme as an appropriate alternative to natural protease HRP for catalyzing chemiluminescence reaction. The signal amplification by nanozyme-catalyzed reaction resulted in a great improvement in the sensitivity of paper test comparing to traditional colloidal gold, fluorescence strip or nanozyme-based colorimetric strip, which was comparable to that of ELISA. Therefore, the false-negative rate might be decreased in the early screenings of virus infection. Due to excellent diagnostic performance, considerable portability and low-cost, nanozyme-based chemiluminescence paper test can be used as a novel POC approach for early screening of SARS-CoV-2 infected patients [Citation94].
11.5. Comparison of LFIA assays
In the study of Adams et al., no significant differences were reported between the sensitivity or specificity of 9 examined LFIA devices [Citation25]. However, many studies demonstrated a significant difference between sensitivities or specificities of different LFIAs. For example, a significant difference between two different IC methods was reported by Bartolini et al. They have demonstrated a sensitivity of 35.3% for KHB, which was greatly lower than Cellex (66.4%) [Citation95]. In another study, the sensitivity and specificity of antibody detection by nine different LFIAs ranged from 65% to 85% and from 93 to 100%, respectively [Citation12]. GeurtsvanKessel et al. also reported different specificities for the examined RDTs [Citation13].
11.6. Comparison of LFIAs and RT-PCR
The advantages of LFIA assay compared to RT‐PCR include simplicity of test, time-saving and no need to additional equipment and training. Therefore, it can be easily used at the bedside, in any clinic or laboratory, at airports or railway stations [Citation86]. However, results of LFIA assay were not promising in all studies and some of them have reported poor sensitivity in confirmed COVID-19 patients [Citation67]. For example, in the study of Bartolini et al., only 59.6% of the RT-PCR positive samples became positive by IC assays. A great disagreement between RT-PCR and IC results may be because of poor sensitivity of the IC assays [Citation95]. Li et al. attributed false-negative results of POC LFIA to low concentrations of IgM and IgG levels, the difference in individual antibody production in COVID‐19 patients and the rapid disappearance of IgM antibody after 2 weeks [Citation86]. Due to the low sensitivity of IC assays during the early phase of infection, this method is not suggested for diagnosing COVID-19 patients in early stages of illness and cannot be changed with RT-PCR as an acute diagnostic protocol for COVID-19 [Citation96]. However, when RT-PCR is not available, IC assay can be combined with chest CT for diagnosing COVID-19. Imai et al. found that the diagnostic sensitivity of the CT scan is increased when it combined with IC assay [Citation96]. Spicuzza and colleagues revealed that in case of disagreement between clinical/radiological characteristic and molecular test results, the rapid POC antibody tests may help the clinician to make a correct diagnosis as long as they are not used within the first days of symptoms onset. However, the diagnostic and screening values of these tests should be confirmed in larger sample size [Citation97].
11.7. Comparison of LFIAs and ELISA/CLIAs
According to the need for rapid serological tests, it is necessary to evaluate them against panels of serologically positive or negative samples by ELISA, CLIA or ECLIA before their extensive use [Citation23]. Many studies demonstrated higher sensitivity for ELISA-based methodology compared to LFIAs. In the following section, studies demonstrating the better performance of ELISA/CLIA compared with LFIA devices are described (). Ong and colleagues demonstrated that specificities of LFIAs and ELISA were very high and the ELISA demonstrated higher sensitivity than LFIAs [Citation64]. In comparison of anti-SARS-CoV-2 IgG detection by two POC devices and one ELISA, Bond et al. revealed that the sensitivity of the ELISA was consistently higher than two POC devices, when samples are categorized by days post-symptom onset [Citation87]. Adams et al. concluded that the diagnostic performance of the accessible commercial LFIA devices is not well enough at the present time. However, sensitivity of the ELISA was high 10 days post-symptoms onset [Citation31]. In the study of Bartolini et al., positive samples by both RT-PCR and IC testing, were examined by ELISAs/CLIAs. Overall agreement percentage between ELISA/CLIA and KHB IC assay was 15% and between ELISA/CLIA and Cellex IC assay was 43%. The contradiction between immunoglobulins positivity patterns detected by IC and ELISA/CLIA tests emphasized the need of assays validation before their marketing and application to prevent errors at both clinical and epidemiological level [Citation95].
Table 3. Comparison of COVID-19 LFIAs and ELISA/CLIAs
It was found that the positivity of LFIAs is increased over time from symptom onset [Citation31]. In the study of Tuaillon et al., regardless of the used test (ELISA assays or POC tests), the rate of detection was enhanced to over 80%, but never reached 100%, 15 days after the onset of symptoms [Citation67]. However, all seven IgG/IgM LFIAs examined in the study of Van Elslande et al. demonstrated sensitivity of >92% for detecting IgG antibodies 14–25 days after the onset of the symptom compared to 89.5% for the IgG ELISA [Citation26]. In the study of Lu e al., one CLIA and two LFIAs were validated. The disagreement cases were samples collected during the first 2 weeks of symptoms. As performing the tests was commonly not advisable before two weeks post symptom onset, the difference between assay formats would not considered as clinically significant [Citation98]. In the study of Lassaunière et al., diagnostic performances of 6 different LFIAs was evaluated and compared to ELISA (Wantai SARS-CoV-2 Ab). The highest sensitivity was achieved in late phase of disease (14 to 20 days after the onset of disease symptoms) compared to the early and middle phase. Wantai Total Ab ELISA and AutoBio Diagnostics POC test demonstrated the highest concordance between an ELISA and POC test (93%). However, they showed that although some ELISA assays and POC tests may demonstrate comparable sensitivities and specificities, their results may not necessarily correlate with each other for a single patient sample [Citation12]. In another study, a head-to-head comparison of 10 LFIAs and 2 ELISAs in terms of performance by time from symptom onset and disease severity was performed. The highest percent of seropositivity was reported when samples were taken>20 days after symptom onset. The sensitivity of all examined assays in hospitalized patients three or more weeks into their disease course was good to excellent. However, COVID-19 diagnosis is still based on viral detection methods at time of symptom onset. Moreover, a direct correlation between LFIA band intensity scores and ELISA signal to cutoff (S/CO) values was reported. In case of considering weak LFIA bands as negative, specificity was enhanced and sensitivity was decreased [Citation99]. Overall, most samples were negative during the first week of COVID-19 and they were positive after the second week of COVID-19 irrespective of the used test. Therefore, good overall concordance between LFIAs and ELISAs was reported during the first week and after the second week of COVID-19 illness [Citation67].
11.8. Comparison of ELISA, CLIA, and LFIAs
The performance of different tests was highly related to the time of sampling. In the study of Pickering et al., a highly specific in-house ELISA for the detection of anti- S, – RBD and – N antibodies was developed and compared with a CLIA-based platform, two ELISAs and seven LFIAs on identical sample sets. The highest sensitivity, with narrowest confidence limits, for all platforms was reported in samples taken 20 days post-onset of symptoms [Citation20]. In the study of Nicol et al., 293 specimens were evaluated by two automated immunoassays (ELISA and CLIA-based methods) and one LFIA. All the examined assays had 100% sensitivity for IgG detection >14 days after onset of illness. However, the overall specificity for IgG detection was higher for CLIA and LFIA compared to ELISA. Furthermore, the best agreement for IgG detection respective to days from symptom onset was seen between CLIA and LFIA assays [Citation100]. The superiority of Wantai ELISA for early detection of COVID-19 (within 10 days after symptom onset) [Citation57] and for detection of protective antibodies [Citation13] in comparison with LFIAs and CLIAs was reported by two studies (). In the study of GeurtsvanKessel et al., the diagnostic performance of 3 platforms including 3 rapid tests, 4 ELISAs, and a high throughput CLIA was evaluated in correlation to an in-house virus NT assay. Wantai SARS-CoV-2 total Ig ELISA demonstrated the best overall performance for detecting functional antibodies in different stages and degrees of severity. It was suggested that by defining of a cutoff in this assay, the presence of nAbs could be indicated [Citation13].
Table 4. Comparison of COVID-19 ELISA, CLIA and LFIAs
Furthermore, the diagnostic performance of seven commercially available SARS-CoV-2 serology assays based on ELISA and CLIA as well as rapid tests were comparatively analyzed. Only Wantai SARS-COV-2 Ab ELISA demonstrated acceptable sensitivity during 10 days after symptom onset and could obtain medically relevant diagnostic power. However, both Wantai SARS-COV-2 Ab ELISA and Elecsys Anti-SARS-CoV-2 assays are proper for sensitive and specific screening of SARS-CoV-2 antibodies from 10 days after symptom onset [Citation57].
12. Antigen-based SARS-CoV-2 LFIAs
Because of long gap between infection and seroconversion, none of the developed serology LFIAs could be applied for diagnostic use. Based on the results of studies on SARS-CoV-1, it was assumed that antigen-based SARS-CoV-2 assays might have superior clinical sensitivity than serology assays. The sensitivity of antigen-based LFIAs is less than RT-PCR; however, further research and development are needed to enhance the clinical sensitivity of antigen-based LFIAs [Citation81]. It is necessary to determine the best antigen target for antigen detecting SARS-CoV-2 LFIAs, which provides the greatest sensitivity. Initial studies have been focused on N protein. However, S and M proteins of SARS-CoV-2 should also be evaluated. Furthermore, targeting two different antigens one for efficient capturing and the other one for the most sensitive detection can be applied to directly detect intact SARS-CoV-2 virions. Therefore, novel capture and detector antibodies pairs should be screened [Citation81].
12.1. Examples of antigen-based LFIAs
COVID-19 Ag Respi-Strip is a rapid and ready-to-use assay for the qualitative detection of SARS-CoV-2 antigen in nasopharyngeal secretions. The ICT strip contains nitrocellulose laminated on a plastic backing as well as conjugate pad with colloidal-gold conjugates overlapping the bottom of the nitrocellulose. The highly conserved SARS-CoV and SARS-CoV-2 N antigen are detected by colloidal gold nanoparticles labeled anti-SARS-CoV-2 antibody migrating with the buffer and the sample. Then, the complexes are recognized by anti-SARS-CoV capture antibody, which are immobilized onto the nitrocellulose membrane. The correct migration of the sample is examined by a control line in the strip. The result can be visually interpreted after 15 min [Citation101,Citation102].
In the study of Grant et al., a half-strip LFIA for detection of N protein of SARS-CoV-2 using commercially existing antibodies was developed. A half-strip (dipstick) LFIA contains only a nitrocellulose analytical membrane and a wick pad. Sample and conjugate pads do not exist in a half-strip LFIA. Sample and conjugate are mixed in a container, such as a 96-well plate, before using a half-strip. If the LOD is near clinically useful ranges, sample and conjugate pad will be added directly to the strip and consequently can be used as a POC test. In this half-strip LFIA, LOD of 0.62 ng/mL was measured using an optical reader [Citation81].
12.2. Comparison of Antigen-based LFIAs and RT-PCR
In the study of Blairon et al., the median positive percentage agreement between Respi-Strip and RT-PCR is fair (23.9%) [Citation103]. In addition, Scohy et al. demonstrated that among 106 positive RT-qPCR confirmed COVID-19 samples, only 32 were diagnosed by the Respi-Strip test resulting in an overall sensitivity of 30.2%. Better antigen detection rates by Respi-Strip kit could be obtained in higher viral loads [Citation101].
It was found that the number of samples outsourced for COVID-19 confirmation by RT-PCR and costs per patient were not significantly decreased by Respi-Strip [Citation103]. Furthermore, COVID-19 Ag Respi-Strip demonstrated poor diagnostic sensitivity compared to gold standard molecular assays such as RT-PCR. Therefore, despite good specificity of 99.5% and rapid performance of Respi-Strip [Citation102], its application as the only frontline testing for COVID-19 diagnosis is not permitted [Citation101]. In contrast, the antigen-based immunofluorescence RDT developed by Porte et al., demonstrated high sensitivity and specificity in respiratory samples mainly obtained during the first week of COVID-19 symptoms and with high viral loads. All false-negative RDT results were related to the samples with RT-PCR cycle threshold (Ct) values >26 indicating lower viral loads. This antigen-based RDTs can be used for early diagnosis of SARS-CoV-2 in case of little availability to molecular methods [Citation104]. Furthermore, four antigen-based SARS-CoV-2 antigen detection tests were compared to RT-PCR. Significant performance heterogeneity was reported among the examined kits. It was demonstrated that patients with high viral loads during early and infective stages of SARS-CoV-2 infection can be detected by screening of respiratory specimens using antigen-based RDTs [Citation105].
13. Red blood cell Agglutination Assay
Kruse et al. constructed a fusion protein consisting of RBD of the SARS-CoV-2 and a single-chain variable fragment (scFv) against red blood cell (RBC) antigen (). The mixture of the fusion protein (RBD-scFv), RBCs and recovered COVID-19 patient serum caused hemagglutination or aggregation of RBCs indicating the development of antibodies against SARS-CoV-2 RBD. The readout of the assay can be visually observed or taken by a camera. Therefore, a rapid POC RBC agglutination assay has been developed to detect antibodies against SARS-CoV-2 within 5 min incubation of RBC, RBD-scFv and anti- SARS-CoV-2 antibodies. This cheap and scalable method can be applied especially in low-resource settings [Citation106]. In another study, a bioconjugate containing anti-D-IgG and peptides from SARS-CoV-2 spike protein was produced and immobilized to reagent red blood cells (RRBCs). Then, selective agglutination was observed in the presence of plasma collected from patients recently infected with SARS-CoV-2. Agreement between the results of the developed gel card assays and an indirect IgG ELISA was reported among 10 clinical samples, five of which were PCR-confirmed COVID-19 samples. This simple, rapid, scalable platform can be evaluated in a large sample cohort before scaled-up application and distribution [Citation107].
Figure 6. Mechanism of red blood cell agglutination assay
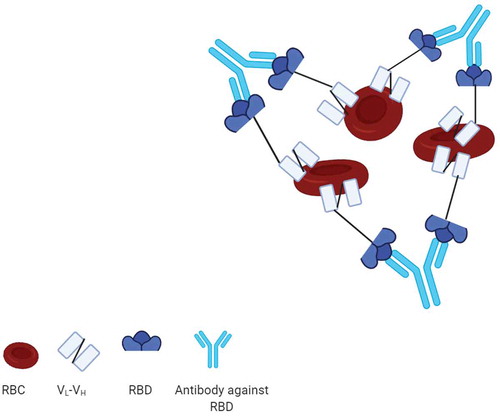
14. Immunofluorescence assay (IFA)
In IFA, a specific target antigen is labeled with an antibody conjugated to a fluorophore and the targets are visualized under a fluorescent microscope. In Direct IFAs, the primary antibody against the target of interest is directly conjugated to a fluorophore. However, in indirect IFAs primary antibody interacts with the target antigen and the antibody directed against the primary antibody is fluorophore-conjugated. Meschi et al. established an indirect IFA. They used household slides prepared with Vero E6 cells infected with SARS-CoV-2 isolate in the BSL3 facility [Citation74]. In the study of Andrey et al., Vero B4 cells transfected with the SARS-CoV-2 spike protein expression vector were seeded on glass slides [Citation105]. In the study of Kohmer et al., after incubation of the prepared slides and diluted patients sample, goat anti‐human fluorescein‐labeled IgG conjugate was used as a secondary antibody and the microscopic analysis was performed [Citation48].
14.1. Comparison of IFAs with Other Serological Assays
In the study of Meschi et al., Abbott SARS-CoV-2 IgG immunoassay was compared to IFA. IgG targeted to SARSCoV-2 N antigen was detected in all patients by Abbott two-week post-symptoms. As whole virus antigens were used for IFA, the sensitivity of IFA was higher than Abbott. However, IFA demonstrated reduced specificity [Citation74]. The performance of two ELISA assays, one LFIA and two in‐house developed assays (IFA and PRNT) was compared for IgG detection in RT-PCR diagnosed COVID-19 patients. The sensitivity of all of the evaluated assays were enhanced in the later phase of infection (≥10 days) compared to the early phase of infection (days 5‐9). The highest sensitivity in the evaluated assays was related to the Vircell ELISA, IFA, and PRNT. It was found that all detected SARS‐CoV‐2 IgG antibodies (with the exception of one sample) using the commercially available assays are potentially protective and showed neutralizing properties in the PRNT [Citation48].
15. Conclusion
Currently, the fundamental methodology for in vitro diagnosis of SARS-CoV-2 infection is based on NAAT. It is not possible to properly diagnose acute disease by IgG testing. However, serology test is useful for retrospective identification of COVID-19 patients and for contact tracing purposes, when doubt of false-positive or false-negative PCR results has been existed [Citation59].
Since serology tests reached their highest positive rates 14 days after symptom onset and the sensitivity of RT-PCR was decreased at this time, serology testing could be considered as a part of diagnostic panel two-week post-symptom onset [Citation98]. Furthermore, it is necessary to determine the optimal algorithm for performing COVID-19 serological tests especially in low-prevalence settings [Citation46].
Additionally, regardless of the examined method, higher sensitivity is achieved by determining combined IgG/IgM titers rather than measuring either antibody type alone [Citation7,Citation108]. It was also demonstrated that the targeted SARS-CoV-2 antigen and the assay format greatly affect the accuracy and reliability of serological methods [Citation37]. Overall, higher sensitive serological test detecting NT antibody could be developed by ELISA/CLIA-based methods targeting S antigen.
16. Expert opinion
It is still unclear whether antibodies can serve as an indicator for the presence of protective or sustained immunity or not [Citation66]. In case of correlation between IgG testing and immunity to subsequent infections, serology testing would be advantageous in vaccine development, clinical trials, and epidemiological studies [Citation59]. Additionally, due to the involvement of many manufacturers in the market, serology tests can be scaled up for millions of people per day, which may assist in improving the detection of the virus in health care settings, especially in limited resources countries [Citation109]. Therefore, the development of serological assays with high sensitivity for screening and enough specificity to prevent unessential interventions is urgently required [Citation44]. However, to obtain adequate sensitivity and specificity, it is recommended to combine clinical, molecular, and serological diagnostic tests [Citation109].
Many antibody tests for the detection of SARS-CoV-2 infection are available with little validation on clinical samples across different populations [Citation12,Citation13]. In the systematic review conducted by Ghaffari et al., the performance of SARS-CoV-2 serological tests including 5 serological CLIA tests, 15 serological ELISA tests, and 42 serological RDTs currently on the market was reviewed. It was found that there are disagreements between the claimed and real performance of serological diagnostic tests on the market. Furthermore, the degree of variability in test sensitivity values was higher than specificity [Citation110]. It was also demonstrated that the diagnostic performance of COVID-19 serological assays is lower than those reported by manufacturers [Citation46]. These findings emphasized the need for independent evaluations of commercialized serological COVID-19 diagnostic tests [Citation1]. It was found that the optimal diagnostic thresholds of kits and the recommended cutoff value are incompatible with each other. Therefore, when using a novel coronavirus kit to decrease the missed diagnosis and misdiagnosis, it is necessary to evaluate performances and other necessary testing requirements of a kit and then results should be carefully interpreted [Citation55]. Additionally, the overall inter-assay agreement can be enhanced by optimizing thresholds; therefore, serologic tests can be better harmonized [Citation58]. However, because of the development of baseline antibodies against SARS-CoV-2 in a larger percentage of patients, the analysis of IgG testing may change in the future [Citation59].
Improper sampling time [Citation111], limited sample size [Citation44] and short follow-up [Citation112] are the common limitations of different studies evaluating serological tests for COVID-19. It was found that sensitivity and specificity of different immunoassays are affected by different variables including patient groups, time of sample acquisition in terms of days post-symptom onset, sample size, and inclusion of relevant control sera from patients with non–SARS-CoV-2 respiratory infections. Therefore, it is very crucial to consider these variables in case of performing the validation study and interpretation of different studies results [Citation1]. For example, patients with other human coronavirus infections should be involved in validation studies of serological assays to assess specificity [Citation46]. Due to higher IgG and IgM levels in blood in the later stages of the disease, serological tests are more effective in later stages (two-week post-symptom onset) [Citation110]. Application of serology testing before two-week post-symptom onset should be determined on a case-by-case basis [Citation98]. Lisboa et al. also revealed in their systematic review that the sensitivity of serological tests for COVID-19 is higher at least three weeks after symptom onset compared with within the first week [Citation113].
It was reported that IgG tests are more sensitive than IgM ones when the samples were taken later after the beginning of symptoms [Citation108]. Furthermore, a high rate of false-negative results ranged from 10 to 44% was reported for SARS-CoV-2 IgM-detecting tests, which are used to detect COVID-19 in the acute phase [Citation114]. Additionally, a systematic and meta-analysis review conducted by Vengesai et al. revealed that the best overall diagnostic test accuracy is obtained by IgG-IgM-based ELISA [Citation7]. Böger et al. also revealed in their systematic review that higher sensitivity, specificity and better negative likelihood ratio (NLR) are achieved when total antibodies are measured [Citation109].
It was also demonstrated that the targeted SARS-CoV-2 antigen and assay methodology have influences on the diagnostic performance of serological methods [Citation37]. It was shown that antibodies targeting different domains of S protein, including S1, RBD and S2, may involve in virus neutralization [Citation58]. Kontou et al. demonstrated that the sensitivity and specificity of S antigen-based tests are higher than N antigen-based ELISA tests may be due to faster immune response to the S antigen and lower cross-reactivity with less conserved regions of spike proteins existing in other coronaviruses (SARS-CoV), respectively [Citation108]. In the systematic review and meta-analysis by Lisboa and colleagues, pooled sensitivity of CLIAs, ELISAs measuring IgG or IgM and LFIAs was 97.8, 84.3, and 66.0%, respectively [Citation113]. Kontou et al. also revealed that the sensitivity of ELISA- and CLIA-based methods are superior followed by LFIA and FIA [Citation108]. As reported by La Marca and colleagues, currently, none of the POC diagnostic SARS-CoV-2 tests is appropriate for wide-scale deployment [Citation44]. The findings of Lisboa et al. are against and do not support the continued use of existing LFIA tests for COVID-19 diagnosis [Citation113]. According to the weak performances of the current LFIA devices, it is required to develop highly sensitive and specific POC LFIAs for application in individual patient and large seroprevalence studies [Citation7].
Furthermore, it is necessary to validate the quality of rapid tests detecting SARS-CoV-2 antibodies by third parties before their clinical applications [Citation95]. In a meta-analysis conducted by Caini et al., six studies based on commercial ELISA or CMIA/CLIA assays and three studies based on in-house tests were included. A summary sensitivity of 82% for IgM, and 85% for both IgG and total antibodies were reported by this meta-analysis. Due to unsatisfactory pooled sensitivity for all types of antibodies, a considerable fraction of seroconverted individuals would be incorrectly considered as non-seroconverted. Caini et al. concluded that currently the use of serological tests for large-scale prevalence surveys are only legitimatized in hard-hit regions [Citation115].
Kontou et al., in their meta-analysis, revealed that all ELISA, CLIA, FIA, and LFIA methods are highly specific [Citation108]. ELISA and LFIA attained specificity levels higher than 99%. As stated by Caini et al., specificity is generally very high for most tests, and pooled estimate was 98% for IgM and 99% for both IgG and total antibodies [Citation115]. Lisboa and colleagues also demonstrated that pooled specificities for each test method are high (ranged from 96.6% to 99.7%). However, especially for LFIAs, other viral infections might provide lower specificity resulting in false-positive [Citation113]. Overall, it should be taken into account that specificity just less than 99% in populations with a low prevalence of (≤5%) means that approximately one-fifth of seroconverted individuals would be false positives [Citation115].
Article highlights
It is required to rapidly develop COVID-19 serological assays with high sensitivities for screening and enough specificities to prevent non-essential interventions.
Due to disagreements between the claimed and real performance of serological diagnostic tests on the market, validation of commercialized serological COVID-19 diagnostic tests across different populations are greatly needed.
Because of higher IgG and IgM levels in blood in the later stages of the disease, serological tests are more effective in later stages.
To achieve higher sensitivity, determining combined IgG/IgM titers rather than measuring either antibody type alone is suggested regardless of the examined method.
Serological test with higher sensitivity, which detects neutralization antibody can be developed by ELISA/CLIA-based methods targeting spike (S) antigen.
Reviewer disclosures
Peer reviewers on this manuscript have no relevant financial or other relationships to disclose.
Declaration of interest
The authors have no relevant affiliations or financial involvement with any organization or entity with a financial interest in or financial conflict with the subject matter or materials discussed in the manuscript. This includes employment, consultancies, honoraria, stock ownership or options, expert testimony, grants or patents received or pending, or royalties.
Acknowledgments
The figures were drawn using BioRender (https://biorender.com/)
Additional information
Funding
- Espejo AP, Akgun Y, Al Mana AF, et al. Review of current advances in serologic testing for COVID-19. Am J Clin Pathol. 2020;154(3):293–304.
- Kubina R, Dziedzic A. Molecularand serological tests for COVID-19 a comparative review of SARS-CoV-2 coronavirus laboratory and point-of-care diagnostics. Diagnostics. 2020;10(6):434.
- Jamaati H, Dastan F, Tabarsi P, et al. A fourteen-day experience with coronavirus disease 2019 (COVID-19) induced Acute Respiratory Distress Syndrome (ARDS): an Iranian treatment protocol. Iranian Journal of Pharmaceutical Research. 2020;19(1):31–36.
- Lee CY-P, Lin RT, Renia L, et al. Serological Approaches for COVID-19: epidemiologic perspective on surveillance and control. Front Immunol. 2020;11:879.
- Dastan F, Saffaei A, Haseli S, et al. Promising Effects of Tocilizumab in COVID-19: a non-controlled, prospective clinical trial. Int Immunopharmacol. 2020;88:106869.
- Organization WH. Laboratory testing for coronavirus disease 2019 (COVID-19) in suspected human cases: interim guidance, 2 March 2020. World Health Organization; 2020.
- Vengesai A, Midzi H, Kasambala M, et al. A systematic and meta-analysis review on the diagnostic accuracy of antibodies in the serological diagnosis of COVID-19. 2020. DOI: 10.21203/rs.3.rs-34638/v2.
- Cai XF, Chen J, Hu JL, et al. A peptide-based magnetic chemiluminescence enzyme immunoassay for serological diagnosis of coronavirus disease 2019. J Infect Dis. 2020;222(2):189–195.
- Wolff F, Dahma H, Duterme C, et al. Monitoring antibody response following SARS-CoV-2 infection: diagnostic efficiency of 4 automated immunoassays. Diagn Microbiol Infect Dis. 2020;98(3):115140.
- To KKW, Tsang OTY, Leung WS, et al. Temporal profiles of viral load in posterior oropharyngeal saliva samples and serum antibody responses during infection by SARS-CoV-2: an observational cohort study [Article]. Lancet Infect Dis. 2020;20(5):565–574.
- Wang H, Ai J, Loeffelholz MJ, et al. Meta-analysis of diagnostic performance of serology tests for COVID-19: impact of assay design and post-symptom-onset intervals. Emerg Microbes Infect. 2020;9(1):2200–2211.
- Lassaunière R, Frische A, Harboe ZB, et al. Evaluation of nine commercial SARS-CoV-2 immunoassays. Medrxiv. 2020. Available from: https://doi.org/10.1101/2020.04.09.20056325
- GeurtsvanKessel CH, Okba NM, Igloi Z, et al. An evaluation of COVID-19 serological assays informs future diagnostics and exposure assessment. Nat Commun. 2020;11(1):1–5.
- Rastawicki W, Rokosz-Chudziak N. Characteristics and assessment of the usefulness of serological tests in the diagnostic of infections caused by coronavirus SARS-CoV-2 on the basis of available manufacturer’s data and literature review. Przegl Epidem. 2020;74(1):49–68.
- Ravi N, Cortade DL, Ng E, et al. Diagnostics for SARS-CoV-2 detection: a comprehensive review of the FDA-EUA COVID-19 testing landscape. Biosens Bioelectron. 2020;165:112454.
- Xu M, Wang D, Wang H, et al. COVID‐19 diagnostic testing: technology perspective. Clin Transl Med. 2020;10(4):e158.
- Zhou Q, Zhu D, Yan H, et al. A preliminary study on analytical performance of serological assay for SARS-CoV-2 IgM/IgG and application in clinical practice. medRxiv. 2020. Available from: https://doi.org/10.1101/2020.05.05.20092551
- Yongchen Z, Shen H, Wang X, et al. Different longitudinal patterns of nucleic acid and serology testing results based on disease severity of COVID-19 patients. Emerg Microbes Infect. 2020;9(1):833–836.
- Wu J-L, Tseng W-P, Lin C-H, et al. Four point-of-care lateral flow immunoassays for diagnosis of COVID-19 and for assessing dynamics of antibody responses to SARS-CoV-2. J Infect. 2020;81(3):435-442.
- Pickering S, Betancor G, Galão RP, et al., Comparative assessment of multiple COVID-19 serological technologies supports continued evaluation of point-of-care lateral flow assays in hospital and community healthcare settings. PLoS Pathog. 2020;16(9): e1008817.
- Zhao J, Yuan Q, Wang H, et al. Antibody Responses to SARS-CoV-2 in patients with novel coronavirus disease 2019. Clin Infect Dis. 2020;71(16):2027–2034.
- Ma H, Zeng W, He H, et al. COVID-19 diagnosis and study of serum SARS-CoV-2 specific IgA, IgM and IgG by chemiluminescence immunoanalysis. medRxiv. 2020. Available from: https://doi.org/10.1101/2020.04.17.20064907
- Saenz-Flor KV, Santafe LM. Concordance of” rapid” serological tests and IgG and IgM chemiluminescence for SARS-COV-2. medRxiv. 2020. Available from: https://doi.org/10.1101/2020.06.01.20114884
- Perera RA, Mok CK, Tsang OT, et al. Serological assays for severe acute respiratory syndrome coronavirus 2 (SARS-CoV-2), March 2020. Eurosurveillance. 2020;25(16):2000421.
- Adams ER, Anand R, Andersson MI, et al. Evaluation of antibody testing for SARS-Cov-2 using ELISA and lateral flow immunoassays. Wellcome Open Res. 2020;5:139..
- Van Elslande J, Houben E, Depypere M, et al. Diagnostic performance of seven rapid IgG/IgM antibody tests and the Euroimmun IgA/IgG ELISA in COVID-19 patients. Clin Microbiol Infect. 2020;26(8):1082–1087.
- Long Q-X, Liu B-Z, Deng H-J, et al. Antibody responses to SARS-CoV-2 in patients with COVID-19. Nat Med. 2020;;26(6):845-848.
- Coste AT, Jaton K, Papadimitriou-Olivgeris M, et al. Comparison of SARS-CoV-2 serological tests with different antigen targets. J Clin Virol. 2021;134:104690.
- Lou B, Li TD, Zheng SF, et al. Serology characteristics of SARS-CoV-2 infection after exposure and post-symptom onset. Eur Respir J. 2020;56(2):2000763.
- Liu W, Liu L, Kou G, et al. Evaluation of nucleocapsid and spike protein-based enzyme-linked immunosorbent assays for detecting antibodies against SARS-CoV-2. J Clin Microbiol. 2020;58(6):e00461-20.
- Adams ER, Ainsworth M, Anand R, et al. Antibody testing for COVID-19: a report from the national COVID scientific advisory panel. Wellcome Open Research. 2020;5( 139):139.
- Xia N, Wang G, Gong W. Serological test is an efficient supplement of RNA detection for confirmation of SARS-CoV-2 infection. 2020. DOI:10.20944/preprints202003.0184.v1.
- Torres R, Rinder HM. Double-edged spike-are SARS-CoV-2 serologic tests safe right now? Lab Med. 2020;51(3):236–238.
- Wang X, Guo X, Xin Q, et al. Neutralizing Antibodies Responses to SARS-CoV-2 in COVID-19 Inpatients and Convalescent Patients. Clin Infect Dis. 2020;71(10):2688-2694. .
- Ko J-H, Joo E-J, Park S-J, et al. Neutralizing antibody production in asymptomatic and mild COVID-19 patients, in comparison with pneumonic COVID-19 patients. J Clin Med. 2020;9(7):2268.
- Zhang P, Gao Q, Wang T, et al. Evaluation of recombinant nucleocapsid and spike proteins for serological diagnosis of novel coronavirus disease 2019 (COVID-19). MedRxiv. 2020. Available from: https://doi.org/10.1101/2020.05.31.20118448.
- Padoan A, Sciacovelli L, Basso D, et al. IgA-Ab response to spike glycoprotein of SARS-CoV-2 in patients with COVID-19: a longitudinal study [Article]. Clin Chim Acta. 2020;507:164–166.
- Brouwer PJM, Caniels TG, Van Der Straten K, et al. Potent neutralizing antibodies from COVID-19 patients define multiple targets of vulnerability. Science. 2020;369(6504):643–650.
- Bonelli F, Sarasini A, Zierold C, et al. Clinical and analytical performance of an automated serological test that identifies S1/S2-Neutralizing IgG in COVID-19 patients semiquantitatively. J Clin Microbiol. 2020;58(9):e01224–20.
- GeurtsvanKessel CH, Okba NM, Igloi Z, et al. Towards the next phase: evaluation of serological assays for diagnostics and exposure assessment. medRxiv. 2020. Available from: https://doi.org/10.1101/2020.04.23.20077156
- Burbelo PD, Riedo FX, Morishima C, et al. Sensitivity in detection of antibodies to nucleocapsid and spike proteins of severe acute respiratory syndrome coronavirus 2 in patients with coronavirus disease 2019. J Infect Dis. 2020;222(2):206–213.
- Schnurra C, Reiners N, Biemann R, et al. Comparison of the diagnostic sensitivity of SARS-CoV-2 nucleoprotein and glycoprotein-based antibody tests. J Clin Virol. 2020;129:104544.
- Manski CF. Bounding the predictive values of COVID-19 antibody tests. Epidemiology. 2021;32(2):162-167.
- La Marca A, Capuzzo M, Paglia T, et al. Testing for SARS-CoV-2 (COVID-19): a systematic review and clinical guide to molecular and serological in-vitro diagnostic assays. Reprod Biomed Online. 2020;41(3):483–499.
- Kumleben N, Bhopal R, Czypionka T, et al. Test, test, test for COVID-19 antibodies: the importance of sensitivity, specificity and predictive powers. Public Health. 2020;185:88–90.
- Bond K, Nicholson S, Lim SM, et al. Evaluation of Serological Tests for SARS-CoV-2: implications for Serology Testing in a Low-Prevalence Setting. J Infect Dis. 2020;222(8):1280–1288.
- Wang S, Sakhatskyy P, Chou T-HW, et al. Assays for the assessment of neutralizing antibody activities against Severe Acute Respiratory Syndrome (SARS) associated coronavirus (SCV). J Immunol Methods. 2005;301(1–2):21–30.
- Kohmer N, Westhaus S, Rühl C, et al. Clinical performance of different SARS-CoV-2 IgG antibody tests. J Med Virol. 2020 Oct;92(10):2243–2247.
- Algaissi A, Hashem AM. Evaluation of MERS-CoV neutralizing antibodies in sera using live virus microneutralization assay. Methods Mol Biol. 2020;2099:107–116.
- Amanat F, White KM, Miorin L, et al. An In Vitro Microneutralization Assay for SARS‐CoV‐2 Serology and Drug Screening. Curr Protoc Microbiol. 2020;58(1):e108.
- Muruato AE, Fontes-Garfias CR, Ren P, et al. A high-throughput neutralizing antibody assay for COVID-19 diagnosis and vaccine evaluation. Nat Commun. 2020;11(1).
- Nie J, Li Q, Wu J, et al. Establishment and validation of a pseudovirus neutralization assay for SARS-CoV-2. Emerg Microbes Infect. 2020;9(1):680–686.
- Tan CW, Chia WN, Qin X, et al. A SARS-CoV-2 surrogate virus neutralization test based on antibody-mediated blockage of ACE2-spike protein-protein interaction. Nat Biotechnol. 2020;38(9):1073–1078.
- Cinquanta L, Fontana DE, Bizzaro N. Chemiluminescent immunoassay technology: what does it change in autoantibody detection? Autoimmunity Highlights. 2017;8(1):9.
- Wan Y, Li Z, Wang K, et al. Performance verification of anti-SARS-CoV-2-specific antibody detection by using four chemiluminescence immunoassay systems. Ann Clin Biochem. 2020;57(6):429–434.
- Russo A, Minichini C, Starace M, et al. Current status of laboratory Diagnosis for COVID-19: a narrative review. Infect Drug Resist. 2020;13:2657.
- Herroelen PH, Martens GA, De Smet D, et al. Kinetics of the humoral immune response to SARS-CoV-2: comparative analytical performance of seven commercial serology tests. medRxiv. 2020. Available from: https://doi.org/10.1101/2020.06.09.20124719
- Plebani M, Padoan A, Negrini D, et al. Diagnostic performances and thresholds: the key to harmonization in serological SARS-CoV-2 assays? Clin Chim Acta. 2020;509:1–7.
- Chew KL, Tan SS, Saw S, et al. Clinical evaluation of serological IgG antibody response on the Abbott Architect for established SARS-CoV-2 infection. Clin Microbiol Infect. 2020;26(9):1256.e9-1256.e11.
- Bryan A, Pepper G, Wener MH, et al. Performance characteristics of the abbott architect SARS-CoV-2 IgG assay and seroprevalence in Boise, Idaho. J Clin Microbiol. 2020 ;58(8) :e00941-20.
- Nakano Y, Kurano M, Morita Y, et al. Time course of the sensitivity and specificity of serum anti-SARS-CoV-2 IgM and IgG antibodies for the diagnosis of symptomatic COVID-19 in Japan.Sci Rep. 2021;11(1):2776.
- Lippi G, Salvagno GL, Pegoraro M, et al. Assessment of Immune Response to SARS-CoV-2 With Fully Automated MAGLUMI 2019-nCoV IgG and IgM Chemiluminescence Immunoassays. Clin Chem Lab Med. 2020;58(7):1156–1159.
- Favresse J, Eucher C, Elsen M, et al. Clinical Performance of the Elecsys Electrochemiluminescent Immunoassay for the detection of SARS-CoV-2 Total Antibodies. Clin Chem. 2020;66(8):1104–1106.
- Ong DSY, De Man SJ, Lindeboom FA, et al. Comparison of diagnostic accuracies of rapid serological tests and ELISA to molecular diagnostics in patients with suspected coronavirus disease 2019 presenting to the hospital. Clin Microbiol Infect. 2020;26(8):1094.e7-1094.e10.
- Marlet J, Petillon C, Ragot E, et al. Clinical performance of four immunoassays for antibodies to SARS-CoV-2, including a prospective analysis for the diagnosis of COVID-19 in a real-life routine care setting. J Clin Virol. 2020;132:104633.
- Beavis KG, Matushek SM, Abeleda APF, et al. Evaluation of the EUROIMMUN Anti-SARS-CoV-2 ELISA Assay for detection of IgA and IgG antibodies. J Clin Virol. 2020;129:104468.
- Tuaillon E, Bolloré K, Pisoni A, et al. Detection of SARS-CoV-2 antibodies using commercial assays and seroconversion patterns in hospitalized patients. J Infect. 2020;81(2):e39–e45.
- Amanat F, Stadlbauer D, Strohmeier S, et al. A serological assay to detect SARS-CoV-2 seroconversion in humans. Nat Med. 2020;26(7):1033-1036.
- Ni L, Ye F, Cheng ML, et al. Detection of SARS-CoV-2-Specific Humoral and Cellular Immunity in COVID-19 Convalescent Individuals. Immunity. 2020 Jun 16;52(6):971–977.e3.
- Peterhoff D, Glück V, Vogel M, et al. A highly specific and sensitive serological assay detects SARS-CoV-2 antibody levels in COVID-19 patients that correlate with neutralization. Infection. 1–8.
- Weidner L, Gänsdorfer S, Unterweger S, et al. Quantification of SARS-CoV-2 antibodies with eight commercially available immunoassays. J Clin Virol. 2020;129:104540.
- Patel EU, Bloch EM, Clarke W, et al. Comparative performance of five commercially available serologic assays to detect antibodies to SARS-CoV-2 and identify individuals with high neutralizing titers. J Clin Microbiol. 2021;59(2):e02257-20.
- Padoan A, Bonfante F, Pagliari M, et al. Analytical and clinical performances of five immunoassays for the detection of SARS-CoV-2 antibodies in comparison with neutralization activity. EBioMedicine. 2020;62:103101.
- Meschi S, Colavita F, Bordi L, et al. Performance evaluation of Abbott ARCHITECT SARS-CoV-2 IgG immunoassay in comparison with indirect immunofluorescence and virus microneutralization test. J Clin Virol. 2020;129:104539.
- Pflüger LS, Bannasch JH, Brehm TT, et al. Clinical evaluation of five different automated SARS-CoV-2 serology assays in a cohort of hospitalized COVID-19 patients. J Clin Virol. 2020;130:104549.
- Trabaud MA, Icard V, Milon MP, et al. Comparison of eight commercial, high-throughput, automated or ELISA assays detecting SARS-CoV-2 IgG or total antibody. J Clin Virol. 2020;132:104613.
- Zhong L, Chuan J, Gong B, et al. Detection of serum IgM and IgG for COVID-19 diagnosis. Sci China Life Sci. 2020 ;63(5):777–780.
- Lin D, Liu L, Zhang M, et al. Evaluations of the serological test in the diagnosis of 2019 novel coronavirus (SARS-CoV-2) infections during the COVID-19 outbreak. Eur J Clin Microbiol Infect Dis . 2020;39(12):2271–2277.
- Egger M, Bundschuh C, Wiesinger K, et al. Comparison of the Elecsys® Anti-SARS-CoV-2 immunoassay with the EDI™ enzyme linked immunosorbent assays for the detection of SARS-CoV-2 antibodies in human plasma. Clin Chim Acta. 2020;509:18–21.
- Tré-Hardy M, Wilmet A, Beukinga I, et al. Validation of a chemiluminescent assay for specific SARS-CoV-2 antibody. Clin Chem Lab Med. 2020 Jul 28;58(8):1357–1364.
- Grant BD, Anderson CE, Williford JR, et al. SARS-CoV-2 coronavirus nucleocapsid antigen-detecting half-strip lateral flow assay toward the development of point of care tests using commercially available reagents. Anal Chem. 2020;92(16):11305–11309.
- Koczula KM, Gallotta A. Lateral flow assays. Essays Biochem. 2016;60(1):111–120.
- El-Moamly A. Immunochromatographic Techniques: benefits for the diagnosis of parasitic infections. Austin Chromatogr. 2014;1(4):8.
- Cassaniti I, Novazzi F, Giardina F, et al. Performance of VivaDiag COVID-19 IgM/IgG Rapid Test is inadequate for diagnosis of COVID-19 in acute patients referring to emergency room department. J Med Virol. 2020;92(10):1724–1727.
- Pan Y, Li X, Yang G, et al. Serological immunochromatographic approach in diagnosis with SARS-CoV-2 infected COVID-19 patients. J Infect. 2020;81(1):e28–e32.
- Xie J, Ding C, Li J, et al. Characteristics of patients with coronavirus disease (COVID-19) confirmed using an IgM-IgG antibody test. J Med Virol. 2020;92(10):2004–2010.
- Bond K, Nicholson MS, Hoang MT, et al. Post-market validation of three serological assays for COVID-19. 2020.
- Prazuck T, Colin M, Giachè S, et al. Evaluation of performance of two SARS-CoV-2 Rapid IgM-IgG combined antibody tests on capillary whole blood samples from the fingertip. PloS One. 2020;15(9):e0237694.
- Demey B, Daher N, François C, et al. Dynamic profile for the detection of anti-SARS-CoV-2 antibodies using four immunochromatographic assays. J Infect. 2020;81(2):e6–e10.
- Lee YL, Liao CH, Liu PY, et al. Dynamics of anti-SARS-Cov-2 IgM and IgG antibodies among COVID-19 patients. J Infect. 2020 ;81(2):e55–e58.
- Fujigaki H, Takemura M, Osawa M, et al. Reliability of serological tests for COVID-19: comparison of three immunochromatography test kits for SARS-CoV-2 antibodies. Heliyon. 2020;6(9):e04929.
- Candel FJ, Viñuela-Prieto JM, González Del Castillo J, et al. Utility of lateral flow tests in SARS-CoV-2 infection monitorization. Revista espanola de quimioterapia: publicacion oficial de la Sociedad Espanola de Quimioterapia. 2020;33(4):258–266.
- Capello F, Cipolla M, Cosco L, et al. The VivaDiag COVID-19 lgM/IgG Rapid Test for the Screening and Early Diagnosis of COVID-19 in patients with No Clinical Signs of the Disease. Int J Endocrinol Metab Disord. 2020;6(1).
- Liu D, Ju C, Han C, et al. Ultra-sensitive nanozyme-based chemiluminescence paper test for rapid diagnosis of SARS-CoV-2 infection. bioRxiv. 2020. Available from:https://doi.org/10.1101/2020.06.05.131748
- Bartolini A, Scapaticci M, Bioli M, et al. Immunochromatographic assays for COVID-19 epidemiological screening: our experience. medRxiv. 2020. DOI: 10.1101/2020.05.28.20116046.
- Imai K, Tabata S, Ikeda M, et al. Clinical evaluation of an immunochromatographic IgM/IgG antibody assay and chest computed tomography for the diagnosis of COVID-19. J Clin Virol. 2020;128:104393.
- Spicuzza L, Montineri A, Manuele R, et al. Reliability and usefulness of a rapid IgM-IgG antibody test for the diagnosis of SARS-CoV-2 infection: a preliminary report. J Infect. 2020;81(2):e53–e54.
- Paiva KJ, Grisson RD, Chan PA, et al. Validation and performance comparison of three SARS-CoV-2 antibody assays. J Med Virol. 2021;93(2):916–923.
- Whitman JD, Hiatt J, Mowery CT, et al. Test performance evaluation of SARS-CoV-2 serological assays. medRxiv: the preprint server for health sciences. 2020;2020.04.25.20074856. Available from:http://doi.org/10.1101/2020.04.25.20074856
- Nicol T, Lefeuvre C, Serri O, et al. Assessment of SARS-CoV-2 serological tests for the diagnosis of COVID-19 through the evaluation of three immunoassays: two automated immunoassays (Euroimmun and Abbott) and one rapid lateral flow immunoassay (NG Biotech). J Clin Virol. 2020;129:104511.
- Scohy A, Anantharajah A, Bodéus M, et al. Low performance of rapid antigen detection test as frontline testing for COVID-19 diagnosis. J Clin Virol. 2020;129:104455.
- Mertens P, De Vos N, Martiny D, et al. Development and potential usefulness of the COVID-19 Ag Respi-Strip diagnostic assay in a pandemic context. Front Med (Lausanne). 2020;7:225.
- Blairon L, Wilmet A, Beukinga I, et al. Implementation of rapid SARS-CoV-2 antigenic testing in a laboratory without access to molecular methods: experiences of a general hospital. J Clin Virol. 2020;129:104472.
- Porte L, Legarraga P, Vollrath V, et al. Evaluation of a novel antigen-based rapid detection test for the diagnosis of SARS-CoV-2 in respiratory samples [Article]. Inter J Infect Dis. 2020;99:328–333.
- Andrey DO, Cohen P, Meyer B, et al. Head-to-head accuracy comparison of three commercial COVID-19 IgM/IgG serology rapid tests. J Clin Med. 2020;9(8):2369.
- Kruse RL, Huang Y, Smetana H, et al. A rapid, point of care red blood cell agglutination assay for detecting antibodies against SARS-CoV-2. bioRxiv. 2020.
- Alves D, Curvello R, Henderson E, et al. Rapid Gel Card agglutination assays for serological analysis following SARS-CoV-2 infection in humans. ACS Sens. 2020;5(8):2596–2603.
- Kontou PI, Braliou GG, Dimou NL, et al. Antibody tests in detecting SARS-CoV-2 infection: a meta-analysis. Diagnostics. 2020;10(5):319.
- Böger B, Fachi MM, Vilhena RO, et al. Systematic review with meta-analysis of the accuracy of diagnostic tests for COVID-19. Am J Infect Control. 2021;49(1):21–29.
- Ghaffari A, Meurant R, Ardakani ACOVID-19. Serological tests: how well do they actually perform? Diagnostics. 2020;10(7):453.
- Shi J, Han D, Zhang R, et al. Molecular and serological assays for SARS-CoV-2: insights from Genome and Clinical Characteristics. Clin Chem. 2020;66(8):1030–1046.
- Sood N, Simon P, Ebner P, et al. Seroprevalence of SARS-CoV-2-specific antibodies among adults in Los angeles county, California, on April 10-11, 2020. JAMA. 2020;323(23):2425–2427.
- Lisboa Bastos M, Tavaziva G, Abidi SK, et al. Diagnostic accuracy of serological tests for covid-19: systematic review and meta-analysis. BMJ. 2020;370:m2516.
- Castro R, Luz PM, Wakimoto MD, et al. COVID-19: a meta-analysis of diagnostic test accuracy of commercial assays registered in Brazil. Braz J Infect Dis. 2020;24(2):180–187.
- Caini S, Bellerba F, Corso F, et al. Meta-analysis of diagnostic performance of serological tests for SARS-CoV-2 antibodies up to 25 April 2020 and public health implications. Euro Surveill. 2020;25(23):2000980.