ABSTRACT
Introduction
Diabetes, chronic kidney disease (CKD) and cardiovascular disease (CVD) are cardiometabolic diseases that remain amongst the leading causes of morbidity and premature mortality. Here, we review the current understanding of how anti-inflammatory intervention via inhibition of the pro-inflammatory but pleiotropic cytokine interleukin (IL) 6 may benefit patients with these or related diseases or complications.
Areas covered
Based on a PubMed literature search, this review integrates and contextualizes evidence regarding the clinical utility of anti-IL-6 intervention in the treatment of cardiometabolic diseases, as well as of the associated condition nonalcoholic hepatosteatosis.
Expert opinion
Evidence implicates the pro-inflammatory effects of IL-6 in the pathophysiology of diabetes, CKD and CVD. Thus, targeting the IL-6 pathway holds a therapeutic potential in these cardiometabolic disorders. However, because IL-6 has multiple homeostatic roles, antagonizing this cytokine may be associated with side effects, such as increased risk of infection as seen with other anti-inflammatory drugs. Additional studies are required to establish the benefit-risk profile of anti-IL-6 intervention in the cardiometabolic diseases, whilst also considering alternative interventions such as lifestyle changes. IL-6 is also elevated in NASH, but the clinical usefulness of targeting IL-6 in this hepatic disorder remains largely unexplored.
1. Introduction
Diabetes, chronic kidney disease (CKD) and cardiovascular disorders (CVD), are among the most frequent causes of death globally. Whilst the etiologies of these cardiometabolic diseases are complex and intertwined, they are all associated with chronic inflammation of varying degrees [Citation1–3]. It remains to be fully elucidated to what extent inflammation constitutes a pathogenic trigger. Nevertheless, anti-inflammatory therapies have substantiated the causal role of inflammation in these diseases, and a growing body of evidence supports that the pro-inflammatory but pleiotropic cytokine interleukin (IL) 6 plays a central role. Levels of IL-6 and the associated biomarker C-reactive protein (CRP) are strong predictors of type 2 diabetes and all-cause mortality and genetically down-regulated IL-6 expression is associated with a favorable cardiometabolic profile [Citation4].
Anti-inflammatory agents include potent biologics to inhibit major pro-inflammatory cytokines, such as tumor necrosis factor (TNF) α and interleukin (IL) 1, the latter of which has been targeted in especially CVD [Citation5]. However, because of their physiological and orchestrating importance in, for example, innate and adaptive immunity, antagonizing these cytokines is associated with side effects, including risk of opportunistic and serious infections [Citation6]. Therefore, although anti-cytokine biologics have revolutionized the pharmacotherapy in rheumatic disorders, a favorable benefits/risks balance for agents blocking proinflammatory cytokines has not yet been firmly established in the setting of cardiometabolic disease.
Attention has turned towards antagonizing more downstream components of the complex inflammatory cascade to avoid undesired effects. With the therapeutic success of agents blocking the pivotal and pleiotropic cytokine IL-6 in, e.g., rheumatoid arthritis [Citation7], it has proven clinically feasible to antagonize a cytokine with an acceptable risk of serious or irreversible side effects. This arguably opens up for considering such anti-cytokine interventions in a broader range of diseases with known or putative inflammation-related pathologic components.
In this review, we outline how the inhibition of IL-6 may hold clinical potential in addressing the inflammatory components of the pathophysiology of the cardiometabolic disorders. These disorders are associated with a range of other inflammation- and metabolism-related complications and disorders. To exemplify this, we end the review by outlining a case for the use of IL-6 antagonism in nonalcoholic steatohepatitis (NASH) which is characterized by a profound scarcity of therapeutic options.
2. The biology of interleukin 6
Originally identified as a proinflammatory cytokine that promote B cell differentiation, activate T cells and regulate the acute-phase response, IL-6 is now also recognized as a hormone-like cytokine due to its diverse physiological effects [Citation8]. Synthesis of IL-6 takes place in almost all cell types [Citation8], including cells of the immune system, delineating the pleiotropic nature of a cytokine that also plays a role in regulating metabolism [Citation9], including insulin sensitivity, energy expenditure and lipid homeostasis, as well as endothelial function [Citation10], neuronal activity [Citation11,Citation12] and more (). Whilst the expression of IL6 is regulated via multiple pathways, main inducers of IL6 expression are TNF-α and IL-1β, two of the major upstream pro-inflammatory cytokines. Other cytokines, as well as adipokines, prostaglandins and Toll-like receptors, also induce IL-6 production.
Figure 1. The effects and blockade of interleukin 6 signaling.
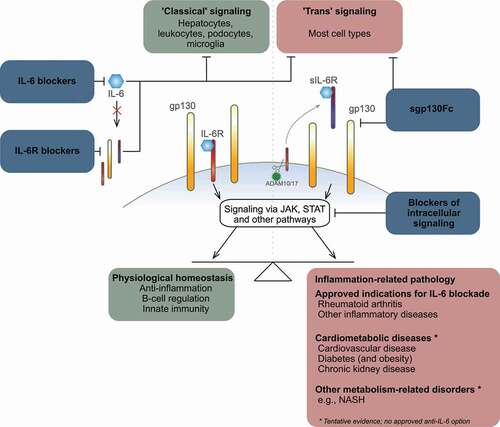
Highlighting its important protective role in the immune response, including in lymphocyte simulation, systemic levels of IL-6 rapidly increase during infections, and inability to induce IL-6 is associated with recurrent or persistent viral, bacterial, parasitic or fungal infections [Citation13]. Conversely, IL-6 plays a major role in maintaining the inflammatory state in, for example, arthritis and other autoimmune diseases, in which IL-6 levels are chronically elevated [Citation14]. IL-6 is a potent activator of the acute-phase response and induces the hepatic production of CRP, which is a widely used biomarker of systemic inflammation. Of note, CRP can now be measured using high-sensitivity assays (hsCRP) [Citation15], which allows for detection of low-grade inflammation, which, especially chronically, plays a major role in the development of, for example, CVD [Citation16]. Thus, as discussed later in this article, IL-6 is linked with diseases, such as diabetes, kidney disease and CVD, which all are pathophysiologically associated with chronic inflammation and elevated levels of IL-6.
2.1. Interleukin 6 signaling pathways
Understanding the IL-6 signaling pathways is key to realizing the therapeutic potential of anti-IL-6 interventions. IL-6 mediates its actions via the two-subunit IL-6 receptor complex, which comprises the IL-6-binding α-subunit (IL-6 R, CD126) and a signal-transducing β-subunit (gp130, CD130) () [Citation8,Citation17]. The activated IL-6 R complex induces cellular processes via multiple pathways, including those involving the Janus kinase (JAK) family and signal transducers and activators of transcription (STAT). In fact, measuring STAT1 and STAT3 activity has been used as a way to assess how IL-6 affects disease risk and outcomes [Citation18,Citation19].
The full complexity of the signaling pathways has been comprehensively reviewed by others [Citation8,Citation17]; here, highlights are presented next to outline how the different IL-6 signaling modes according to the prevailing view each play a role in health and disease, respectively.
IL-6 R (the α-subunit) is primarily expressed on leukocytes and hepatocytes but also on, for example, podocytes and microglia. In contrast, gp130 is expressed on all somatic cell types. An IL-6 family of cytokines has been defined [Citation14]; collectively, cytokines belonging to this family are essential regulators of cell survival and growth, hematopoiesis and general development.
IL-6 signaling in humans is known to take place via two main mechanisms (). First, the assembled, membrane-bound two-subunit IL-6 R complex (IL-6 R and gp130) mediates IL-6 actions in cells that express IL-6 R; this is referred to as the ‘classical’ signaling pathway, which is considered the main mechanism by which IL-6 co-regulate various homeostatic processes, including glucose metabolism. Second, circulating IL-6 may bind soluble IL-6 R (sIL-6 R) and mediates actions in all cells that express gp130; this is known as ‘trans’ signaling, which mediates the pro-inflammatory effects of IL-6. sIL-6 R is mainly generated as the result of the proteolytic cleavage of IL-6 R from the cell membrane; the membrane-bound metalloprotease ADAM10/17 is responsible for the cleavage of IL-6 R, as well as of multiple other substrates, including the TNF-α receptor. Readers are referred to an excellent recent review by Schumacher and Rose-John [Citation20] for an in-depth discussion of this aspect; here, it is relevant to note that ADAM10/17 plays a major physiological role in moderating IL-6 ‘trans’ signaling, which in turn contributes to regulation of innate immunity, including hematopoiesis and neutrophil recruitment via granulopoiesis. Moreover, via the ‘trans’ signaling pathway, IL-6 modifies the activity of several T cell pools, thereby governing parts of adaptive immunity. A third ‘trans-presentation’ signaling mode has been identified in mice, whereas its relevance in humans remains relatively unknown. Here, an antigen-specific interaction between a dendritic cell presenting IL-6 to T cells causes these T cells to commit to a pathogenic effector T cell pool.
The implications of the IL-6 signaling pathways in diabetes, kidney disease and cardiovascular disease are not fully established. However, for example, because IL-6 R is not expressed on endothelial cells (except in the brain, according to some studies) [Citation8], ‘classical’ IL-6 signaling does not occur in this cell type. In the kidneys, podocytes, as discussed later, express IL-6 R on the cell membrane surface [Citation8] and the 'classical' signaling pathway may therefore be involved in kidney disease to the extent podocytes are involved directly or indirectly in renal pathogenesis. As noted above, whilst ‘classical’ IL-6 signaling occurs in only a few cell types, considering that gp130 is expressed on most cell lines, IL-6 ‘trans’ signaling is believed to be active in the cell types directly implicated in the pathophysiology of cardiorenal disease (e.g. vascular endothelial cells) and diabetes (e.g. pancreatic β cells).
3. Current therapeutic uses and challenges
Overall, the complexity and duality of the IL-6 signaling mechanisms inarguably reinforce the notion that targeting IL-6 therapeutically is not straightforward and may interfere with important physiological functions. Moreover, the therapeutic potential of IL-6-targeted therapies may differ based on which of the signaling pathways are targeted.
Currently, three monoclonal antibodies (mAbs) blocking IL-6 activity are licensed for clinical use (). Tocilizumab, arguably the best-known agent, is one of two licensed blockers that target the IL-6 R as a treatment for rheumatic disorders, including RA. Saralimumab also targets the IL-6 R and is indicated in RA. Licensed for use in Castleman’s disease, siltuximab is the one approved mAb against the IL-6 ligand. A number of other IL-6/IL-6 R blocking mAbs are also being developed. Anti-IL-6 mAbs listed in all inhibit the ‘classical’ and ‘trans’ signaling pathways, while the anti-IL-6 R compounds inhibit all three signaling modes including ‘trans’-presentation. To our knowledge, compounds in clinical development selectively targeting IL-6 ‘trans’ signaling include a single compound (sgp130Fc, olamkicept), for which encouraging results were recently reported in inflammatory bowel disease [Citation21]. Finally, various small-molecule inhibitors targeting IL-6-specific JAK pathways are also approved (e.g. baricitinib, tofacitinib and upadacitinib) or in development [Citation22]. Of particular contemporary interest, many IL-6 inhibitors, including tocilizumab [Citation23], have been investigated in clinical trials with some success for the treatment of moderate/severe COVID-19.
Table 1. Licensed and selected in-development blockers of interleukin 6 signaling
Blocking IL-6 for anti-inflammatory benefits is not a curative intervention, and prolonged or chronic treatment is usually needed to suppress the underlying pathology. This fact accentuates that IL-6 blockade should ideally be done in a way that does not lead to increased susceptibility to infections or other adverse side effects. Avoiding interfering with the pivotal homeostatic controls exercised by IL-6 also needs to be balanced against the level of anti-inflammation needed for adequate disease management.
4. Interleukin 6 in diabetes
Diabetes mellitus is a highly prevalent cardiometabolic disease and comprises type 1 and type 2 diabetes (T1D and T2D). While a common feature is insulin deficiency, the pathogenesis of the two disorders is very different. T1D is a classic autoimmune disorder, in which the insulin-producing β cells of the pancreatic islets are gradually destroyed by a self-intolerant immune system [Citation24]. The pathogenesis of T2D represents several metabolic derangements; at least eight according to the ‘ominous octet’ model, among which inflammation is a key contributor [Citation25]. These derangements contribute in complex interdependent ways to insufficient insulin secretion relative to the level of, primarily muscular and hepatic, insulin sensitivity [Citation26]. Ultimately, irrespective of the cause, in both type 1 and type 2 diabetes, hyperglycemia ensues as the key downstream inducer of several pathogenic processes that results in micro- and macrovascular complications. Among these processes is the intracellular generation of reactive oxygen species, which promote and sustain, via epigenetic changes, pro-inflammatory pathways. Although type 1 diabetes according to the ‘double-diabetes’ hypothesis [Citation27] (i.e. type 1 diabetes accompanied by overweight, family history of type 2 diabetes and insulin resistance) qualifies as a cardiometabolic disease, its place in this family of diseases is fully justified because of the increased risk of vascular complications associated with type 1 diabetes as the results of the uncontrolled, chronic hyperglycemia.
Diabetes and overweight and obesity are closely linked; however, whether excess body weight in T2D, and in particular in T1D, is a pathogenic trigger or a symptom (or both) remains debatable. The prevalence of diabetes mellitus, as well as obesity, is increasing, and especially the number of people living with T2D has reached epidemic proportions and continues to rise [Citation28]. Whilst many compounds are available to control glycemia, patients frequently develop macro- and/or microvascular complications in both T2D and T1D [Citation29]. In fact, the overall mortality in T1D is 3-fold higher than for the general population and higher than in any other disease, including T2D, primarily due to death from cardiovascular causes [Citation30].
With its multiple roles in maintaining glucose homeostasis [Citation31], IL-6 is an interesting target in diabetes. IL-6 has an enhancing effect on insulin-induced clearance of glucose in the liver and in skeletal muscle, and treatment with IL-6 infusions (at 5 μg/hour) has been shown to augment insulin-induced glucose disposal in humans [Citation32]. Furthermore, IL-6 is secreted from the skeletal muscle during exercise [Citation33]. Moreover, IL-6 has been shown in humans to augment insulin secretion by increasing the secretion of glucagon-like peptide 1 [Citation34]. Highlighting the duality of the cytokine, however, it has long been suggested that systemic chronic inflammation mediated via pro-inflammatory cytokines such as IL-6 is central in the pathophysiology of T2D () [Citation35,Citation36]. For example, it is well established that chronic inflammation promotes insulin resistance [Citation37,Citation38] and blocking IL-6 with tocilizumab improved insulin resistance [Citation39]. In persons with T2D, elevated levels of IL-6 and CRP have been detected in several studies [Citation40] and are likely related to excess adipose tissue () [Citation41]. In such tissue, macrophages express IL-6, which promotes fatty acid oxidation and thus lipolysis [Citation42]. Accordingly, in obese individuals, including those without T2D, IL-6 levels are elevated [Citation43]. It is of note, however, that treatment with anti-IL-6 agents is associated with an increase in body weight [Citation44], likely because of the overall catabolic effect of physiological levels of IL-6 in adipose tissue. The excess IL-6 produced by macrophages in adipose tissue may also exacerbate insulin resistance and promote gluconeogenesis in the liver [Citation45].
Figure 2. Interleukin 6 in cardiometabolic disorders.
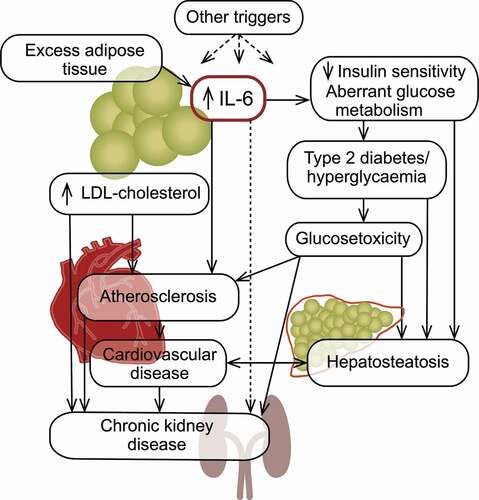
4.1. Current evidence in type 1 diabetes
In T1D, the complex and incompletely elucidated immunopathogenesis involves destructive autoimmune processes, essentially due to an imbalance in the pools of immune cells and malfunctioning pancreatic β cells that co-orchestrate their own immune-mediated demise or destruction by making themselves visible or susceptible to the immune effector cells [Citation24]. The direct or indirect importance of IL-6 in the T1D immunopathogenesis – whether the impacts of the cytokine are positive, negative or both – continues to be unclear and as evidence builds, so does the complexity [Citation46]. As outlined earlier in this review, IL-6 is a potent regulator of several T cell pools; the cytokine is involved in regulating potentially auto-aggressive T effector cell and T helper 17 (Th17) cell pools, as well as in inhibiting T regulatory cells [Citation18]. Th17 cells activated via the ‘trans’-presentation mode involving IL-6 become pathogenic and involved in autoimmunity and tissue damage. IL-6 ‘trans’ presentation ‘signaling’ may therefore be involved in initiating the immunopathogenesis of T1D, as supported by a finding of increased Th17 cell counts in patients with new-onset T1D [Citation47]. Increased IL-6 ‘trans’ signaling [Citation18] and increased expression of IL-6 have been detected in monocytes from individuals with T1D [Citation48], whom may also carry a specific genetic variant of the IL-6 R [Citation49].
Both elevated [Citation50,Citation51,] and normal [Citation18,Citation52,Citation53] circulating levels of IL-6 have been found in patients with T1D, with indications of an age dependency. A recent meta-analysis concluded that the cytokine is in fact elevated in the disease [Citation35] and corroborated the view that the relevance of IL-6 in T1D may depend on the age of the patients, and therefore also largely on the disease stage. However, the situation is likely even more complex because, as discussed earlier, IL-6 is associated with glucose disposal. Moreover, in rodents and ex vivo human islets, IL-6 has been shown to reduce oxidative stress [Citation54], which is one of the various stress factors that are considered co-responsible for the demise of the β cells in T1D. Moreover, and importantly, a recent study showed that whilst pancreatic islet cells (α as well as β cells) constitutively expressed IL-6 in line with its physiological roles and previous evidence [Citation55], the expression was greatly diminished in insulin-deficient donor islets [Citation56].
Providing clinical evidence, results from the phase 2 EXTEND trial investigating the treatment of new-onset T1D with tocilizumab were recently reported [Citation57]. In RA, tocilizumab has been show to exert its proven benefits by promoting T regulatory cells and inhibiting pathogenic Th17 cells [Citation58], which as mentioned above are suggested to be involved in the T1D immunopathogenesis. However, the EXTEND trial showed that blocking the IL-6 receptor with tocilizumab did not slow the loss of β cells in new-onset T1D.
Taken together, it is clear from current evidence, that the role of IL-6 in T1D is a good example of how the cytokine plays a role in both health and disease. Accordingly, the exact and net role of IL-6 in the pathophysiology of T1D remains elusive and will require further studies to unravel. The fact that IL-6 receptor blockade with tocilizumab did not appear to improve the outcome in newly diagnosed T1D might suggest a need to focus any IL-6-directed intervention also in this disease on the ‘trans’ signaling pathways, i.e. the IL-6 related pathways believed to be associated with pathogenesis.
4.2 Current evidence in type 2 diabetes
In general, the potential of IL-6 blocking to lower the risk of T2D onset or improve glycemic control in T2D remains largely hypothetical and evidence in support is limited or indirect. Whilst blocking IL-6 specifically has not been studied in T2D in clinical trials of scale, a recent meta-analysis concluded that the role of IL-6 in the disease is minor [Citation59]. Moreover, blocking IL-1β with canakinumab in the large CANTOS (‘Canakinumab Anti-Inflammatory Thrombosis Outcomes Study’) trial (further discussed below) in patients without diabetes but with previous myocardial infarction and with high CRP levels did not reduce blood glucose levels or the risk of new-onset diabetes, despite the significant reduction of systemic inflammation [Citation60]. Furthermore, in line with the well-established molecular mechanism by which TNF-α negatively interferes with insulin signaling [Citation36], inhibition of this proinflammatory cytokine has been shown to reduce insulin resistance in ob/ob mice but not HbA1c levels in humans with T2D [Citation26]. Considering that TNF-α and IL-1β are upstream inducers of IL-6 signaling – predominantly the ‘trans’ signaling mode via their ability to activate ADAM10/17 – these findings do not seem to support that blocking IL-6 have an impact on hyperglycemia in T2D.
Taken together, IL-6 inhibition to prevent disease onset or ameliorate the hyperglycemia does not appear to be attractive based on evidence accumulated hitherto. In addition, even though current evidence suggests only a small overall benefit, the finding of genetic variations in the component involved in IL-6 signaling [Citation61] might suggest that certain T2D subgroups may particularly benefit from IL-6 inhibition. Studies exploring this aspect are not available; until they are, the case for IL-6 blockade in isolated T2D arguably is not supported by a favorable benefits/risks balance. Nevertheless, addressing chronic inflammation in this archetypal cardiometabolic disease to avoid the associated complications including CKD and CVD might be very relevant as discussed below.
5. Interleukin 6 in chronic kidney disease
CKD () is a common cardiometabolic disease, defined by the progressive loss of renal function [Citation62]. CKD, which has many underlying causes, usually advances through stages of reduced and ultimately very low glomerular filtration rates (end-stage renal disease). CKD develops over the course of multiple years and contributes increasingly frequently to morbidity and premature mortality. Highlighting the burden of the disease, estimates implicated CKD in 1.4 million deaths globally in 2019, where around 700 million cases of CKD were identified, representing a 24% prevalence increase since 2010 [Citation63]. CKD appears to be profoundly underdiagnosed and ways to manage CKD are sparse [Citation62].
Figure 3. Interleukin 6 in chronic kidney disease.
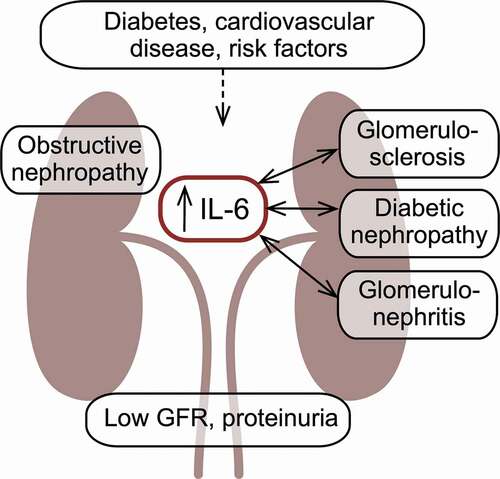
Collectively, cardiometabolic disease, including especially diabetes but also obesity, hypertension and CVD, represents the main CKD-associated risk factors; other factors such as genetic susceptibility, race, advanced age, and smoking also contribute to the overall risk for CKD [Citation64]. Local inflammation is present in many patients with CKD [Citation65] and based on the location of the inflammation in the kidneys, CKD can be classified as glomerulonephritis or interstitial nephritis [Citation62]. CKD affects all parts of the kidney, which gradually becomes fibrotic, void of functional cells and characterized by glomerulosclerosis [Citation62]. A multitude of hemodynamic and metabolic changes ensue.
As a major risk factor for CKD, diabetes is the leading cause of kidney failure [Citation66], representing one of the intertwined and omnidirectional relationships connecting the cardiometabolic diseases. As many as 40% of patients with both type 1 and type 2 diabetes suffer from diabetic nephropathy [Citation67], the risk of which may be associated with IL6 polymorphisms [Citation68]. This reflects the view that CKD in patients with diabetes is the renal manifestation of the toxic excess glucose levels characterizing diabetes. Individuals with diabetes appear to develop CKD regardless of their level of glycemic control [Citation69]. Conversely, longitudinal studies have shown that early and intensive intervention to control glycemia markedly and sustainably reduce the risk of CKD in patients with diabetes [Citation70].
Many proinflammatory cytokines are considered to be either inducing or exacerbating CKD [Citation71,Citation72]. Moreover, patients with diabetes and with diabetic nephropathy have higher IL-6 levels than patients without the nephropathy [Citation73]. IL-6 is produced by all types of kidney cells, i.e., podocytes and mesangial, endothelial and epithelial cells, as reviewed by Su et al. [Citation74] and summarized below. Only podocytes express IL-6 R and therefore are the only renal cell type relevant in terms of IL-6 ‘classical’ signaling. In contrast, all cells of the kidney express the gp130; accordingly, all these cells are susceptible to the overall pathogenic effects of IL-6 ‘trans’ signaling. Podocytes, in response to elevated glucose levels, have been shown to also be capable of producing IL-6 and it has been reported that in rats, IL-6 blockade prevents podocyte apoptosis and damage. Mesangial cells also have been shown to respond ominously to elevated IL-6 levels by recruiting monocytes by secretion of chemoattractant protein 1. Despite the apparent involvement of ‘classical’ IL-6 signaling in only one type of kidney cells, both this and the ‘trans’ signaling pathway have been shown to be active in the development of diabetic nephropathy [Citation68]. The CKD-related renal abnormalities associated with high IL-6 expression include changes to the endothelium (for example, increased permeability), podocyte damage, glomerular hypertrophy, and increased fibronectin expression by the mesangium, the expansion of which has been found to correlate with IL-6 mRNA levels.
In alignment with the seemingly pathogenic net effect of IL-6 in the cells of the kidney, IL-6 is widely considered to be an important factor in either the induction or progression of CKD. Accordingly, systemic IL-6 levels have been shown to be elevated even in early-stage CKD [Citation74], and IL-6 levels have been shown to be an independent predictor of mortality in later-stage CKD Citation75. Impaired function may also lead to a lower renal clearance of IL-6, suggesting that elevated IL-6 levels may be a manifestation of CKD and not entirely a pathogenic factor.
CKD may also be the result of inflamed and consequently damaged glomeruli, i.e. glomerulonephritis, the symptomatology and etiology of which are diverse. The primary causes of glomerulonephritis are kidney-specific; secondary causes include infections, systemic lupus erythematosus, vasculitis and diabetes. Several animal studies have implicated IL-6 and members of the cytokine family in the development of glomerulonephritis [Citation76–78], and elevated urine and renal IL-6 levels are associated with poor outcomes in mesangial proliferative glomerulonephritis, where IL-6 induces the mesangial proliferation [Citation79]. However, non-clinical and clinical studies of inhibition of the IL-6 pathways have shown mixed results using both ‘classical’ signaling blockade of IL-6 and IL-6 R and inhibition of the ‘trans’ signaling pathway with sgp130Fc, as well as antibodies targeting other members of the IL-6 cytokine family [Citation68].
Elevated IL-6 levels have also been associated with a separate form of CKD due to glomerulosclerosis, i.e., focal segmental glomerulosclerosis [Citation80]. Whether these observations represent independent pathogenic processes or if they reflect the underlying pathology is not established. Future studies are needed to elucidate if targeting the IL-6 pathways in focal segmental glomerulosclerosis has beneficial effects on renal function.
Thus, whilst evidence implicate many other cytokines in the development or exacerbation of CKD, targeting IL-6 specifically for anti-inflammatory intervention in CKD appears to be a promising avenue to pursue. However, none of the currently available IL-6 inhibitors are licensed for use in kidney disease, and clinical attempts at IL-6 inhibition in CKD have been sparse. Although no beneficial effect on kidney function was observed with canakinumab, indirect evidence from the CANTOS trial supported that patients with CKD and elevated IL-6 levels constitute a subgroup that benefits particularly well in terms of CVD risk reduction from intervention to reduce inflammation [Citation81]. Recently, results of the RESCUE (‘Reduction in Inflammation in Patients with Advanced Chronic Renal Utilizing Antibody-Medicated IL-6 Inhibition’, ClinicalTrials.gov identifier NCT03926117) trial were reported [Citation82]; this was a phase 2 trial that investigated the anti-inflammatory effects of the human monoclonal anti-IL-6 ligand antibody ziltivekimab in patients with advanced CKD and elevated levels of C-reactive protein. Outcomes measures of the RESCUE trial focused on CVD-relevant endpoints and general markers of inflammation; thus, kidney-specific outcomes were not reported beyond the glomerular filtration rate and urinary albumin-to-creatinine ration, both of which were not affected by ziltivekimab compared with placebo. Ziltivekimab treatment resulted in statistically significant placebo-adjusted reductions of CRP of up to 92% after 12 weeks. Whether or not ziltivekimab also has beneficial effects on renal function is currently unknown.
In another study, ziltivekimab appeared to benefit hemodialysis-requiring CKD in patients with a single nucleotide polymorphism in a gene (TMPRSS6), which has been shown to increase the pro-inflammatory actions of IL-6 [Citation83]. The high inflammatory status present in patients with CKD requiring hemodialysis frequently results in anemia, which is related to IL-6-mediated upregulated expression levels of the iron-regulating hormone hepcidin [Citation84], as well as erythropoietin resistance [Citation85]. Patients with advanced CKD may therefore require erythropoiesis-stimulating agents such high-dose erythropoietin, which has been shown to be associated with increased incident CVD risk [Citation86]. In the placebo-controlled phase 1/2 study, patients receiving ziltivekimab had a lower need for erythropoiesis-stimulating agents, and their levels of inflammatory markers, including hsCRP, were significantly reduced.
While these results with ziltivekimab do not provide evidence of a direct benefit of ziltivekimab on renal function, the hypothesis is that the marked anti-inflammatory effects of the ziltivekimab can result in CVD risk reduction in patients with CKD. The potential benefit of ziltivekimab on CV risk in patients with CKD will be explored in the ZEUS (Ziltivekimab Cardiovascular Outcomes Study; ClinicalTrials.gov identifier NCT05021835) phase 3 cardiovascular outcomes trial (also discussed below in the section on CVD) [Citation87], which will also expand on the safety and tolerability profile of ziltivekimab demonstrated in the RESCUE trial, which found only minimal evidence of bone marrow suppression, risk for infections, hepatic toxicity, or change in atherogenic lipid levels.
6. Interleukin 6 in cardiovascular disease
Cardiovascular disorders (CVDs) continue to be the world’s leading cause of death [Citation88] as the final outcome of a prolonged pathogenesis that ultimately leads to coronary artery disease (CAD), stroke or peripheral artery disease (PAD). Heart failure is another frequently observed manifestation of (cardio)vascular morbidity.
The promise of IL-6 inhibition in CVD was recently comprehensively reviewed by Ridker and Rane [Citation87], and readers are referred to that excellent resource for a recent update. Below, we present selected highlights for ASCVD with focus on pre-clinical and clinical experience gained hitherto and expected in the near future. In addition to stable CVD (ASCVD and heart failure), reports suggest a potential beneficial role of anti-inflammatory agents in patients with acute coronary ischemia, which is associated with acute inflammation during ischemia/reperfusion injury. In patients with myocardial infarction, a single dose of tocilizumab was recently shown to modestly but significantly improve the myocardial salvage index when given in connection with an acute ST-segment–elevation myocardial infarction [Citation89].
The vicious interplay between the cardiometabolic diseases is arguably epitomized by the ASCVDs [Citation90–93] with inflammation as a common denominator. Here, a landmark was reached with the CANTOS trial, which indicated a CV risk reduction by means of IL-1β inhibition with canakinumab only in participants whose circulating IL-6 levels were also markedly reduced by canakinumab [Citation5,Citation94,Citation95]. CANTOS, together with recent reports confirming the CV risk-reducing effects of the inorganic anti-inflammatory compound colchicine [Citation96,Citation97], reinforced the promise of anti-inflammatory intervention in CVD [Citation98–100]. Presently, however, canakinumab is not approved for use in CVD, whereas colchicine was recently approved in Canada for CV risk reduction in patients with CAD. Still, although proven markedly beneficial in CVD, colchicine, which undergoes renal clearance, may not be optimal for the many patients with both CVD and CKD. On this background and in light of the CANTOS findings, IL-6 antagonism inarguably emerges as an intriguing proposition for anti-inflammatory intervention in CVD.
Preceding CANTOS, which is considered a proof-of-concept trial when it comes to the role of inflammation in CVD, several years of evidence from animal models and clinical trials had firmly associated chronic low-grade inflammation with both atherosclerosis and heart failure [Citation101]. A large body of evidence from animal models show the multiple actions and effects of IL-6 as it relates to vascular physiology and pathophysiology. In ASCVD, chronic inflammation is one of the major abnormalities responsible for starting and exacerbating the atherosclerotic process, by which lesions in the artery wall develop and ultimately cause endothelial dysfunction. Studies have shown that IL-6 is expressed by endothelial cells in response to various stress factors, including inflammation [Citation102]. Pathogenic vascular effects of IL-6 include pro-coagulant effects via cellular and humoral effectors [Citation103], and induction of vascular defects, such as increased vascular permeability [Citation104] and expression of cell adhesion molecules [Citation105]. In keeping with the notion that the ominous effects of IL-6 are mediated via the ‘trans’ signaling pathway, this IL-6 signaling mode has been shown to contribute to atherosclerosis [Citation106] and to destabilization of atherosclerotic plagues [Citation107].
Whilst results from the CANTOS trial are the most recent and arguably also the conclusive piece of evidence corroborating a central role for IL-6 in the inflammation-related pathophysiology of CVD, prior discoveries and data had instilled realism in the promise of pursuing anti-IL-6 intervention in CVD. In brief, studies from the past decades have found that elevated systemic levels of IL-6 and CRP are associated with increased risk of CV morbidity or mortality [Citation108–110]. CRP was later qualified as a clinically useful biomarker but not a causal factor in ASCVD; in contrast, a causal relationship of IL-6 with ASCVD has been strongly substantiated using approaches such as Mendelian randomization, which robustly assesses causal relationships between, for example, a protein and an outcome [Citation94,Citation101,Citation111]. Increased levels of both hsCRP and IL-6 are now recognized predictors of poor cardiovascular outcomes.
To answer whether targeting the IL-6 pathways may reduce the risk for CVD, the large-scale ZEUS CVOT (6200 patients with CKD and elevated CRP levels) has recently been initiated in August 2021 [Citation99]. The aims of the ZEUS trial are many; the primary objective is to demonstrate that ziltivekimab reduces the risk of major adverse cardiovascular events in patients at high CV risk. In addition, ZEUS will also explore the effect of IL-6 inhibition with ziltivekimab on the risk of heart failure leading to hospitalization or urgent visits. Furthermore, as discussed above, a secondary objective is to explore the effect of ziltivekimab on a number of CKD-related outcomes. Just as importantly, the ZEUS trial will also provide additional safety data to corroborate or refine the seemingly acceptable safety profile seen in RESCUE, where ziltivekimab did not appear to be associated with the side effects seen with the use of, for example, tocilizumab in RA. Accordingly, as discussed above, ziltivekimab in RESCUE did not appear to markedly increase lipid levels or to induce (sustained) neutropenia.
A role for IL-6 in other vascular pathologies has also been suggested. For example, IL-6 has long been implicated in the formation and progression of abdominal aortic aneurysm (AAA), a disorder characterized by progressive dilation of the aortic wall, which can lead to aortic rupture and is associated with high mortality [Citation112,Citation113]. The pathogenesis of AAA is intimately associated with degradation of the extracellular matrix and with collagen deposition. This process is mediated by matrix metalloproteinases (MMPs), the production of which by inflammatory cells is directly stimulated by IL-6 [Citation114]. Human AAA biopsies contain significantly higher levels of IL-6 compared to controls [Citation115] and observational studies have consistently linked high IL-6 levels with AAA [Citation116]. A long-term prospective study has demonstrated that elevated IL-6 levels are independently associated with future AAA [Citation117]. Interestingly, circulating IL-6 levels are also correlated with the aortic diameter in subjects without AAA, suggesting an early effect of IL-6 in AAA formation [Citation113]. These findings were corroborated by several Mendelian randomization studies linking IL-6 to the pathophysiology of the disease [Citation116,Citation118]. In experimental murine models, IL-6 antibody treatment was shown to the lower the growth rate and prevent aortic rupture [Citation119]. While the current body of evidence indicates that IL-6 is an active partaker in the pathogenesis of AAA, it is unknown whether targeting the IL-6 pathway results in halting aortic aneurysm growth in humans.
Other morbidities related to cardiovascular complications and mortality and with suggested links to inflammation and IL-6 specifically include hypertension [Citation120,Citation121]), cardiac hypertrophy [Citation122] and myocardial fibrosis [Citation123]. However, larger clinical studies to investigate the utility of IL-6 inhibition in these conditions are, to our knowledge, not available.
7. Interleukin 6 in NASH
NASH is a progressed stage of nonalcoholic liver disease (NAFLD) [Citation124] and is estimated to be present in a substantial part of the general population [Citation125], although it is not diagnosed in the majority of the cases. NASH is intimately associated with cardiometabolic diseases: around 37% of individuals with either type 2 diabetes or obesity also have NASH [Citation126], which predisposes for high cardiovascular risk [Citation127]. The pathophysiology of NASH is complex and multifactorial [Citation128,Citation129]. Overall, it involves metabolic derangements starting with or exacerbated by peripheral insulin resistance (), which may lead to steatosis and lipotoxicity, fibrogenesis and cirrhosis. Inflammation, which manifests via elevated circulating and intrahepatic levels of proinflammatory cytokines including IL-6 [Citation130], is believed to be a driving factor in the fibrogenesis, as well as in NASH-related oncogenesis. Currently, no pharmacological treatments are approved to prevent, manage or reverse NASH. However, arguably spurred by the increased recognition of the high and rising prevalence of the disorder, a number of initiatives are ongoing, many of which target the metabolic derangements [Citation128,Citation131]. Current in-development anti-inflammatory intervention include a focus on parts of the inflammation-related pathogenesis other than the IL-6-related pathways, such as C–C motif chemokine receptor 2 and receptor 5 antagonism [Citation132,Citation133]. In animal models of NASH, IL-6 blockade has not been shown to have a beneficial effect on NASH parameters [Citation134]. Nevertheless, the fact that IL-6 levels are elevated in the disease could indicate that IL-6 blockade may be beneficial in breaking the vicious fibrosis-inducing circles in NASH. The fact that no pharmacotherapeutic intervention is available to effectively prevent or ameliorate NASH, clinical trials using IL-6 blockers in NASH are arguably warranted but have not been initiated to our knowledge.
8. Conclusions
Evidence continues to mount in support of the involvement of IL-6 in the pathophysiology of the cardiometabolic diseases. Here, and in related disorders or complications, inhibition of the pivotal pro-inflammatory but pleiotropic cytokine holds therapeutic potential. At this point, however, clinical use requires further study to clarify the true potential relative to the safety and tolerability, and to other alternative interventions such lifestyle changes, i.e., to confirm a favorable benefits/risks profile in these prevalent and burdensome disorders. Second-generation anti-IL-6 or anti-IL-6 R antibodies targeting either of the three IL-6 signaling pathways, compounds targeting the predominantly pathogenic ‘trans’ signaling pathway exclusively, or blockers of intracellular signal transduction may prove to be what eventually establish IL-6 inhibition as a successful therapy beyond its current rather limited therapeutic application in a few other diseases.
9. Expert opinion
Still the leading causes of morbidity and premature mortality, the prevalence of the cardiometabolic diseases diabetes, cardiovascular disease (CVD) and chronic kidney disease (CKD), as well as the associated complications, continues to rise. In parallel, treatment options in general remain sparse.
As our understanding of the pathophysiology of the disorders increases, evidence continues to build that their pathophysiology is intimately associated with chronic inflammation of varying degrees as a common denominator across the cardiometabolic diseases, as well as related conditions such as nonalcoholic hepatosteatosis (NASH).
Interleukin (IL) 6 – a pivotal cytokine with multiple physiological functions, including in host defense, but also with profound pro-inflammatory effects – is one of the cytokines that are increasingly recognized as being intimately involved in the pathogenesis of these diseases. Blocking IL-6 using monoclonal antibodies, of which three are licensed for clinical use, has proven its worth in, for example, rheumatoid arthritis. The arguably best-known compound is tocilizumab, which is an example of a successful anti-inflammatory therapeutic targeting a specific and downstream component of the complex inflammatory cascade to minimize the risk of side effects such as increased occurrence or reoccurrence of infections.
In type 1 diabetes, elevated levels of IL-6 have been found and may drive part of the immunopathogenesis resulting in the immune-mediated destruction of β cells in the pancreatic islet. Conversely, IL-6 appears to have physiologically important roles in the islets, exemplifying the pleiotropic nature of the cytokine. In type 2 diabetes, chronic inflammation, either independently or related to the excess adipose tissue often accompanying diabetes, is believed to lead to, for example, insulin resistance, thereby contributing to hyperglycemia. Chronic hyperglycemia, also in type 1 diabetes, causes glucotoxicity-induced damages to the endothelium, as well as in renal and neuronal tissue and elsewhere. However, the potential for anti-IL-6 intervention in type 1 and type 2 diabetes predominantly relates to the treatment of CVD and CKD, for which diabetes is a major risk factor. IL-6 blockade in CVD and CKD is being actively explored and arguably is a promising proposition as shown recently based on indirect evidence from the CANTOS trial with the IL-1β inhibitor canakinumab (IL-1 is one of the main inducers of IL-6 production) and direct evidence from the RESCUE trial with the anti-IL-6 monoclonal antibody ziltivekimab. On the farther horizon, IL-6 blockade may also prove beneficial in NASH, in which blood sample measurements and mechanistic evidence from primarily animal studies implicate IL-6 in both protective and damaging effects. However, for this disease, no known clinical initiatives are ongoing.
The dichotomy of the net effects of physiological IL-6 – i.e., the fact that the cytokine plays roles in both health and disease – relates to the two main signaling pathways used by IL-6. The ‘classical’ signaling pathway, which is employed by only some cell types, is generally associated with the homeostatic effects of the cytokine. The ‘trans’ signaling pathway is what evidence points toward as the pathway overly active in the diseases associated with elevated IL-6 levels and ominous, often chronic, inflammation. Anti-IL-6 antibodies and antibodies targeting the IL-6 receptor appear adequately safe and well tolerated, have proven their clinical therapeutic worth in inflammatory diseases, such as rheumatoid arthritis, and are being tested in late-stage clinical trials in multiple other indications, including CVD and CKD. Nevertheless, compounds targeting the ‘trans’ signaling pathway may address the inflammation-related part of pathophysiology of the in-scope diseases even more specifically.
Taken together, clinical trials of scale remain required to fully establish the place for anti-IL-6 interventions in the cardiometabolic diseases and their related complications and disorders.
Article highlights
Diabetes, chronic kidney disease (CKD) and cardiovascular disease (CVD) are associated with chronic inflammation. Recent evidence suggests that inflammation also plays a role in non-alcoholic hepatosteatosis.
The pro-inflammatory but pleiotropic cytokine interleukin (IL) 6 is implicated in the pathophysiology of these cardiometabolic diseases. Blocking IL-6 may hold therapeutic potential but remains to be fully studied.
In clinical trials in type 1 and type 2 diabetes, anti-IL-6 treatment has not been shown to remedy the hyperglycemia characterizing both diseases.
Although speculative at this point, IL-6 may play a role in the immunopathogenesis of type 1 diabetes; however, exemplifying the pleiotropic nature of the cytokine, recent evidence indicates that IL-6 also has important physiological roles in the pancreatic islets.
IL-6 blockade to address the central inflammation-related components of CVD and CKD, whether occurring independently or as complications to diabetes, is promising and is being actively studied in clinical trials of scale, including the ZEUS cardiovascular outcomes trial.
Utilizing the increasingly deep understanding of the complex IL-6 signaling pathways may facilitate the development of compounds targeting the ominous effects of the cytokine specifically.
IL-6 blocking in autoimmune disorders such as rheumatoid arthritis has been proven beneficial but is associated with increased risk of infections and other side effects. Evidence from additional clinical trials is required to establish the benefit–risk balance for the use of anti-IL-6 intervention in CVD, CKD and diabetes, as well as their related disorders and complications. In addition, the clinical utility of such pharmacotherapy should be considered in light of alternative interventions, such as lifestyle changes.
In our opinion, amongst the cardiometabolic diseases, CVD and CKD currently emerge as the best candidates for therapies targeting IL-6.
Declaration of interest
F F Kreiner, M von Herrath and B J von Scholten are employees and shareholders of Novo Nordisk A/S. G K Hovingh reports research grants from the Netherlands Organization for Scientific Research (vidi 016.156.445), CardioVascular Research Initiative, European Union and the Klinkerpad fonds, institutional research support from Aegerion, Amgen, AstraZeneca, Eli Lilly, Genzyme, Ionis, Kowa, Pfizer, Regeneron, Roche, Sanofi, and The Medicines Company; speaker’s bureau and consulting fees from Amgen, Aegerion, Sanofi, and Regeneron until April 2019 (fees paid to the academic institution); and part-time employment at Novo Nordisk A/S since April 2019. The authors have no other relevant affiliations or financial involvement with any organization or entity with a financial interest in or financial conflict with the subject matter or materials discussed in the manuscript apart from those disclosed.
Reviewer disclosures
Peer reviewers on this manuscript have no relevant financial or other relationships to disclose
Additional information
Funding
References
- Ruparelia N, Chai JT, Fisher EA, et al. Inflammatory processes in cardiovascular disease: a route to targeted therapies. Nat Rev Cardiol. 2017;14(3):133–144.
- Hutton HL, Ooi JD, Holdsworth SR, et al. The NLRP3 inflammasome in kidney disease and autoimmunity. Nephrology (Carlton, Vic). 2016;21(9):736–744.
- Tsalamandris S, Antonopoulos AS, Oikonomou E, et al. The role of inflammation in diabetes: current concepts and future perspectives. Eur Cardiol. 2019;14(1):50–59.
- Georgakis MK, Malik R, Li X, et al. Genetically downregulated interleukin-6 signaling is associated with a favorable cardiometabolic profile: a phenome-wide association study. Circulation. 2021;143(11):1177–1180.
- Ridker PM, Everett BM, Thuren T, et al. Antiinflammatory therapy with canakinumab for atherosclerotic disease. N Engl J Med. 2017;377(12):1119–1131.
- Murdaca G, Spanò F, Contatore M, et al. Infection risk associated with anti-TNF-α agents: a review. Expert Opin Drug Saf. 2015;14(4):571–582.
- Choy EH, De Benedetti F, Takeuchi T, et al. Translating IL-6 biology into effective treatments. Nat Rev Rheumatol. 2020;16(6):335–345.
- Rose-John S. Interleukin-6 signalling in health and disease. F1000Res. 2020;9:1013.
- Wallenius V, Wallenius K, Ahrén B, et al. Interleukin-6-deficient mice develop mature-onset obesity. Nat Med. 2002;8(1):75–79.
- Villar-Fincheira P, Sanhueza-Olivares F, Norambuena-Soto I, et al. Role of interleukin-6 in vascular health and disease. Front Mol Biosci. 2021;8:641734.
- Kummer KK, Zeidler M, Kalpachidou T, et al. Role of IL-6 in the regulation of neuronal development, survival and function. Cytokine. 2021;144:155582.
- Rothaug M, Becker-Pauly C, Rose-John S. The role of interleukin-6 signaling in nervous tissue. Biochim Biophys Acta. 2016;1863(6):1218–1227.
- Rose-John S, Winthrop K, Calabrese L. The role of IL-6 in host defence against infections: immunobiology and clinical implications. Nat Rev Rheumatol. 2017;13(7):399–409.
- Jones SA, Jenkins BJ. Recent insights into targeting the IL-6 cytokine family in inflammatory diseases and cancer. Nat Rev Immunol. 2018;18(12):773–789.
- Ridker PM. Clinical application of C-reactive protein for cardiovascular disease detection and prevention. Circulation. 2003;107(3):363–369.
- Arnold N, Lechner K, Waldeyer C, et al. Inflammation and cardiovascular disease: the future. Eur Cardiol. 2021;16:e20.
- Schaper F, Rose-John S. Interleukin-6: biology, signaling and strategies of blockade. Cytokine Growth Factor Rev. 2015;26(5):475–487.
- Hundhausen C, Roth A, Whalen E, et al. Enhanced T cell responses to IL-6 in type 1 diabetes are associated with early clinical disease and increased IL-6 receptor expression. Sci Transl Med. 2016;8(356):356ra119.
- Schneider A, Long SA, Cerosaletti K, et al. In active relapsing-remitting multiple sclerosis, effector T cell resistance to adaptive T regs involves IL-6–Mediated signaling. Sci Transl Med. 2013;5(170):170ra115.
- Schumacher N, Rose-John S. ADAM17 activity and IL-6 trans-signaling in inflammation and cancer. Cancers (Basel). 2019;11(11):1736.
- Schreiber S, Aden K, Bernardes JP, et al. Therapeutic Interleukin-6 trans-signaling inhibition by olamkicept (sgp130Fc) in patients with active inflammatory bowel disease. Gastroenterology. 2021;160(7):2354–2366.e2311.
- Harrington R, Al Nokhatha SA, Conway R. JAK inhibitors in rheumatoid arthritis: an evidence-based review on the emerging clinical data. J Inflamm Res. 2020;13:519–531.
- Mariette X, Hermine O, Tharaux PL, et al. Effectiveness of tocilizumab in patients hospitalized with COVID-19: a follow-up of the CORIMUNO-TOCI-1 randomized clinical trial. JAMA Intern Med. 2021;181(9):1241.
- von Scholten BJ, Kreiner FF, Gough SCL, et al. Current and future therapies for type 1 diabetes. Diabetologia. 2021;64(64):1037–1048.
- Defronzo RA. Banting Lecture. From the triumvirate to the ominous octet: a new paradigm for the treatment of type 2 diabetes mellitus. Diabetes. 2009;58(4):773–795.
- DeFronzo RA, Ferrannini E, Groop L, et al. Type 2 diabetes mellitus. Nat Rev Dis Primers. 2015;1:15019.
- Khawandanah J. Double or hybrid diabetes: a systematic review on disease prevalence, characteristics and risk factors. Nutr Diabetes. 2019;9(1):33.
- Saeedi P, Petersohn I, Salpea P, et al. Global and regional diabetes prevalence estimates for 2019 and projections for 2030 and 2045: results from the international diabetes federation diabetes atlas. Diabetes Res Clin Pract. 2019;157:107843. 9(th) edition
- Rawshani A, Rawshani A, Franzén S, et al. Mortality and cardiovascular disease in type 1 and type 2 diabetes. N Engl J Med. 2017;376(15):1407–1418.
- Petrie JR, Sattar N. Excess cardiovascular risk in type 1 diabetes mellitus. Circulation. 2019;139(6):744–747.
- Timper K, Denson JL, Steculorum SM, et al. IL-6 improves energy and glucose homeostasis in obesity via enhanced central IL-6 trans-signaling. Cell Rep. 2017;19(2):267–280.
- Carey AL, Steinberg GR, Macaulay SL, et al. Interleukin-6 increases insulin-stimulated glucose disposal in humans and glucose uptake and fatty acid oxidation in vitro via AMP-activated protein kinase. Diabetes. 2006;55(10):2688–2697.
- Pedersen BK, Febbraio MA. Muscle as an endocrine organ: focus on muscle-derived interleukin-6. Physiol Rev. 2008;88(4):1379–1406.
- Ellingsgaard H, Hauselmann I, Schuler B, et al. Interleukin-6 enhances insulin secretion by increasing glucagon-like peptide-1 secretion from L cells and alpha cells. Nat Med. 2011;17(11):1481–1489.
- Chen YL, Qiao YC, Pan YH, et al. Correlation between serum interleukin-6 level and type 1 diabetes mellitus: a systematic review and meta-analysis. Cytokine. 2017;94:14–20.
- Hotamisligil GS. Inflammation, metaflammation and immunometabolic disorders. Nature. 2017;542(7640):177–185.
- The diabetes control and complications trial research group, Effect of intensive therapy on residual beta-cell function in patients with type 1 diabetes in the diabetes control and complications trial. A randomized, controlled trial. The diabetes control and complications trial research group. Ann Intern Med. 1998;128:517–523
- Zatterale F, Longo M, Naderi J, et al. Chronic adipose tissue inflammation linking obesity to insulin resistance and type 2 diabetes. Front Physiol. 2019;10:1607.
- Schultz O, Oberhauser F, Saech J, et al. Effects of inhibition of interleukin-6 signalling on insulin sensitivity and lipoprotein (a) levels in human subjects with rheumatoid diseases. PloS one. 2010;5(12):e14328.
- Romeo GR, Lee J, Shoelson SE. Metabolic syndrome, insulin resistance, and roles of inflammation–mechanisms and therapeutic targets. Arterioscler Thromb Vasc Biol. 2012;32(8):1771–1776.
- Saltiel AR, Olefsky JM. Inflammatory mechanisms linking obesity and metabolic disease. J Clin Invest. 2017;127(1):1–4.
- van Hall G, Steensberg A, Sacchetti M, et al. Interleukin-6 stimulates lipolysis and fat oxidation in humans. J Clin Endocrinol Metab. 2003;88(7):3005–3010.
- Wueest S, Konrad D. The controversial role of IL-6 in adipose tissue on obesity-induced dysregulation of glucose metabolism. Am J Physiol Endocrinol Metab. 2020;319(3):E607–e613.
- Patsalos O, Dalton B, Himmerich H. Effects of IL-6 signaling pathway inhibition on weight and BMI: a systematic review and meta-analysis. Int J Mol Sci. 2020;21(17):6290.
- Cookson P. The blind leading the blind. Nurs Mirror Midwives J. 1974;139(22):64–65.
- Gomes KB. IL-6 and type 1 diabetes mellitus: t cell responses and increase in IL-6 receptor surface expression. Ann Transl Med. 2017;5(1):16.
- Marwaha AK, Crome SQ, Panagiotopoulos C, et al. Tan R: cutting edge: increased IL-17-secreting T cells in children with new-onset type 1 diabetes. J Iimmunol. 2010;185(7):3814–3818. Baltimore, Md: 1950.
- Bradshaw EM, Raddassi K, Elyaman W, et al. Monocytes from patients with type 1 diabetes spontaneously secrete proinflammatory cytokines inducing Th17 cells. J Iimmunol. 2009;183(7):4432–4439. Baltimore, Md: 1950.
- Ferreira RC, Freitag DF, Cutler AJ, et al. Functional IL6R 358Ala allele impairs classical IL-6 receptor signaling and influences risk of diverse inflammatory diseases. PLoS Genet. 2013;9(4):e1003444.
- Rosa JS, Oliver SR, Flores RL, et al. Altered inflammatory, oxidative, and metabolic responses to exercise in pediatric obesity and type 1 diabetes. Pediatr Diabetes. 2011;12(5):464–472.
- Bender C, Rajendran S, von Herrath MG. New insights into the role of autoreactive CD8 T cells and cytokines in human type 1 diabetes. Front Endocrinol (Lausanne). 2020;11:606434.
- Lo HC, Lin SC, Wang YM. The relationship among serum cytokines, chemokine, nitric oxide, and leptin in children with type 1 diabetes mellitus. Clin Biochem. 2004;37(8):666–672.
- Pestana RM, Domingueti CP, Duarte RC, et al. Cytokines profile and its correlation with endothelial damage and oxidative stress in patients with type 1 diabetes mellitus and nephropathy. Immunol Res. 2016;64(4):951–960.
- Marasco MR, Conteh AM, Reissaus CA, et al. Interleukin-6 reduces β-cell oxidative stress by linking autophagy with the antioxidant response. Diabetes. 2018;67(8):1576–1588.
- Jablonowska M, Milnerowicz H, Rabczynski J, et al. Immunohistochemical localization of interleukin-6 in human pancreatitis. Appl Immunohistochem Mol Morphol. 2008;16(1):40–43.
- Rajendran S, Anquetil F, Quesada-Masachs E, et al. IL-6 is present in beta and alpha cells in human pancreatic islets: expression is reduced in subjects with type 1 diabetes. Clin Immunol. 2020;211:108320.
- Greenbaum CJ, Serti E, and Lambert K, et al. IL-6 receptor blockade does not slow β cell loss in new-onset type 1 diabetes. JCI Insight. 2021;6(21):e150074.
- Pesce B, Soto L, Sabugo F, et al. Effect of interleukin-6 receptor blockade on the balance between regulatory T cells and T helper type 17 cells in rheumatoid arthritis patients. Clin Exp Immunol. 2013;171(3):237–242.
- Bowker N, Shah RL, Sharp SJ, et al. Meta-analysis investigating the role of interleukin-6 mediated inflammation in type 2 diabetes. EBioMedicine. 2020;61:103062.
- Everett BM, Donath MY, Pradhan AD, et al. Anti-inflammatory therapy with canakinumab for the prevention and management of diabetes. J Am Coll Cardiol. 2018;71(21):2392–2401.
- Ahluwalia TS, Prins BP, Abdollahi M, et al. Genome-wide association study of circulating interleukin 6 levels identifies novel loci. Hum Mol Genet. 2021;30(5):393–409.
- Romagnani P, Remuzzi G, Glassock R, et al. Chronic kidney disease. Nat Rev Dis Primers. 2017;3:17088.
- Institute for Health Metrics and Evaluation (IHME). GBD 2019 cause and risk summary: chronic kidney disease. Seattle [USA]: IHME, University of Washington; 2022. [20 Jan].
- Tuttle KR, Alicic RZ, Duru OK, et al. Clinical characteristics of and risk factors for chronic kidney disease among adults and children: an analysis of the CURE-CKD registry. JAMA Network Open. 2019;2(12):e1918169.
- Andrade-Oliveira V, Foresto-Neto O, Watanabe IKM, et al. Inflammation in renal diseases: new and old players. Front Pharmacol. 2019;10:1192.
- Koye DN, Magliano DJ, Nelson RG, et al. The global epidemiology of diabetes and kidney disease. Adv Chronic Kidney Dis. 2018;25(2):121–132.
- Gross JL, de Azevedo MJ, Silveiro SP, et al. Diabetic nephropathy: diagnosis, prevention, and treatment. Diabetes Care. 2005;28(1):164–176.
- Feigerlová E, Battaglia-Hsu SF. IL-6 signaling in diabetic nephropathy: from pathophysiology to therapeutic perspectives. Cytokine Growth Factor Rev. 2017;37:57–65.
- Alsaad KO, Herzenberg AM. Distinguishing diabetic nephropathy from other causes of glomerulosclerosis: an update. J Clin Pathol. 2007;60(1):18–26.
- Stephens JW, Brown KE, Min T. Chronic kidney disease in type 2 diabetes: implications for managing glycaemic control, cardiovascular and renal risk. Diabetes Obes Metab. 2020;22(Suppl 1):32–45.
- Zoccali C, Vanholder R, Massy ZA, et al. The systemic nature of CKD. Nat Rev Nephrol. 2017;13(6):344–358.
- Araújo LS, Torquato BGS, da Silva CA, et al. Renal expression of cytokines and chemokines in diabetic nephropathy. BMC Nephrol. 2020;21(1):308.
- Taslipinar A, Yaman H, Yilmaz MI, et al. The relationship between inflammation, endothelial dysfunction and proteinuria in patients with diabetic nephropathy. Scand J Clin Lab Invest. 2011;71(7):606–612.
- Su H, Lei CT, Zhang C. Interleukin-6 signaling pathway and its role in kidney disease: an update. Front Immunol. 2017;8:405.
- Barreto DV, Barreto FC, Liabeuf S, et al. Plasma interleukin-6 is independently associated with mortality in both hemodialysis and pre-dialysis patients with chronic kidney disease. Kidney Int. 2010;77(6):550–556.
- Kiberd BA. Interleukin-6 receptor blockage ameliorates murine lupus nephritis. J Am Soc Nephrol. 1993;4(1):58–61.
- Liang B, Gardner DB, Griswold DE, et al. Anti-interleukin-6 monoclonal antibody inhibits autoimmune responses in a murine model of systemic lupus erythematosus. Immunology. 2006;119(3):296–305.
- Ryffel B, Car BD, Gunn H, et al. Interleukin-6 exacerbates glomerulonephritis in (NZB x NZW)F1 mice. Am J Pathol. 1994;144(5):927–937.
- Horii Y, Muraguchi A, Iwano M, et al. Involvement of IL-6 in mesangial proliferative glomerulonephritis. J Iimmunol. 1989;143:3949–3955.
- Kronbichler A, Oh J, Meijers B, et al. Focal segmental glomerulosclerosis: genetics, mechanism, and therapies. Biomed Res Int. 2016;2016:9643785.
- Ridker PM, MacFadyen JG, Glynn RJ, et al. Inhibition of interleukin-1β by canakinumab and cardiovascular outcomes in patients with chronic kidney disease. J Am Coll Cardiol. 2018;71(21):2405–2414.
- Ridker PM, Devalaraja M, Baeres FMM, et al. IL-6 inhibition with ziltivekimab in patients at high atherosclerotic risk (RESCUE): a double-blind, randomised, placebo-controlled, phase 2 trial. Lancet. 2021;397(10289):2060–2069.
- Pergola PE, Devalaraja M, Fishbane S, et al. Ziltivekimab for treatment of anemia of inflammation in patients on hemodialysis: results from a phase 1/2 multicenter, randomized, double-blind, placebo-controlled trial. J Am Soc Nephrol. 2021;32(1):211–222.
- Nemeth E, Rivera S, Gabayan V, et al. IL-6 mediates hypoferremia of inflammation by inducing the synthesis of the iron regulatory hormone hepcidin. J Clin Invest. 2004;113(9):1271–1276.
- Jelkmann W. Proinflammatory cytokines lowering erythropoietin production. J Interferon Cytokine Res. 1998;18(8):555–559.
- Garimella PS, Katz R, Patel KV, et al. Association of serum erythropoietin with cardiovascular events, kidney function decline, and mortality: the health aging and body composition study. Circ Heart Fail. 2016;9(1):e002124.
- Ridker PM, Rane M. Interleukin-6 signaling and Anti-interleukin-6 therapeutics in cardiovascular disease. Circ Res. 2021;128(11):1728–1746.
- Roth GA, Mensah GA, Johnson CO, et al. Global burden of cardiovascular diseases and risk factors, 1990-2019: update from the GBD 2019 study. J Am Coll Cardiol. 2020;76(25):2982–3021.
- Broch K, Anstensrud AK, Woxholt S, et al. Randomized trial of interleukin-6 receptor inhibition in patients with acute ST-segment elevation myocardial infarction. J Am Coll Cardiol. 2021;77(15):1845–1855.
- Libby P, Buring JE, Badimon L, et al. Atherosclerosis. Nat Rev Dis Primers. 2019;5(1):56.
- Martinez BK, White CM. The emerging role of inflammation in cardiovascular disease. Ann Pharmacother. 2018;52(8):801–809.
- Martínez GJ, Celermajer DS, Patel S. The NLRP3 inflammasome and the emerging role of colchicine to inhibit atherosclerosis-associated inflammation. Atherosclerosis. 2018;269:262–271.
- Montarello NJ, Nguyen MT, Wong DTL, et al. Inflammation in coronary atherosclerosis and its therapeutic implications. Cardiovasc Drugs Ther. 2020. https://doi.org/10.1007/s10557-020-07106-6
- Ridker PM, Libby P, MacFadyen JG, et al. Modulation of the interleukin-6 signalling pathway and incidence rates of atherosclerotic events and all-cause mortality: analyses from the Canakinumab Anti-Inflammatory Thrombosis Outcomes Study (CANTOS). Eur Heart J. 2018;39(39):3499–3507.
- Ridker PM, MacFadyen JG, Everett BM, et al. Relationship of C-reactive protein reduction to cardiovascular event reduction following treatment with canakinumab: a secondary analysis from the CANTOS randomised controlled trial. Lancet. 2018;391(10118):319–328.
- Aimo A, Pascual-Figal DA, Barison A, et al. Colchicine for the treatment of coronary artery disease. Trends Cardiovasc Med. 2020;31(8):497–504.
- Nidorf SM, Fiolet ATL, Mosterd A, et al. Thompson PL: colchicine in patients with chronic coronary disease. N Engl J Med. 2020;383(19):1838–1847.
- Boland J, Long C. Update on the inflammatory hypothesis of coronary artery disease. Curr Cardiol Rep. 2021;23(2):6.
- Ridker PM. Inhibiting interleukin-6 to reduce cardiovascular event rates: a next step for atherothrombosis treatment and prevention. J Am Coll Cardiol. 2021;77(15):1856–1858.
- Ridker PM. From CANTOS to CIRT to COLCOT to clinic: will all atherosclerosis patients soon be treated with combination lipid-lowering and inflammation-inhibiting agents? Circulation. 2020;141(10):787–789.
- Ridker PM. From C-reactive protein to interleukin-6 to interleukin-1: moving upstream to identify novel targets for atheroprotection. Circ Res. 2016;118(1):145–156.
- Hartman J, Frishman WH. Inflammation and atherosclerosis: a review of the role of interleukin-6 in the development of atherosclerosis and the potential for targeted drug therapy. Cardiol Rev. 2014;22(3):147–151.
- Neumann FJ, Ott I, Marx N, et al. Effect of human recombinant interleukin-6 and interleukin-8 on monocyte procoagulant activity. Arterioscler Thromb Vasc Biol. 1997;17(12):3399–3405.
- Alsaffar H, Martino N, Garrett JP, et al. Interleukin-6 promotes a sustained loss of endothelial barrier function via Janus kinase-mediated STAT3 phosphorylation and de novo protein synthesis. Am J Physiol Cell Physiol. 2018;314(5):C589–c602.
- Romano M, Sironi M, Toniatti C, et al. Role of IL-6 and its soluble receptor in induction of chemokines and leukocyte recruitment. Immunity. 1997;6(3):315–325.
- Schuett H, Oestreich R, Waetzig GH, et al. Transsignaling of interleukin-6 crucially contributes to atherosclerosis in mice. Arterioscler Thromb Vasc Biol. 2012;32(2):281–290.
- Zhang K, Huang XZ, Li XN, et al. Interleukin 6 destabilizes atherosclerotic plaques by downregulating prolyl-4-hydroxylase α1 via a mitogen-activated protein kinase and c-Jun pathway. Arch Biochem Biophys. 2012;528(2):127–133.
- Ridker PM, Cushman M, Stampfer MJ, et al. Inflammation, aspirin, and the risk of cardiovascular disease in apparently healthy men. N Engl J Med. 1997;336(14):973–979.
- Ridker PM, Hennekens CH, Buring JE, et al. C-reactive protein and other markers of inflammation in the prediction of cardiovascular disease in women. N Engl J Med. 2000;342(12):836–843.
- Ridker PM, Buring JE, Cook NR, et al. C-reactive protein, the metabolic syndrome, and risk of incident cardiovascular events: an 8-year follow-up of 14 719 initially healthy American women. Circulation. 2003;107(3):391–397.
- Sarwar N, Butterworth AS, and Freitag DF, et al. Interleukin-6 receptor pathways in coronary heart disease: a collaborative meta-analysis of 82 studies. Lancet. 2012;379:1205–1213. .
- Anagnostakos J, Lal BK. Abdominal aortic aneurysms. Prog Cardiovasc Dis. 2021;65:34–43.
- Rohde LE, Arroyo LH, Rifai N, et al. Plasma concentrations of interleukin-6 and abdominal aortic diameter among subjects without aortic dilatation. Arterioscler Thromb Vasc Biol. 1999;19(7):1695–1699.
- Thompson RW, Geraghty PJ, Lee JK. Abdominal aortic aneurysms: basic mechanisms and clinical implications. Curr Probl Surg. 2002;39(2):110–230.
- Shteinberg D, Halak M, Shapiro S, et al. Abdominal aortic aneurysm and aortic occlusive disease: a comparison of risk factors and inflammatory response. Eur J Vasc Endovasc Surg. 2000;20(5):462–465.
- Harrison SC, Smith AJ, Jones GT, et al. Interleukin-6 receptor pathways in abdominal aortic aneurysm. Eur Heart J. 2013;34(48):3707–3716.
- Tang W, Yao L, Hoogeveen RC, et al. The association of biomarkers of inflammation and extracellular matrix degradation with the risk of abdominal aortic aneurysm: the ARIC study. Angiology. 2019;70(2):130–140.
- Zhang Y, Xu G, Wang P. Smoking, hypertension, and GG genotype of the IL-6 rs1800796 polymorphism are independent risk factors for Abdominal Aortic Aneurysm in Han population. Pharmgenomics Pers Med. 2021;14:1115–1121.
- Kokje VBC, Gäbel G, Koole D, et al. IL-6: a Janus-like factor in abdominal aortic aneurysm disease. Atherosclerosis. 2016;251:139–146.
- Fernandez-Real JM, Vayreda M, Richart C, et al. Circulating interleukin 6 levels, blood pressure, and insulin sensitivity in apparently healthy men and women. J Clin Endocrinol Metab. 2001;86(3):1154–1159.
- Vázquez-Oliva G, Fernández-Real JM, Zamora A, et al. Lowering of blood pressure leads to decreased circulating interleukin-6 in hypertensive subjects. J Hum Hypertens. 2005;19(6):457–462.
- Hirota H, Yoshida K, Kishimoto T. Taga T: continuous activation of gp130, a signal-transducing receptor component for interleukin 6-related cytokines, causes myocardial hypertrophy in mice. Proc Natl Acad Sci U S A. 1995;92(11):4862–4866.
- Meléndez GC, McLarty JL, and Levick SP, et al. Interleukin 6 mediates myocardial fibrosis, concentric hypertrophy, and diastolic dysfunction in rats. Hypertension. . 2010;56(2):225–231.
- Peng C, Stewart AG, Woodman OL, et al. Non-alcoholic steatohepatitis: a review of its mechanism, models and medical treatments. Front Pharmacol. 2020;11:603926.
- Younossi Z, Anstee QM, Marietti M, et al. Global burden of NAFLD and NASH: trends, predictions, risk factors and prevention. Nat Clin Pract Gastroenterol Hepatol. 2018;15(1):11–20.
- Younossi ZM, Golabi P, de Avila L, et al. The global epidemiology of NAFLD and NASH in patients with type 2 diabetes: a systematic review and meta-analysis. J Hepatol. 2019;71(4):793–801.
- Kasper P, Martin A, Lang S, et al. NAFLD and cardiovascular diseases: a clinical review. Clin Res Cardiol. 2020;110(7):921–937.
- Sanyal AJ. Past, present and future perspectives in nonalcoholic fatty liver disease. Nat Clin Pract Gastroenterol Hepatol. 2019;16(6):377–386.
- Schuster S, Cabrera D, Arrese M, et al. Triggering and resolution of inflammation in NASH. Nat Clin Pract Gastroenterol Hepatol. 2018;15:349–364.
- Wieckowska A, Papouchado BG, Li Z, et al. Increased hepatic and circulating interleukin-6 levels in human nonalcoholic steatohepatitis. Am J Gastroenterol. 2008;103(6):1372–1379.
- Newsome PN, Buchholtz K, and Cusi K, et al. A Placebo-controlled trial of subcutaneous semaglutide in nonalcoholic steatohepatitis. N Engl J Med. 2020;384(12): 1113–1124.
- Lefere S, Devisscher L, Tacke F. Targeting CCR2/5 in the treatment of nonalcoholic steatohepatitis (NASH) and fibrosis: opportunities and challenges. Expert Opin Investig Drugs. 2020;29(2):89–92.
- Ratziu V, Sanyal A, Harrison SA, et al. Cenicriviroc treatment for adults with nonalcoholic steatohepatitis and fibrosis: final analysis of the phase 2b CENTAUR study. Hepatology. 2020;72(3):892–905.
- Yamaguchi K, Itoh Y, Yokomizo C, et al. Blockade of interleukin-6 signaling enhances hepatic steatosis but improves liver injury in methionine choline-deficient diet-fed mice. Lab Invest. 2010;90(8):1169–1178.