ABSTRACT
Introduction
Trained Immunity (TI) refers to the long-term modulation of the innate immune response, based on previous interactions with microbes, microbial ligands, or endogenous substances. Through metabolic and epigenetic reprogramming, monocytes, macrophages, and neutrophils develop an enhanced capacity to mount innate immune responses to subsequent stimuli and this is persistent due to alterations at the myeloid progenitor compartment.
Areas covered
The purpose of this article is to review the current understanding of the TI process and to discuss its potential clinical implications in the near future. We address the evidence of TI involvement in various diseases, the currently developed new therapy, and discuss how TI may lead to new clinical tools to improve existing standards of care.
Expert opinion
The state of the art in this domain has made considerable progress, linking TI-related mechanisms in multiple immune-mediated pathologies, starting with infections to autoimmune disorders and cancers. As a relatively new area of immunology, it has seen fast progress with many of its applications ready to be investigated in clinical settings.
1. Trained immunity
The classical innate immune response at a cellular level starts with Pattern Recognition Receptors (PRRs) [Citation1], proteins capable of detecting various stimuli classified into Damage Associated and Pathogen Associated Molecular Patterns (DAMPs and PAMPs) [Citation2]. Their recognition leads to the activation of pro-inflammatory pathways, such as the canonical NF-kB pathway, which induces the production of cytokines and chemokines, increased cell survival, and immune cell differentiation [Citation3]. After an initial stimulation of certain PRRs, the functional status of innate immune cells is altered, with specific epigenetic marks and changes in the cellular metabolism, that persist even after the initial immune response is over. This will be further referred to as trained immunity (TI) and cells that possess this phenotype show a more potent immune response upon re-stimulation with the same or different pathogens [Citation4,Citation5] (). Therefore, TI represents the memory of the innate immune response, characterized by a long-term effect of initial stimulations in which cells respond with a higher intensity to subsequent stimulation with DAMPs or PAMPs [Citation6]. It is also a form of heterologous immunity strictly restricted to the innate branch of the immune response, in contrast to other adaptive mechanisms such as heterologous T-cell priming.
Figure 1. A simplified schematic representation of TI. The innate immune system (purple lines) is activated by engagement of PRRs, which is followed by downstream signaling pathways, such as the NF-kB pathway, leading to transcriptional activation of pro-inflammatory genes and release of cytokines and chemokines. On the first interaction (a) with a PAMP/DAMP, an interplay between metabolic and epigenetic changes induces the trained phenotype (blue lines). Upon the second interaction (b), a more potent cytokine response will be observed, due to the functional epigenetic reprogramming of the cell.
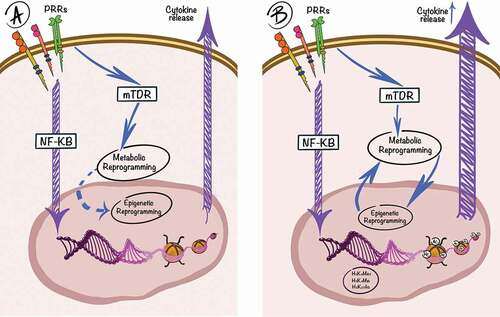
In the context of TI, the most extensively studied inducers are the Bacillus Calmette–Guérin (BCG) vaccine and β-glucan, isolated from Candida albicans. The BCG vaccine was extremely useful in TI research due to its widespread use against Mycobacterium tuberculosis infections, which resulted in significant amounts of clinical data and more opportunity for research in humans. Even though BCG presents a multitude of PAMPs, the TI phenotype was shown to be induced by the NOD2 receptor [Citation7], which binds to muramyl dipeptide (MDP). The BCG-trained cells show increased aerobic glycolysis, oxidative phosphorylation, and glutaminolysis, changes induced through the Akt/mTOR/HIF1α pathway [Citation8]. Associated epigenetic marks are represented by increased trimethylation of at the fourth lysine of the H3 histone (H3K4me3) and decreased H3K9me3 at the promoters of mTOR, HK2, PFKP, and GLS, with a synergistic effect of increased transcription for said genes, plus an increased H3K4me3 at TNFA and IL6 promoters and H3K27ac in multiple genes in AKT, EGFR, FGF, and VEGF pathways [Citation9].
β-glucan is recognized by the Dectin-1 and Complement Receptor 3, which in turn activate the NF-kB pathway with all its associated effects [Citation10]. A lot of similar findings regarding metabolic and epigenetic changes were described in β-glucan induced TI, such as the activation of the mTOR pathway, increased glycolysis, and H3K4me3 and H3K27Ac epigenetic marks [Citation11].
The currently proposed mechanism for H3K4me3 involves the WDR5/MLL1 methyltransferase complex guided by immune priming long non-coding RNAs (IPLs) [Citation12]. Following BCG vaccination, the levels of IPLs UMLILO and IPL-IL1, which direct H3K4me3 at IL-8 and IL-1β promoters, were increased in human neutrophils. The cells with a higher expression of IPLs also had an increased activity [Citation13]. As for other methylation marks, the Lysine Methyltransferase Set7, which induces H3K4me1, was identified as a key driver of TI, and several polymorphisms in the SETD7 gene were associated with TI response change [Citation14]. Another Methyltransferase that plays a significant role in TI response is G9a, responsible for H3K9 methylation, which is associated with transcriptional downregulation of the methylated region. G9a is downregulated in trained monocytes and its pharmacological inhibition promoted the TI phenotype [Citation15].
The current knowledge about metabolic and epigenetic changes in TI were extensively reviewed previously [Citation16–18]. In brief, the rate of metabolic processes in trained cells is changed in order to meet the new energetic requirements. More importantly, the accumulation of certain metabolites induces specific epigenetic changes in TI. In turn, these epigenetic programs lead to altered expression of metabolic enzymes, ultimately maintaining the same metabolic circuits. An example of such an interaction is the accumulation TCA cycle metabolites, fumarate, succinate, and malate, which lead to the inhibition of α-ketoglutarate-dependent demethylases. In trained cells, one of such demethylases – KDM5 – is inhibited, a process that is directly linked to increased H3K4me3 [Citation19]. The accumulation of Acetyl-CoA due to increased glycolysis can increase the activity of acetyltransferases [Citation20]. In trained cells, the increased level of nicotinamide adenine dinucleotide (NAD+) stimulates the activity of sirtuins, a group of enzymes with important effects on immune responses [Citation21]. Additionally, the previously mentioned Set7 methyltransferase is necessary for the accumulation of TCA cycle metabolites.
The TI phenotype was also confirmed to be induced by PAMPs and DAMPs such as LPS, C. albicans [Citation4], poly I:C, oxidized LDL, Lipoprotein A, and others [Citation22–24]. Even though the trained phenotype was established in these cases, the pathways involved and the metabolic changes can be different. For example, in β-glucan induced TI, oxidative phosphorylation is reduced, unlike the case with BCG. Similarly, Reactive Oxygen Species (ROS) production is increased in BCG and oxLDL training, while unchanged upon stimulation with β-glucan [Citation22]. However, stimuli such as LPS [Citation25], C. albicans [Citation26], or poly I:C [Citation27] can also induce immune tolerance, a phenomenon that can be viewed as the opposite of training. The shift between training and tolerance was shown to be dose-dependent [Citation23]. These dual effects of stimuli may also be time dependent: despite a broad association of sepsis with post-septic immunosuppression because of innate immune tolerance, in a polymicrobial sepsis mouse model, bone marrow monocytes had a TI phenotype and depicted higher innate immune responses at 3 months after sepsis [Citation28]. An interesting finding is that while trained cells can accumulate lactate, it was shown to posses anti-inflammatory effects on PBMCs, Monocytes [Citation29] and Macrophages [Citation30] through histone lactylation. This highlights that the mechanisms of immune training and tolerance are interlinked in a complex interaction between metabolic and epigenetic factors.
Some effects of the TI protection were confirmed to last from 3 months up to 1 year [Citation31], and a nonspecific protective effect of the BCG vaccine against other infections potentially even lasts up to 5 years [Citation32]. The presence of a long-term effect is due to the involvement of Hematopoietic Stem Cells (HSCs), a finding initially confirmed in mice as β-glucan was able to induce a shift toward myelopoiesis and metabolic changes in HSCs [Citation33]. A later study confirmed similar findings in BCG-vaccinated healthy volunteers, highlighting the importance of HNF1A and HNF1B as Transcription Factors in BCG-induced TI and proposed S100A12, CCL20, and CCL23 as predictive serum biomarkers for TI [Citation34]. Of note, the capacity to develop TI was observed in other cell types, such as fibroblasts, microglial cells [Citation35], epithelial [Citation36], and even smooth muscle cells [Citation37], most likely due to their expression of PRRs.
The TI effect can play dual roles in the pathophysiology of immune-mediated diseases, the understanding of which is likely to bring new avenues for personalized medicine approaches in the future. On the one hand, harnessing TI to prevent or treat infections and immunodeficiencies or to promote anti-tumor processes is a strategy for TI-deficient states. On the other hand, the hyperinflammatory states that are associated with TI contribute to autoimmune and autoinflammatory diseases (). Subject to future research findings, the knowledge on TI will provide us with tools to help in the prevention and therapy of immune-mediated diseases where TI is either defective or exacerbated. In the following sections, we will review the evidence that supports the implications of TI in clinical situations and outline future directions of development toward practical clinical tools based on TI.
Figure 2. Implications of TI in disease and possible TI-based interventions. In some pathology, the immune response is skewed – for example in Autoimmune disorders or Atherosclerosis, a therapeutic goal would be to generally reduce the reactivity of the immune system. Immunosuppression is a useful tool in transplantation in order to avoid rejection. A boost in immunity is favorable in cancer and potentially immunodeficient states. In the case of vaccination, TI usually acts as a nonspecific protective effect, but immunomodulation can also be an important tool to combat specific diseases.
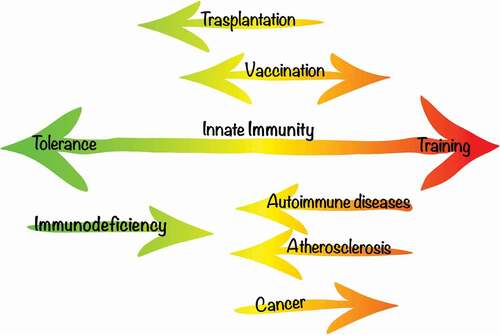
2. Vaccination
An important body of research addressing the clinical relevance of TI is directed toward the development and improvement of vaccines. By inducing TI, it is possible to ensure a stronger innate immune response that will offer a better protection against the targeted pathogen and cross-protection against other infections. The heterologous effects of vaccines have been previously reviewed [Citation38,Citation39], with most data available for the BCG vaccine.
Besides the expected effect against tuberculosis, the BCG vaccine was shown to possess a protective role against C. albicans [Citation40], S. aureus [Citation41], malaria [Citation42], as well as viral infections like herpes [Citation43], yellow fever [Citation9], HPV [Citation44] and a general protection against acute upper [Citation45] and lower respiratory tract infections [Citation46]. These effects can be explained by both innate and adaptive heterologous immunity; however, the cross-protective effect of BCG was observed in T- and B-cell-deficient mice [Citation7], confirming TI as a key player. An unexpected effect of the BCG vaccination, which highlights its immunomodulatory effects and the complexity of immune interactions, is the protection against Type I Diabetes and Multiple Sclerosis, currently explained by TNF induced autoreactive T cell death [Citation47].
The use of BCG as a vector for recombinant vaccines started over 30 years ago and has shown great promise in multiple studies. Its use presents high interest due to its good safety profile and low manufacturing cost. VPM1002 is a recombinant BCG vaccine obtained by replacing the C gene with hly, encoding listeriolysin. It is a potential candidate to replace its predecessor as an improvement, and is currently in a phase 3 clinical trial for Tuberculosis prevention in infants (NCT04351685). Another recombinant vaccine that presents high interest is MTBVAC, a live attenuated M. tuberculosis vaccine, obtained through deletion of the phoP and fadD26 genes. MTBVAC is also in phase 3 trials for TB treatment (NCT04975178), and initial studies suggest that it has a significantly higher efficacy compared to BCG [Citation48]. When tested for TI effects, MTBVAC induced training in human monocytes, with higher pro-inflammatory cytokine release upon re-stimulation, metabolic reprogramming, H3K4me3 at TNFΑ and IL6 gene promoters, and in mouse models, cross-protection against S. pneumoniae was confirmed [Citation49].
The development of rBCG vaccines is an approach currently used in respiratory viral infections such as human Respiratory Syncytial Virus (hRSV) and Metapneumovirus (hMPV). After multiple unsuccessful attempts in the development of vaccines against hRSV, the rBCG vaccines that express the nucleoprotein (N) or the M2-1 protein have shown good results. rBCG-N-hRSV passed a phase 1 clinical trial, showing an adequate immune response and no safety issues reported 30 days post-vaccination [Citation50]. The rBCG-P (hMPV phosphoprotein) vaccine is still in preclinical phase and was shown to induce protective immunity in mice [Citation51]. In HIV research, multiple strains of rBCG and combinations were developed that produce a CD8 + T immune response in mice. A recombinant vaccine based on MTBVAC to combat HIV-1 has also been reported [Citation52].
In 2020, the idea that the BCG vaccine might have a protective role against COVID-19 pandemic was proposed [Citation53]. At the same time, another group showed that after accounting for several confounders, the epidemiological data does not support this hypothesis [Citation54]. A definitive answer regarding this question is expected soon as multiple clinical trials studying BCG as a prevention measure against COVID-19 are approaching their final phase (NCT04348370, NCT04327206, NCT04328441, NCT04379336, NCT04417335). VPM1002 is similarly investigated for COVID-19 prevention (NCT04439045, NCT04387409, NCT04435379). A recent study finds that influenza vaccination has immunomodulatory/suppressive effects and changes the response to SARS-CoV-2 and other viral stimuli [Citation55]. Whether this effect plays a positive or negative role in the severity of Covid-19 is still a subject for discussion and confidently asserting that it is a challenge. The idea that the immune response in coronavirus infections is pathologically upregulated is also supported by the fact that MERS-CoV infected endothelial cells show signs of trained immunity, such as increased proinflammatory cytokine expression, downregulated OXPHOS, accumulation of ROS, and epigenetic reprogramming [Citation56].
The TI model can also explain the efficacy of multibacterial formulas and bacterial lysates in nonspecific respiratory tract infections (RTIs). MV-130, a heat-inactivated bacterial mix, was confirmed to induce TI in monocytes and HSCs, through the activation of the mTOR pathway and provide cross-protection against viral infections in mice [Citation57], including SARS-CoV-2 [Citation58]. The bacterial lysate, OM-85 has similarly shown TI cross-protection properties against murine coronavirus, triggering a stronger type 1 IFN response [Citation59]. Multiple lysate formulas are available, with a considerable number displaying nonspecific protection in RTIs [Citation60].
As research regarding TI continues, it becomes clearer that evaluating and formulating guidelines for vaccine use that also account for nonspecific effects and cross-protection from other pathogens is important. This approach allows to significantly improve existing vaccines by considering their immunomodulatory effects, and for diseases in which an efficient vaccine has not yet been developed, the nonspecific protection developed by innate immune training can become a temporary solution. As reviewed elsewhere [Citation61], the addition of an ‘amplifier,’ which represents an epigenetic and/or metabolic modulator, to the classic vaccine formulas could increase their efficacy. The vaccines that contain TI inducers are also referred to as Trained Immunity-based Vaccines [Citation62].
3. Cancer
Intravesical BCG vaccine administration was used to treat non-muscle-invasive bladder cancer (NIBC) since the 1960s [Citation63]. The intravesical application of BCG is seen as the first immunotherapy of cancer. It is currently part of the standard of care in the treatment and recurrence prevention of stage I and II NIBC, alongside gemcitabine, which has similar effectiveness [Citation64,Citation65]. The primary mechanism driving this involves important local effects of BCG on the urothelium and, more specifically, the tumor microenvironment. BCG possesses tropism to fibronectin [Citation66], found mostly on damaged urothelium, where the cancer is located to produce an immune response. The resolution of the carcinoma requires the involvement of local macrophages, which are activated through the MyD88/NF-kB signaling pathway [Citation67]. The resulting cytokine release is later followed by recruitment of CD8+ T cells, NK cells, neutrophils, macrophages, and other cell types [Citation68]. Besides the local interactions, important systemic effects suggestive of TI have been noted even since the 90s, when a pro-inflammatory phenotype was observed in blood circulating monocytes after BCG instillations [Citation69]. Furthermore, the second and following instillations elicit a stronger immune response with a higher release of urinary IL-1, IL-2, IL-6, and TNF [Citation70]. The currently proposed TI mechanism in BCG immunotherapy is that the systemic training effects will result in a trained population of circulating monocytes and macrophages, following an increased release of cytokines that will facilitate the activation of T cells [Citation71].
TI is strongly linked to autophagy, since β-glucan training induces transcription of autophagy-related genes and the inhibition of autophagy blocks trained immunity in vitro in response to BCG or β-glucan [Citation72]. When examining SNPs in autophagy-related genes, rs3759601 in ATG2B was shown to change the TI response without affecting the initial immune response, and more importantly, the SNP correlates with NIBC progression and recurrence [Citation72]. This evidence shows that TI plays a role in BCG therapy and an ongoing clinical study (NTC03091660) set to investigate the outcome of intradermal BCG priming before the intravesical therapy should provide more information regarding the importance of the systemic TI effect.
In support of the TI-induced protection against cancer, it can also be mentioned that BCG immunization reduces the risk of lung cancer [Citation73], leukemia [Citation74], and lymphomas [Citation75], and intralesional BCG administration is also used for a long time as therapy in stage III–IV for melanomas. Multiple trials testing the use of BCG as an adjuvant for chemotherapy or tumor cell vaccines have shown good results [Citation76].
A field that currently shows great promise is nanobiologic therapy. It allows for more precise targeting of specific tissues in order to avoid unwanted side-effects. Multiple methods to deliver BCG with nanoparticles were explored. One of them is the use of BCG cell wall skeleton encapsulated in lipid particles (BCG-CWS) [Citation77], a drug that was also proposed as an adjuvant for other types of cancer [Citation78]. MTP10-HDL is derived from muramyl dipeptide, capable of inducing TI, with apolipoprotein A1 as a carrier molecule, which is used for hematopoietic stem cells, multipotent progenitors, and myeloid progenitors targeting. MTP10-HDL was able to successfully induce TI in innate immune cells and inhibit tumor growth in a mouse melanoma model [Citation79].
4. Inflammatory and autoimmune disorders
The fact that TI can lead to inflammatory consequences has best been illustrated to date in the context of atherosclerosis. Older evidence in rodents already showed that BCG administration is positively correlated to atherosclerosis [Citation80], while in vitro training of monocytes with beta-glucan induced transcriptional activation of proinflammatory and proatherogenic genes [Citation4]. After the discovery that oxLDL can train human primary monocytes, the role of TI in the development of atherosclerosis received more attention. Known hallmarks of TI were identified in monocytes such as the activation of the mTOR pathway [Citation81] or characteristic epigenetic and metabolic changes [Citation82]. Many studies show that a high-fat diet, which is a known risk factor for atherosclerosis, induces a pro-inflammatory phenotype in monocytes and HSCs [Citation83], which plays an important role in the progression of atherosclerotic lesions [Citation84,Citation85]. Similarly, an increased level of glucose in diabetes, a pathology often associated with atherosclerosis, leads to increased glycolysis and a pro-inflammatory phenotype in monocytes, an effect driven by Runt-related transcription factor (RUNX) 1 [Citation86]. As it was already mentioned, the involvement of HSCs increases the effective duration of immune cell training and this was confirmed in the context of atherosclerosis. When bone marrow from diabetic mice was transplanted to atherosclerosis prone normoglycemic ones, the progression of plaques was significantly accelerated [Citation87].
In addition to the implications for atherosclerosis and cardiovascular diseases, TI can predispose and aggravate other inflammatory and autoimmune diseases as well. Current circumstantial evidence has already pointed out several features of innate immune responses in autoinflammatory and autoimmune conditions, which are shared with trained immunity, also reviewed by Arts et al. [Citation88]. Several such examples are outlined below.
Rheumatoid arthritis (RA), the most common autoimmune disease, is characterized by circulating autoantibodies and synovitis with progressive cartilage and bone damage. Anti-parietal cell antibodies often precede clinical manifestations and were shown to induce TI phenotype in monocytes [Citation89]. The hyperinflammatory status of circulating monocytes was established in several studies, which confirmed an increased cytokine response and hypermetabolic status with increased glycolysis induced by STAT3 [Citation90,Citation91]. In contrast, another study reported that H3K4me3 was not increased at TNFA and IL6 promoters in RA patients [Citation92]. The targeting of P13K/Akt/mTOR pathway in RA is already a topic of scientific research for some time. Rapamycin was tested in humans and combined with conventional RA therapy reduces the disease activity [Citation93] and its combination in nanoparticles also showed good results in RA mice models [Citation94].
In Systemic Lupus Erythematosus (SLE), besides the well-known presence of autoantibodies and autoreactive T cell activity, more and more evidence indicates the involvement of innate immunity as a major player in the complex interactions characteristic for this disease [Citation95]. Even though the link between SLE and TI has not been formally established, certain similar features point in that direction, such as the mTOR pathway activation [Citation96] or the epigenetic profile of monocytes from patients with SLE compared to trained monocytes (enrichment in H3K4Me3 [Citation97] and H4 acetylation [Citation98]). In addition, functional reprogramming at the HSCs level has been reported based on transcriptomic assessment of bone marrow samples in patients with SLE and controls, suggestive of myeloid skewing similarly to a TI signature [Citation99].
Gout is an inflammatory disease caused by deposits of Monosodium Urate (MSU) crystals in joints and is usually preceded by increased serum urate concentrations. MSU is a DAMP that triggers an immune response dependent on the NLRP3 inflammasome activation [Citation100]. Interestingly, urate was reported to depict antitumoral properties [Citation101] and was proposed as an adjuvant for the BCG vaccine [Citation102]. Soluble urate was also shown to alter the transcription and proinflammatory cytokine production profile in primary human peripheral mononuclear cells [Citation103,Citation104] by activating the Akt/mTOR pathway [Citation105]. Conversely, conflicting results evoking anti-inflammatory properties of soluble urate were reported in monocytes [Citation106], which could be explained with differences in methodology.
5. Transplantation
Pharmacological immunosuppression is an important tool that greatly improved the outcomes of organ transplantation, but its long-term use is associated with major adverse effects. Because of that, multiple alternatives are currently being explored. Targeting TI is an important avenue to explore, due to the fact that macrophages play a crucial role in allograft rejection by initiating the CD4+ T cell response [Citation107].
As previously mentioned, precise targeting of immune cells is possible with nanobiologics, so multiple attempts at developing immunosuppressants were made using this technology. These include H3K27 demethylase inhibitor GSK-J4 or inhibition of ROS production with diethyl malonate, while the most promising avenue to explore was mTOR pathway inhibition with rapamycin [Citation108]. In 2018, a combination of mTORi-HDL and TRAF6i-HDL was developed and tested on mice with promising results, inhibiting TI in macrophages, and reducing organ transplant rejection [Citation109].
6. Future clinical implications of trained immunity
To summarize, TI represents the adaptation of innate immune cells upon encounter with a stimulus that elicits an innate response and also leads to persistent metabolic and epigenetic alterations. This facilitates a faster and stronger inflammatory response to a subsequent challenge with either the same or a different stimulus. The long-term persistence of TI is ensured by the presence and the mitotic heritability of these epigenetic changes at the level of progenitor cells. Different stimuli may rewire cells to different epigenetic and transcriptional programs, which may variably impact the host, depending on their own susceptibility or underlying pathology. In the coming years, the further characterization of these trained immunity features will probably represent an important scientific goal before developing clinically valid and useful TI-based strategies. Relevant open questions to be clarified include which stimuli can lead to TI, how fast is TI induced or erased, how long does TI last, and how is TI passed on during cell division or trans generationally. Fundamental knowledge in this field is, on the one hand, likely to inform the development of clinical tools contributing to personalized preventive strategies and risk assessment, while, on the other, lead to therapeutic developments aimed at either inducing or inhibiting trained immunity.
Therapeutically, TI can be targeted for inhibition at several levels – PRRs, mTOR pathway, metabolic, and epigenetic levels. Rapamycin is in use as an immunosuppressor since 1999, and the development of nanobiologics will likely lead to a new generation of drugs in this class. Precise targeting of myeloid cells is expected to reduce the toxic systemic effects of mTOR inhibitors. In a similar fashion, but with the opposite effect, the MTP10-HDL nanobiologic is a candidate drug to enhance the immune response. These two drugs may become important opposing therapies aimed at modulating trained immunity. Moreover, epigenetic marks could be targeted through enzymes, such as methyltransferases and demethylases. An example of such drugs is 3-Deazaneplanocin A, which can inhibit H3K27me3 with effects that are in line with our expectations, such as inducing apoptosis [Citation110], reduction of cellular growth, and migration [Citation111]. These might prove an important alternative to explore but are most likely to be inferior, due to off-target secondary effects. The alternative epigenetic approach is to target IPLs that participate in the setting of specific histone marks associated with TI.
The immune boosters may improve the clinical outcomes in all types of cancer and allow more therapeutic options. For example, the use of general anesthesia is avoided in oncologic surgeries when possible due to its immunosuppressive effect [Citation112,Citation113] and canceling this effect presents high clinical interest. Immunotherapy is an avenue that is currently widely explored in cancer and, if nanobiologics prove successful in humans, they will become a feasible solution that might partially replace chemotherapy. A general boost in immunity could also prove useful in elderly as prophylaxis [Citation114].
Along the same line, it can be envisioned that the vaccination process will be impacted by TI-based approaches, as more clinical data on the efficiency of recombinant vaccines and immune boosters will be released. Various TI inducing DAMPs and PAMPs can be used as adjuvants, offering a major improvement over existing vaccines.
Assessing the TI status in patients can provide useful information to guide medical decisions. The identification of reliable biomarkers for TI may potentially help stratify patients into risk groups for strong or weak immune responses in the context of different pathologies. Feasible combinations of biomarkers may include cytokines, chemokines, or other inflammatory proteins (such as S100A12, CCL20, and CCL23 [Citation34]), as well as relevant IPLs (UMLILO and IPL-IL1 [Citation13]) and quantification of specific histone mark enrichment at relevant gene regions (H3K4Me3).
Assessing the TI status can be useful in order to establish if it is a good target for medication and further monitor the patient’s response. In inflammatory disorders, signs of TI often precede clinical symptoms [Citation83,Citation90]; therefore, a TI dysregulation can be indicative of a disease onset or increased susceptibility.
In addition to markers of TI, predictive models for the cytokine production capacity have been reported, and genetic variation was shown to be the main determinant [Citation115]. Several polymorphisms that influence the immune response and some that are specifically associated to TI have been previously mentioned [Citation14]. This suggests that integrating genetic susceptibility variants into stratification tools for people that may show variable TI phenotypes may prove useful for risk management strategies.
In conclusion, the data summarized in this review illustrate the involvement of TI in important pathophysiological processes and show the significant advances in targeting TI to alter disease outcome. Further knowledge into TI mechanisms and sources of variability will make it increasingly likely to translate this knowledge into clinically relevant tools for prevention and therapeutics.
7. Expert opinion
Over a decade ago, the concept of trained immunity was introduced and expanded our understanding of immunological memory. The TI phenotype is linked to immunometabolic and epigenetic changes that determine the transcriptional program of trained cells upon reinfection or subsequent challenge with sterile stimuli. These changes are passed on from Hematopoietic Stem Cells to circulating monocytes and macrophages, ensuring a systemic and relatively long-lasting effect. It is still difficult to determine the extent to which an in vivo innate immune response is the result of TI, and this is the subject of current research. Some degree of immunomodulation due to TI processes is most likely part of every immune response since hosts encounter pathogens at every step, with every interaction triggering an immune response, which in turn influences subsequent responses. The molecular markers of TI are seen in many cell types, and the involved pathways are mostly metabolic, part of the normal function of any cell. From this perspective, TI can also be seen as an evolutionarily acquired additional effect of the accelerated metabolism driven by enhanced glycolysis in immune cells. Therefore, it is important to recognize that many known pathways are interlinked and can drive additional, including long-term effects. The metabolic changes seen in TI, might also have additional roles for nonimmune cells. Understanding the epigenetic interactions that drive phenomena like TI is an important step for research in the fields of biology and medicine.
In the coming years, one focus will be implementing the approaches to modulate TI for precise and immediate gains in the existing therapeutic strategy: induction of TI in fighting infection or cancer and limitation of TI in inflammatory or autoimmune diseases. The most obvious and most likely fastest implementation is the addition of nonspecific immune boosters to existing vaccine formulas.
In our opinion, the end goals of research in this field are heavily tied into personalized medicine. This will generally involve two directions – firstly, the creation of necessary tools to assess the TI signatures or susceptibility, and secondly, the development of a new generation of immunomodulatory drugs used independently or in combination with other medication to adjust immune responses.
With regard to the former, we envisage that genetic and non-genetic factors that are associated with TI will contribute to the prediction of individuals at risk of developing heightened TI states or individuals who will have a lower degree of developing TI in response to certain stimuli. For the latter, nanobiologic drugs targeting myeloid cells already show great potential since TI involves important metabolic pathways, and avoiding off-target side-effects is of key importance. More therapeutic options to explore are drugs that influence chromatin accessibility. Further advancement into epigenetic-based therapeutic modulation of TI will likely be brought by RNA-based approaches that can specifically target certain immune priming long non-coding RNAs that orchestrate epigenetic regulation at specific loci.
In the coming years, we expect that TI signatures associated with more stimuli and more disease phenotypes will be characterized allowing us to refine our search for specific modulation approaches. As the results of important clinical studies will be released, therapeutic development will move into the next phases, while trained immunity-based vaccines and adjuvants will be an important preventive tool for limiting infection, especially in situations where no specific vaccines are available.
Article highlights
Trained innate immunity refers to long-term modifications of the immune cell reactivity through epigenetic and metabolic changes. It is induced by pattern recognition through mTOR signaling and leads to enhanced subsequent innate immune responses.
Therapeutic strategies targeting TI can lead to the development of new classes of immunostimulant and immunosuppressive drugs, useful in various clinical settings. The use of TI-based drugs has already been studied in the context of cancer, rheumatoid arthritis, and transplantology.
Existing vaccines and non-specific immune boosters can lead to heterologous protection, which is explained within the TI framework. It may also contribute to tumor cell removal by increasing the activity of T cells, which is reflected in the effectiveness of BCG in different types of cancer.
Maladaptive activation of the pathways involved in TI is proposed to be another part of the pathophysiology of autoimmune and inflammatory disorders. The effectiveness of mTOR inhibitors in these pathologies can be partially explained by the inhibition of TI. More efficient strategies that target TI may prove useful as chronic anti-inflammatory medication.
The use of HDL nanoparticles allows for precise targeting of hematopoietic stem cells. Drugs that both activate and suppress TI, such as MTP10-HDL and mTORi-HDL, show great promise in animal studies.
Declaration of interest
L Joosten declares that he is the scientific founder of Trained Therapeutix Discovery (TTxD) and owns two patents related to trained immunity. The authors have no other relevant affiliations or financial involvement with any organization or entity with a financial interest in or financial conflict with the subject matter or materials discussed in the manuscript apart from those disclosed.
Reviewer disclosures
Peer reviewers on this manuscript have no relevant financial or other relationships to disclose.
Additional information
Funding
References
- Janeway CA Jr. Approaching the asymptote? Evolution and revolution in immunology. Cold Spring Harb Symp Quant Biol. 1989;5 Pt 4(1):1–13. PMID: 2700931.
- Matzinger P. The danger model: a renewed sense of self. Science. 2002 Apr 12;296(5566):301–305. PMID: 11951032.
- Hayden MS, Ghosh S. NF-κB in immunobiology. Cell Res. 2011Feb;21(2):223–244. Epub 2011 Jan 18. PMID: 21243012; PMCID: PMC3193440.
- Quintin J, Saeed S, Martens JHA, et al. Candida albicans infection affords protection against reinfection via functional reprogramming of monocytes. Cell Host Microbe. 2012 Aug 16;12(2):223–232. PMID: 22901542; PMCID: PMC3864037.
- Rizzetto L, Ifrim DC, Moretti S, et al. Fungal chitin induces trained immunity in human monocytes during cross-talk of the host with Saccharomyces cerevisiae. J Biol Chem. 2016 Apr 8;291(15):7961–7972. Epub 2016 Feb 17. PMID: 26887946; PMCID: PMC4825003.
- Netea MG, Quintin J, van der Meer JW. Trained immunity: a memory for innate host defense. Cell Host Microbe. 2011 May 19;9(5):355–361. PMID: 21575907.
- Kleinnijenhuis J, Quintin J, Preijers F, et al. Bacille Calmette-Guerin induces NOD2-dependent nonspecific protection from reinfection via epigenetic reprogramming of monocytes. Proc Natl Acad Sci U S A. 2012 Oct 23;109(43):17537–17542. Epub 2012 Sep 17. PMID: 22988082; PMCID: PMC3491454.
- Arts RJW, Carvalho A, La Rocca C, et al. Immunometabolic pathways in BCG-induced trained immunity. Cell Rep. 2016 Dec 6;17(10):2562–2571. PMID: 27926861; PMCID: PMC5177620.
- Arts RJW, Moorlag SJCFM, Novakovic B, et al. BCG vaccination protects against experimental viral infection in humans through the induction of cytokines associated with trained immunity. Cell Host Microbe. 2018 Jan 10;23(1):89–100.e5. PMID: 29324233.
- Chan GC, Chan WK, Sze DM. The effects of beta-glucan on human immune and cancer cells. J Hematol Oncol. 2009 Jun 10;2(1):25. PMID: 19515245; PMCID: PMC2704234.
- Cheng SC, Quintin J, Cramer RA, et al. mTOR- and HIF-1α-mediated aerobic glycolysis as metabolic basis for trained immunity. Science. 2014 Sep 26;345(6204):1250684. Erratum in: Science. 2014 Nov 7;346:aaa1503. van der Meer, Brian M J W [corrected to van der Veer, Brian M J W]. PMID: 25258083; PMCID: PMC4226238
- Fanucchi S, Fok ET, Dalla E, et al. Immune genes are primed for robust transcription by proximal long noncoding RNAs located in nuclear compartments. Nat Genet. 2019 Jan;51(1):138–150. Epub 2018 Dec 10. Erratum in: Nat Genet. 2019 Feb;51(2):364. PMID: 30531872.
- Moorlag SJCFM, Rodriguez-Rosales YA, Gillard J, et al. BCG vaccination induces long-term functional reprogramming of human neutrophils. Cell Rep. 2020 Nov 17;33(7):108387. PMID: 33207187; PMCID: PMC7672522.
- Keating ST, Groh L, van der Heijden CDCC, et al. The Set7 lysine methyltransferase regulates plasticity in oxidative phosphorylation necessary for trained immunity induced by β-glucan. Cell Rep. 2020 Apr 21;31(3):107548. PMID: 32320649; PMCID: PMC7184679.
- Mourits VP, van Puffelen JH, Novakovic B, et al. Lysine methyltransferase G9a is an important modulator of trained immunity. Clin Transl Immunology. 2021 Feb 18;10(2):e1253. PMID: 33708384; PMCID: PMC7890679.
- Fanucchi S, Domínguez-Andrés J, Joosten LAB, et al. The intersection of epigenetics and metabolism in trained immunity. Immunity. 2021 Jan 12;54(1):32–43. Epub 2020 Nov 20. PMID: 33220235.
- Riksen NP, Netea MG. Immunometabolic control of trained immunity. Mol Aspects Med. 2021 Feb;77:100897. Epub 2020 Sep 2. PMID: 32891423; PMCID: PMC7466946.
- Ferreira AV, Domiguéz-Andrés J, Netea MG. The role of cell metabolism in innate immune memory. J Innate Immun. 2022;14(1):42–50. Epub 2020 Dec 30. Erratum in: J Innate Immun. 2021;13(3):194. PMID: 33378755; PMCID: PMC8787524.
- Moorlag SJCFM, Matzaraki V, van Puffelen JH, et al. An integrative genomics approach identifies KDM4 as a modulator of trained immunity. Eur J Immunol. 2022 Mar;52:431–446. Epub 2021 Dec 10. PMID: 34821391.
- Cai L, Sutter BM, Li B, et al. Acetyl-CoA induces cell growth and proliferation by promoting the acetylation of histones at growth genes. Mol Cell. 2011 May 20;42(4):426–437. PMID: 21596309; PMCID: PMC3109073.
- Anderson KA, Madsen AS, Olsen CA, et al. Metabolic control by sirtuins and other enzymes that sense NAD+, NADH, or their ratio. Biochim Biophys Acta Bioenerg. 2017 Dec;1858:991–998. Epub 2017 Sep 22. PMID: 28947253; PMCID: PMC5648639.
- Bekkering S, Blok BA, Joosten LA, et al. In vitro experimental model of trained innate immunity in human primary monocytes. Clin Vaccine Immunol. 2016 Dec 5;23(12):926–933. PMID: 27733422; PMCID: PMC5139603.
- Ifrim DC, Quintin J, Joosten LA, et al. Trained immunity or tolerance: opposing functional programs induced in human monocytes after engagement of various pattern recognition receptors. Clin Vaccine Immunol. 2014 Apr;21:534–545. Epub 2014 Feb 12. PMID: 24521784; PMCID: PMC3993125.
- van der Heijden CDCC, Noz MP, Joosten LAB, et al. Epigenetics and Trained Immunity. Antioxid Redox Signal. 2018 Oct 10;29(11):1023–1040. Epub 2017 Nov 21. PMID: 28978221; PMCID: PMC6121175.
- Biswas SK, Lopez-Collazo E. Endotoxin tolerance: new mechanisms, molecules and clinical significance. Trends Immunol. 2009 Oct;30(10):475–487. Epub 2009 Sep 24. PMID: 19781994.
- Dillon S, Agrawal S, Banerjee K, et al. Yeast zymosan, a stimulus for TLR2 and dectin-1, induces regulatory antigen-presenting cells and immunological tolerance. J Clin Invest. 2006 Apr;116(4):916–928. PMID: 16543948; PMCID: PMC1401484.
- Butcher SK, O’Carroll CE, Wells CA, et al. Toll-like receptors drive specific patterns of tolerance and training on restimulation of macrophages. Front Immunol. 2018 May 14;9:933. PMID: 29867935; PMCID: PMC5960718.
- Bomans K, Schenz J, Sztwiertnia I, et al. Sepsis induces a long-lasting state of trained immunity in bone marrow monocytes. Front Immunol. 2018 Nov 19;9:2685. PMID: 30510555; PMCID: PMC6254543.
- Ratter JM, Rooijackers HMM, Hooiveld GJ, et al. In vitro and in vivo effects of lactate on metabolism and cytokine production of human primary PBMCs and monocytes. Front Immunol. 2018 Nov 12;9:2564. PMID: 30483253; PMCID: PMC6240653.
- Yang K, Xu J, Fan M, et al. Lactate suppresses macrophage pro-inflammatory response to LPS stimulation by inhibition of YAP and NF-κB activation via GPR81-mediated signaling. Front Immunol. 2020 Oct 6;11:587913. PMID: 33123172; PMCID: PMC7573489.
- Kleinnijenhuis J, Quintin J, Preijers F, et al. Long-lasting effects of BCG vaccination on both heterologous Th1/Th17 responses and innate trained immunity. J Innate Immun. 2014;6(2):152–158. Epub 2013 Oct 30. PMID: 24192057; PMCID: PMC3944069.
- Nankabirwa V, Tumwine JK, Mugaba PM, et al. Child survival and BCG vaccination: a community based prospective cohort study in Uganda. BMC Public Health. 2015 Feb 22;15(1):175. PMID: 25886062; PMCID: PMC4342809.
- Mitroulis I, Ruppova K, Wang B, et al. Modulation of myelopoiesis progenitors is an integral component of trained immunity. Cell. 2018 Jan 11;172(1–2):147–161.e12. PMID: 29328910; PMCID: PMC5766828.
- Cirovic B, de Bree LCJ, Groh L, et al. BCG vaccination in humans elicits trained immunity via the hematopoietic progenitor compartment. Cell Host Microbe. 2020 Aug 12;28(2):322–334.e5. Epub 2020 Jun 15. PMID: 32544459; PMCID: PMC7295478.
- Hamada A, Torre C, Drancourt M, et al. Trained immunity carried by non-immune cells. Front Microbiol. 2019 Jan 14;9:3225. PMID: 30692968; PMCID: PMC6340064.
- Bigot J, Guillot L, Guitard J, et al. Respiratory epithelial cells can remember infection: a proof-of-concept study. J Infect Dis. 2020 Mar 2;221(6):1000–1005. PMID: 31678998.
- Schnack L, Sohrabi Y, Lagache SMM, et al. Mechanisms of trained innate immunity in oxLDL primed human coronary smooth muscle cells. Front Immunol. 2019 Jan 23;10:13. PMID: 30728822; PMCID: PMC6351498.
- Blok BA, Arts RJ, van Crevel R, et al. Trained innate immunity as underlying mechanism for the long-term, nonspecific effects of vaccines. J Leukoc Biol. 2015 Sep;98(3):347–356. Epub 2015 Jul 6. PMID: 26150551.
- Gyssens IC, Netea MG. Heterologous effects of vaccination and trained immunity. Clin Microbiol Infect. 2019 Dec;25(12):1457–1458. Epub 2019 May 31. PMID: 31158520.
- van ‘t Wout JW, Poell R, van Furth R. The role of BCG/PPD-activated macrophages in resistance against systemic candidiasis in mice. Scand J Immunol. 1992 Nov;36(5):713–719. PMID: 1439583.
- Sher NA, Chaparas SD, Greenberg LE, et al. Effects of BCG, Corynebacterium parvum, and methanol-extraction residue in the reduction of mortality from Staphylococcus aureus and Candida albicans infections in immunosuppressed mice. Infect Immun. 1975 Dec;12(6):1325–1330. PMID: 1107224; PMCID: PMC415439.
- Berendsen ML, van Gijzel SW, Smits J, et al. BCG vaccination is associated with reduced malaria prevalence in children under the age of 5 years in sub-Saharan Africa. BMJ Glob Health. 2019 Nov 14;4(6):e001862. PMID: 31798997; PMCID: PMC6861070.
- Hippmann G, Wekkeli M, Rosenkranz AR, et al. Unspezifische immunstimulation mit BCG bei herpes simplex recidivans. nachbeobachtung 5 bis 10 Jahre nach BCG-Vakzination [Nonspecific immune stimulation with BCG in Herpes simplex recidivans. Follow-up 5 to 10 years after BCG vaccination]. Wien Klin Wochenschr. 1992;104(7):200–204. German. PMID: 1523844.
- Salem A, Nofal A, Hosny D. Treatment of common and plane warts in children with topical viable Bacillus Calmette-Guerin. Pediatr Dermatol. 2013 Jan-Feb;30(1):60–63. Epub 2012 Sep 7. PMID: 22958215.
- Wardhana, Datau EA, Sultana A, et al. The efficacy of Bacillus Calmette-Guerin vaccinations for the prevention of acute upper respiratory tract infection in the elderly. Acta Med Indones. 2011 Jul;43(3):185–190. PMID: 21979284.
- Stensballe LG, Nante E, Jensen IP, et al. Acute lower respiratory tract infections and respiratory syncytial virus in infants in Guinea-Bissau: a beneficial effect of BCG vaccination for girls community based case-control study. Vaccine. 2005 Jan 26;23(10):1251–1257. PMID: 15652667.
- Ristori G, Faustman D, Matarese G, et al. Bridging the gap between vaccination with Bacille Calmette-Guérin (BCG) and immunological tolerance: the cases of type 1 diabetes and multiple sclerosis. Curr Opin Immunol. 2018 Dec;55:89–96. Epub 2018 Nov 15. PMID: 30447407
- Martín C, Marinova D, Aguiló N, et al. MTBVAC, a live TB vaccine poised to initiate efficacy trials 100 years after BCG. Vaccine. 2021 Dec 8;39(50):7277–7285. Epub 2021 Jul 6. PMID: 34238608.
- Tarancón R, Domínguez-Andrés J, Uranga S, et al. New live attenuated tuberculosis vaccine MTBVAC induces trained immunity and confers protection against experimental lethal pneumonia. PLoS Pathog. 2020 Apr 2;16:e1008404. PMID: 32240273; PMCID: PMC7117655.
- Abarca K, Rey-Jurado E, Muñoz-Durango N, et al. Safety and immunogenicity evaluation of recombinant BCG vaccine against respiratory syncytial virus in a randomized, double-blind, placebo-controlled phase I clinical trial. EClinicalMedicine. 2020 Oct 6;27:100517. PMID: 33073219; PMCID: PMC7548429.
- Soto JA, Gálvez NMS, Pacheco GA, et al. Induction of protective immunity by a single low dose of a master cell bank cGMP-rBCG-P vaccine against the human metapneumovirus in mice. Front Cell Infect Microbiol. 2021 Jun 29;11:662714. PMID: 34268134; PMCID: PMC8276701.
- Broset E, Saubi N, Guitart N, et al. MTBVAC-Based TB-HIV vaccine is safe, elicits HIV-T cell responses, and protects against mycobacterium tuberculosis in mice. Mol Ther Methods Clin Dev. 2019 Feb 7;13:253–264. PMID: 30859110; PMCID: PMC6395831.
- O’Neill LAJ, Netea MG. BCG-induced trained immunity: can it offer protection against COVID-19? Nat Rev Immunol. 2020 Jun;20(6):335–337. PMID: 32393823; PMCID: PMC7212510.
- Hensel J, McAndrews KM, McGrail DJ, et al. Protection against SARS-CoV-2 by BCG vaccination is not supported by epidemiological analyses. Sci Rep. 2020 Oct 27;10(1):18377. PMID: 33110184; PMCID: PMC7591473.
- Debisarun PA, Gössling KL, Bulut O, et al. Induction of trained immunity by influenza vaccination - impact on COVID-19. PLoS Pathog. 2021 Oct 25;17(10):e1009928. PMID: 34695164; PMCID: PMC8568262.
- Shao Y, Saredy J, Xu K, et al. Endothelial Immunity trained by coronavirus infections, DAMP stimulations and regulated by anti-oxidant NRF2 may contribute to inflammations, myelopoiesis, COVID-19 cytokine storms and thromboembolism. Front Immunol. 2021 Jun 25;12:653110. PMID: 34248940; PMCID: PMC8269631.
- Del Fresno C, García-Arriaza J, Martínez-Cano S, et al. The bacterial mucosal immunotherapy MV130 protects against SARS-CoV-2 infection and improves COVID-19 vaccines immunogenicity. Front Immunol. 2021 Nov 18;12:748103. PMID: 34867974; PMCID: PMC8637175.
- Brandi P, Conejero L, Cueto FJ, et al. Trained immunity induction by the inactivated mucosal vaccine MV130 protects against experimental viral respiratory infections. Cell Rep. 2022 Jan 4;38(1):110184. PMID: 34986349; PMCID: PMC8755442.
- Salzmann M, Haider P, Kaun C, et al. Innate immune training with bacterial extracts enhances lung macrophage recruitment to protect from betacoronavirus infection. J Innate Immun. 2021 Nov;12:1–13. Epub ahead of print. PMID: 34775384
- Suárez N, Ferrara F, Rial A, et al. Bacterial lysates as immunotherapies for respiratory infections: methods of preparation. Front Bioeng Biotechnol. 2020 Jun 5;8:545. PMID: 32582669; PMCID: PMC7289947.
- Domínguez-Andrés J, van Crevel R, Divangahi M, et al. Designing the next generation of vaccines: relevance for future pandemics. mBio. 2020 Dec 22;11(6):e02616–20. PMID: 33443120; PMCID: PMC8534290.
- Sánchez-Ramón S, Conejero L, Netea MG, et al. Trained immunity-based vaccines: a new paradigm for the development of broad-spectrum anti-infectious formulations. Front Immunol. 2018 Dec 17;9:2936. PMID: 30619296; PMCID: PMC6304371.
- Morales A, Eidinger D, Bruce AW. Intracavitary Bacillus Calmette-Guerin in the treatment of superficial bladder tumors. J Urol. 1976 Aug;116(2):180–183. PMID: 820877.
- Lu JL, Xia QD, Lu YH, et al. Efficacy of intravesical therapies on the prevention of recurrence and progression of non-muscle-invasive bladder cancer: a systematic review and network meta-analysis. Cancer Med. 2020 Nov;9(9):7800–7809. Epub 2020 Oct 11 Erratum in: Cancer Med. 2021 Aug;10(16):5722. PMID: 33040478; PMCID: PMC7643689
- Anastasiadis A, de Reijke TM. Best practice in the treatment of nonmuscle invasive bladder cancer. Ther Adv Urol. 2012Feb;4(1):13–32. PMID: 22295042; PMCID: PMC3263923.
- Ratliff TL, Kavoussi LR, Catalona WJ. Role of fibronectin in intravesical BCG therapy for superficial bladder cancer. J Urol. 1988 Feb;139(2):410–414. PMID: 3276931.
- de Queiroz NMGP, Marinho FV, de Araujo ACVSC, et al. MyD88-dependent BCG immunotherapy reduces tumor and regulates tumor microenvironment in bladder cancer murine model. Sci Rep. 2021 Aug 2;11(1):15648. PMID: 34341449; PMCID: PMC8329301.
- Han J, Gu X, Li Y, et al. Mechanisms of BCG in the treatment of bladder cancer-current understanding and the prospect. Biomed Pharmacother. 2020Sep;129:110393. Epub 2020 Jun 16. PMID: 32559616.
- Conti P, Reale M, Nicolai M, et al. Bacillus Calmette-Guérin potentiates monocyte responses to lipopolysaccharide-induced tumor necrosis factor and interleukin-1, but not interleukin-6 in bladder cancer patients. Cancer Immunol Immunother. 1994 Jun;38(6):365–371. PMID: 8205557.
- De Boer EC, De Jong WH, Steerenberg PA, et al. Induction of urinary interleukin-1 (IL-1), IL-2, IL-6, and tumour necrosis factor during intravesical immunotherapy with Bacillus Calmette-Guérin in superficial bladder cancer. Cancer Immunol Immunother. 1992;34(5):306–312. PMID: 1540977.
- van Puffelen JH, Keating ST, Oosterwijk E, et al. Trained immunity as a molecular mechanism for BCG immunotherapy in bladder cancer. Nat Rev Urol. 2020 Sep;17(9):513–525. Epub 2020 Jul 16. PMID: 32678343.
- Buffen K, Oosting M, Quintin J, et al. Autophagy controls BCG-induced trained immunity and the response to intravesical BCG therapy for bladder cancer. PLoS Pathog. 2014 Oct 30;10(10):e1004485. PMID: 25356988; PMCID: PMC4214925.
- Usher NT, Chang S, Howard RS, et al. Association of BCG vaccination in childhood with subsequent cancer diagnoses: a 60-year follow-up of a clinical trial. JAMA Network Open. 2019 Sep 4;2(9):e1912014. PMID: 31553471; PMCID: PMC6763973.
- Morra ME, Kien ND, Elmaraezy A, et al. Early vaccination protects against childhood leukemia: a systematic review and meta-analysis. Sci Rep. 2017 Nov 22;7(1):15986. PMID: 29167460; PMCID: PMC5700199.
- Villumsen M, Sørup S, Jess T, et al. Risk of lymphoma and leukaemia after Bacille Calmette-Guérin and smallpox vaccination: a Danish case-cohort study. Vaccine. 2009 Nov 16;27(49):6950–6958. Epub 2009 Sep 9. PMID: 19747577.
- Kremenovic M, Schenk M, Lee DJ. Clinical and molecular insights into BCG immunotherapy for melanoma. J Intern Med. 2020 Dec;288(6):625–640. Epub 2020 Mar 4. PMID: 32128919.
- Nakamura T, Fukiage M, Higuchi M, et al. Nanoparticulation of BCG-CWS for application to bladder cancer therapy. J Control Release. 2014 Feb 28;176:44–53. Epub 2013 Dec 31. PMID: 24389133.
- Masuda H, Nakamura T, Noma Y, et al. Application of BCG-CWS as a systemic adjuvant by using nanoparticulation technology. Mol Pharm. 2018 Dec 3;15(12):5762–5771. Epub 2018 Nov 8. PMID: 30380885.
- Priem B, van Leent MMT, Teunissen AJP, et al. Trained immunity-promoting nanobiologic therapy suppresses tumor growth and potentiates checkpoint inhibition. Cell. 2020 Oct 29;183(3):786–801.e19. PMID: 33125893; PMCID: PMC8074872.
- Lamb DJ, Eales LJ, Ferns GA. Immunization with Bacillus Calmette-Guerin vaccine increases aortic atherosclerosis in the cholesterol-fed rabbit. Atherosclerosis. 1999 Mar;143(1):105–113. PMID: 10208485.
- Sohrabi Y, Lagache SMM, Schnack L, et al. mTOR-dependent oxidative stress regulates oxLDL-induced trained innate immunity in human monocytes. Front Immunol. 2019 Jan 22;9:3155. PMID: 30723479; PMCID: PMC6350618.
- Bekkering S, van den Munckhof I, Nielen T, et al. Innate immune cell activation and epigenetic remodeling in symptomatic and asymptomatic atherosclerosis in humans in vivo. Atherosclerosis. 2016 Nov;254:228–236. Epub 2016 Oct 12. PMID: 27764724
- Christ A, Günther P, Lauterbach MAR, et al. Western diet triggers NLRP3-dependent innate immune reprogramming. Cell. 2018 Jan 11;172(1–2):162–175.e14. PMID: 29328911; PMCID: PMC6324559.
- van Kampen E, Jaminon A, van Berkel TJ, et al. Diet-induced (epigenetic) changes in bone marrow augment atherosclerosis. J Leukoc Biol. 2014 Nov;96(5):833–841. Epub 2014 Jul 14. PMID: 25024399.
- Seijkens T, Hoeksema MA, Beckers L, et al. Hypercholesterolemia-induced priming of hematopoietic stem and progenitor cells aggravates atherosclerosis. FASEB J. 2014 May;28(5):2202–2213. Epub 2014 Jan 30. PMID: 24481967.
- Swirski FK, Libby P, Aikawa E, et al. Ly-6Chi monocytes dominate hypercholesterolemia-associated monocytosis and give rise to macrophages in atheromata. J Clin Invest. 2007 Jan;117(1):195–205. PMID: 17200719; PMCID: PMC1716211.
- Edgar L, Akbar N, Braithwaite AT, et al. Hyperglycemia induces trained immunity in macrophages and their precursors and promotes atherosclerosis. Circulation. 2021 Sep 21;144(12):961–982. Epub 2021 Jul 13. PMID: 34255973; PMCID: PMC8448412.
- Arts RJW, Joosten LAB, Netea MG. The potential role of trained immunity in autoimmune and autoinflammatory disorders. Front Immunol. 2018 Feb 20;9:298. PMID: 29515591; PMCID: PMC5826224.
- Dai X, Dai X, Gong Z, et al. Disease-Specific autoantibodies induce trained immunity in RA synovial tissues and its gene signature correlates with the response to clinical therapy. Mediators Inflamm. 2020 Oct 6;2020:2109325. PMID: 33082707; PMCID: PMC7558774.
- McGarry T, Hanlon MM, Marzaioli V, et al. Rheumatoid arthritis CD14+ monocytes display metabolic and inflammatory dysfunction, a phenotype that precedes clinical manifestation of disease. Clin Transl Immunology. 2021 Jan 19;10(1):e1237. PMID: 33510894; PMCID: PMC7815439.
- Lioté F, Boval-Boizard B, Weill D, et al. Blood monocyte activation in rheumatoid arthritis: increased monocyte adhesiveness, integrin expression, and cytokine release. Clin Exp Immunol. 1996 Oct;106(1):13–19. PMID: 8870692; PMCID: PMC2200557.
- Messemaker TC, Mikkers HMM, Huizinga TW, et al. Inflammatory genes TNFα and IL6 display no signs of increased H3K4me3 in circulating monocytes from untreated rheumatoid arthritis patients. Genes Immun. 2017 Sep;18(3):191–196. Epub 2017 Aug 10. PMID: 28794503.
- Yao H, Niu H, Yan N, et al. FRI0063 Rapamycin induces remission in patients with refractory rheumatoid arthritis. Ann Rheum Dis. 2018;77:578.
- Shao P, Ma L, Ren Y, et al. Modulation of the immune response in rheumatoid arthritis with strategically released rapamycin. Mol Med Rep. 2017 Oct;16(4):5257–5262. Epub 2017 Aug 18. PMID: 28849205; PMCID: PMC5647081.
- Herrada AA, Escobedo N, Iruretagoyena M, et al. Innate immune cells’ contribution to systemic lupus erythematosus. Front Immunol. 2019 Apr 15;10:772. PMID: 31037070; PMCID: PMC6476281.
- He J, Ma J, Ren B, et al. Advances in systemic lupus erythematosus pathogenesis via mTOR signaling pathway. Semin Arthritis Rheum. 2020 Apr;50:314–320. Epub 2019 Nov 11. PMID: 31796213.
- Zhang Z, Shi L, Dawany N, et al. H3K4 tri-methylation breadth at transcription start sites impacts the transcriptome of systemic lupus erythematosus. Clin Epigenetics. 2016 Feb 2;8(1):14. PMID: 26839600; PMCID: PMC4736279.
- Zhang Z, Song L, Maurer K, et al. Global H4 acetylation analysis by ChIP-chip in systemic lupus erythematosus monocytes. Genes Immun. 2010 Mar;11(2):124–133. Epub 2009 Aug 27. PMID: 19710693; PMCID: PMC2832080.
- Grigoriou M, Banos A, Filia A, et al. Transcriptome reprogramming and myeloid skewing in haematopoietic stem and progenitor cells in systemic lupus erythematosus. Ann Rheum Dis. 2020 Feb;79(2):242–253. Epub 2019 Nov 28. PMID: 31780527; PMCID: PMC7025734
- Shi Y, Evans JE, Rock KL. Molecular identification of a danger signal that alerts the immune system to dying cells. Nature. 2003 Oct 2;425(6957):516–521. Epub 2003 Sep 7. PMID: 14520412.
- Hu DE, Moore AM, Thomsen LL, et al. Uric acid promotes tumor immune rejection. Cancer Res. 2004 Aug 1;64(15):5059–5062. PMID: 15289304.
- Taus F, Santucci MB, Greco E, et al. Monosodium urate crystals promote innate anti-mycobacterial immunity and improve BCG efficacy as a vaccine against tuberculosis. PLoS One. 2015 May 29;10(5):e0127279. PMID: 26023779; PMCID: PMC4449037.
- Kono H, Chen CJ, Ontiveros F, et al. Uric acid promotes an acute inflammatory response to sterile cell death in mice. J Clin Invest. 2010 Jun;120:1939–1949. Epub 2010 May 24. PMID: 20501947; PMCID: PMC2877935
- Crișan TO, Cleophas MC, Oosting M, et al. Soluble uric acid primes TLR-induced proinflammatory cytokine production by human primary cells via inhibition of IL-1Ra. Ann Rheum Dis. 2016 Apr;75(4):755–762. Epub 2015 Feb 3. PMID: 25649144.
- Crişan TO, Cleophas MCP, Novakovic B, et al. Uric acid priming in human monocytes is driven by the AKT-PRAS40 autophagy pathway. Proc Natl Acad Sci U S A. 2017 May 23;114(21):5485–5490. Epub 2017 May 8. PMID: 28484006; PMCID: PMC5448210.
- Ma Q, Honarpisheh M, Li C, et al. Soluble uric acid is an intrinsic negative regulator of monocyte activation in monosodium urate crystal-induced tissue inflammation. J Immunol. 2020 Aug 1;205:789–800. Epub 2020 Jun 19. PMID: 32561569.
- Liu W, Xiao X, Demirci G, et al. Innate NK cells and macrophages recognize and reject allogeneic nonself in vivo via different mechanisms. J Immunol. 2012 Mar 15;188:2703–2711. Epub 2012 Feb 10. PMID: 22327074; PMCID: PMC3298083.
- van Leent MMT, Meerwaldt AE, Berchouchi A, et al. A modular approach toward producing nanotherapeutics targeting the innate immune system. Sci Adv. 2021 Mar 5;7(10):eabe7853. PMID: 33674313; PMCID: PMC7935355.
- Braza MS, van Leent MMT, Lameijer M, et al. Inhibiting Inflammation with myeloid cell-specific nanobiologics promotes organ transplant acceptance. Immunity. 2018 Nov 20;49(5):819–828.e6. Epub 2018 Nov 6. PMID: 30413362; PMCID: PMC6251711.
- Akpa CA, Kleo K, Lenze D, et al. DZNep-mediated apoptosis in B-cell lymphoma is independent of the lymphoma type, EZH2 mutation status and MYC, BCL2 or BCL6 translocations. PLoS One. 2019 Aug16;14(8):e0220681. PMID: 31419226; PMCID: PMC6697340.
- Girard N, Bazille C, Lhuissier E, et al. 3-Deazaneplanocin A (DZNep), an inhibitor of the histone methyltransferase EZH2, induces apoptosis and reduces cell migration in chondrosarcoma cells. PLoS One. 2014 May 22;9(5):e98176. PMID: 24852755; PMCID: PMC4031152.
- Cruz FF, Rocco PR, Pelosi P. Anti-inflammatory properties of anesthetic agents. Crit Care. 2017 Mar 21;21(1):67. PMID: 28320449; PMCID: PMC5359894.
- Arain MR, Buggy DJ. Anaesthesia for cancer patients. Curr Opin Anaesthesiol. 2007 Jun;20(3):247–253. PMID: 17479030.
- Bulut O, Kilic G, Domínguez-Andrés J, et al. Overcoming immune dysfunction in the elderly: trained immunity as a novel approach. Int Immunol. 2020 Nov 23;32(12):741–753. PMID: 32766848; PMCID: PMC7680842.
- Bakker OB, Aguirre-Gamboa R, Sanna S, et al. Integration of multi-omics data and deep phenotyping enables prediction of cytokine responses. Nat Immunol. 2018 Jul;19(7):776–786. Epub 2018 May 21. PMID: 29784908; PMCID: PMC6022810.