ABSTRACT
Introduction
Interferon beta (IFN beta) preparations are an established group of drugs used for immunomodulation in patients with multiple sclerosis (MS). Subcutaneously (sc) applied interferon beta-1a (IFN beta-1a sc) has been in continuous clinical use for 25 years as a disease-modifying treatment.
Areas covered
Based on data published since 2018, we discuss recent insights from analyses of the pivotal trial PRISMS and its long-term extension as well as from newer randomized studies with IFN beta-1a sc as the reference treatment, the use of IFN beta-1a sc across the patient life span and as a bridging therapy, recent data regarding the mechanisms of action, and potential benefits of IFN beta-1a sc regarding vaccine responses.
Expert opinion
IFN beta-1a sc paved the way to effective immunomodulatory treatment of MS, enabled meaningful insights into the disease process, and remains a valid therapeutic option in selected vulnerable MS patient groups.
1. Introduction
Since our previous review of the knowledge base on interferon beta-1a for subcutaneous injection (IFN beta-1a sc) in multiple sclerosis (MS) 20 years after its regulatory approval in Europe [Citation1], significant new data and evaluations have been published. The present article therefore provides a necessary update.
Interferons (IFNs) are signaling glycoproteins initially discovered as cytokines involved in antiviral defense mechanisms [Citation2]. The advent of recombinant DNA technology enabled the development of IFN alpha-based medications as drugs primarily directed against viral diseases [Citation3]. After the immunoregulatory potential of IFNs was recognized, IFN beta preparations followed as the first immunomodulatory treatment option for patients with MS [Citation4].
IFN beta preparations are a group of disease-modifying therapies (DMTs) with one of the longest histories of use in people with MS. They were the first therapeutic agents that convincingly reduced the relapse rate and disability progression in patients with relapsing-remitting MS (RRMS), the first to show efficacy in secondary progressive MS (SPMS) with relapses, and the first to be used successfully and safely in pediatric patients with MS [Citation5–7]. The therapeutic potential of IFN beta in this disease has been widely attributed to the selective anti-inflammatory action via downregulation of the chronic overexpression of proinflammatory cytokines [Citation8,Citation9]. Recently, however, new evidence revived the previously prevailing hypothesis of an etiological role of herpesviridae, in particular Epstein-Barr virus (EBV), in the pathogenesis of MS [Citation10–12] – refocusing the interest to the antiviral effects of IFN beta as mediators of its therapeutic potential in the disease [Citation13].
IFN beta-1a sc has been in continuous clinical use for 25 years after its regulatory approval in Europe, i.e. for the full time span of one human generation. IFN beta-1a sc (Rebif®) received its marketing authorization on 4 May 1998 in Europe and on 8 March 2002 in the U.S.A.. The recommended dosage of IFN beta-1a is 44 μg three times weekly by subcutaneous injection. A reduced dose of 22 μg dose is recommended for patients who do not tolerate the higher standard dose [Citation14]. The safety profile of IFN beta-1a sc is similar in children, adolescents, and adults [Citation7], and the licensed indication covers a wide spectrum of patient ages. Since its introduction, the estimated cumulative exposure to subcutaneously injected glycosylated IFN beta-1a amounts to more than 1.9 million patient years [Citation15].
While several drugs were licensed, introduced, and established in the meantime [Citation16,Citation17], IFN beta-1a sc remains a mainstay in the DMT of MS [Citation18] based on extensive data from clinical studies and real-world use (, ). This is illustrated by the current prescription prevalence of IFN beta-1a preparations in Germany of 70.8 per 1000 MS patients (IFN beta-1b: 34.1) – in a range comparable to the oral DMTs teriflunomide (54.5) and fingolimod (63.8), while the overall DMT prescription prevalence in MS patients was 483 per 1000 in 2019 [Citation41].
Figure 1. Key clinical studies with IFN beta-1a sc covering the full spectrum of relapsing MS course types and disease durations [Citation20,Citation21,Citation24–26,Citation28,Citation30,Citation33,Citation35].
![Figure 1. Key clinical studies with IFN beta-1a sc covering the full spectrum of relapsing MS course types and disease durations [Citation20,Citation21,Citation24–26,Citation28,Citation30,Citation33,Citation35].](/cms/asset/c71b7fcc-23cb-41c4-81cf-aa800da49dd8/ierm_a_2248391_f0001_oc.jpg)
Table 1. Therapeutic studies on IFN beta-1a sc in multiple sclerosis discussed in this review.
In our previous publication [Citation1], we comprehensively reviewed the knowledge base on IFN beta-1a and IFN beta-1b in MS 20 years after their market introduction in Europe. Meanwhile, long-term experience with IFN beta-1a sc grew larger over a full generation as the early patients grew older. Moreover, significant progress was achieved regarding our understanding of the disease mechanisms of MS, and treatment approaches have been modified accordingly.
In the light of these developments, we provide a follow-up review covering data and insights on IFN beta-1a sc published since 2018 and after more than 25 years of extensive use in clinical practice. We discuss recent insights from the pivotal trial PRISMS and its long-term extension, data from randomized studies with IFN beta-1a sc as the reference, the use of IFN beta-1a sc across the patient life span, the support of adherence to the prescribed regimen, the use of IFN beta-1a sc as a bridging treatment, novel insights concerning the mechanisms of action, and potential benefits of IFN beta-1a sc regarding vaccination.
2. Insights from the pivotal trial PRISMS
The parameter NEDA (no evidence of disease activity) was introduced in 2012 to characterize the MS disease course. It has since been increasingly used as an efficacy endpoint in clinical trials on RRMS [Citation42]. In a post hoc analysis of the pivotal study PRISMS (Prevention of Relapses and Disability by Interferon beta-1a Subcutaneously in Multiple Sclerosis) initiated in the 1990s [Citation24], efficacy was confirmed using different definitions of the endpoint NEDA [Citation43]. The proportion of patients with status NEDA-3 (defined as the absence of relapses, 3-month confirmed disability progression, and active T2 lesions) in year 1 was 30.1% in the IFN beta-1a sc high dose (44 µg) group compared to 10.9% in the placebo group [Citation43]. These low rates are consistent with characteristics of the PRISMS population that comprised patients with relatively high levels of disease activity (with an average of 3 relapses in the previous 2 years) as compared to more recent trials performed in RRMS patients (with, e.g. ~2 relapses on average in the ASCLEPIOS trials on ofatumumab published in 2022 [Citation44]). In fact, over the last 25 years there has been a shift toward earlier use of IFN beta-1a preparations, i.e. in patients with less active MS, besides changes in the inclusion criteria of the clinical trials [Citation45–48].
Of note, exploratory analyses of the original data from the first year of the PRISMS study revealed an early onset of therapeutic efficacy of IFN beta-1a sc: significant improvements of radiological and clinical endpoints were observed 2 and 3 months after treatment initiation, respectively [Citation43]. These findings are in line with data from the placebo-controlled randomized IMPROVE study in 180 patients with active RRMS, showing that IFN beta-1a sc (44 µg three times weekly) has a beneficial impact on magnetic resonance imaging outcomes as early as 4 weeks after treatment initiation (reduction in the mean cumulative number of new gadolinium-enhancing lesions by 68%) [Citation49]. A recent study showed that the treatment with sc IFN beta-1a reduces the number of new lesions evolving into black holes, while ventricular enlargement occurs in the first year of treatment, likely due to pseudo-atrophy [Citation50].
Using the criteria of the Magnetic Resonance Imaging in MS (MAGNIMS) network to categorize the disease course of RRMS patients in the PRISMS study population, Sormani et al. found a median time to clinical disease activity (CDA) of 2.6 years in patients with a MAGNIMS score of 0 after 1 year of therapy, while patients with higher scores experienced new disease activity earlier (median time to CDA 1.6 and 1.3 years with score 1 and 2, respectively) [Citation51]. The median time to confirmed progression in the Expanded Disability Status Scale (EDSS) was 3.2 years in patients with a MAGNIMS score of 2 after 1 year of therapy, while the median was not reached during the 15–16 year observation period for the patient group with lower MAGNIMS scores of 0 or 1. The risk of progression was significantly lower in patients with a score of 1 versus 2 (p < 0.0001), suggesting that the continuation of IFN beta-1a sc (44 µg) after 1 year of therapy is a valid treatment option for patients with a MAGNIMS score < 2 at this point in time.
To achieve optimal efficacy, IFN beta appears to require an exposure beyond a certain threshold that may not be reached in all patients treated with the once weekly regimen licensed for intramuscular IFN beta-1a [Citation52]. This notion is supported by a previous exploratory analysis of long-term data collected over 15 years in the PRISMS study (PRISMS-15) [Citation25]: outcomes were compared in the lowest and highest quartile of the cumulative sc IFN beta-1a dose groups. Higher cumulative dose, longer time on treatment, and higher treatment adherence appeared to be associated with better outcomes in patients with RRMS. Among the patients with longer mean time on IFN beta-1a sc therapy (14.7 years, highest quartile), 86% did not reach an EDSS score of 6.0 or higher compared to 48% of the patients with shorter mean time on IFN beta-1a sc treatment (2.9 years, lowest quartile) [Citation25]. Similarly, exposure to higher cumulative doses of IFN beta-1a sc was associated with lower proportions of patients experiencing 3-month confirmed progression in the EDSS (52.8% versus 68.5%) and/or conversion to SPMS (20.8% versus 52.1%), while each additional 5 years on treatment were correlated to a 13% lower risk of relapse (hazard ratio 0.87) [Citation25]. Moreover, patients with worse outcomes may have been more likely to discontinue or switch the treatment. These findings suggest that in patients with active MS, IFN beta-1a sc is capable of reducing the risk of disease progression over prolonged periods of time. We elaborate on the underlying mechanisms of action in section 5.2 of this review.
3. Data from recent clinical studies with interferon beta-1a sc as a comparator
Besides 25 major clinical studies, including seven phase III trials and comprising a total of > 6500 patients [Citation15], IFN beta-1a sc has been widely used as a reference in randomized clinical trials that investigated drug candidates which are today considered highly effective. Beyond pivotal data on the novel treatment options, these studies provided valuable information on IFN beta-1a sc.
In a post hoc analysis of the pivotal studies OPERA I and II [Citation39] of ocrelizumab in RRMS, the proportion of patients with 24-week composite confirmed disability accumulation (CDA) due to progression independent of relapse activity (PIRA) was 80.6% in the IFN beta-1a sc group (137 of 170 events) compared to 89.1% in the ocrelizumab group (115 of 129 events) [Citation53]. In the IFN beta arm, CDA was more often due to relapse-associated worsening. The overall event rates were lower in the ocrelizumab group versus the IFN beta-1a sc (44 µg) group, primarily driven by the pronounced effect of ocrelizumab on acute inflammatory events. Accordingly, the percentage of patients with NEDA after the 96-week treatment period was 47.7% in the ocrelizumab group and 27.1% in the IFN beta-1a sc group [Citation54]. Subgroup analyses, however, showed an increased probability of the status NEDA with age: Among those patients who were randomized to IFN beta-1a sc, the proportion with NEDA was higher in the subgroup aged 40 years or older versus younger patients (33.7% vs. 22.6%) [Citation54].
Similar to these results, 89% of patients treated with IFN beta-1a sc remained free of 6-month confirmed disability progression during the two-year observation period in the CARE-MS I trial on alemtuzumab [Citation37]. This percentage was comparable to the percentage of 92% reported for the group that received the antibody therapy. The mean EDSS score improved from baseline by 0.14 points in both groups [Citation37].
A recent Bayesian network meta-analysis revealed a rate ratio of annualized relapse rate and hazard ratios of time to 3-month and 6-month confirmed disability progression for the treatment with IFN beta-1a sc (44 μg) versus placebo of 0.64, 0.66 and 0.78, respectively [Citation55]. Orally applied DMTs tended toward lower relapse rate ratios and similar progression hazard ratios compared to IFN beta-1a sc (44 μg) [Citation55]. However, these results should be interpreted with caution due to differences in the populations included in these trials that were performed in a total time span of more than three decades. In a direct comparison of IFN beta-1a sc with an oral DMT, the phase 3 TENERE trial found a similar adjusted annual relapse rate in the study group treated with the higher dosage of teriflunomide (14 mg) versus IFN beta-1a sc in relapsing MS (0.26 vs. 0.22) [Citation40].
While two prospective randomized trials (SENTINEL [Citation56] and ONWARD [Citation57]) investigated combinations of IFN beta-1a with natalizumab and cladribine, respectively, this path of development has not been followed any further predominantly due to safety concerns and limited magnitude of the expected effects versus monotherapy.
4. Interferon beta-1a sc across the patient life span
4.1. Treatment of pediatric/juvenile MS patients
Few disease-modifying medications have been licensed for the treatment of MS in pediatric patients. In Germany, IFN beta-1a remains the most frequently used DMT in children and adolescents with MS [Citation58]. In the U.S.A., pediatric patients were more likely to receive other DMTs prior to starting IFN beta-1a sc, and they stayed on treatment with IFN beta-1a sc for a shorter time compared to other regions of the world [Citation59]. In 2016–2017, 48% of those aged >12 years and 70% of those <12 years started an injectable first-line therapy with either glatiramer acetate or IFN beta. Commonly used oral or intravenous therapies initially prescribed for pediatric MS in the US include dimethyl fumarate, natalizumab, rituximab, fingolimod, and teriflunomide [Citation60].
The drug was prospectively investigated in juvenile MS patients and has a similar safety profile in adult and pediatric populations [Citation61]. However, the safety and efficacy of IFN beta-1a sc in children below 2 years of age have not yet been established, and it should not be used in this age group [Citation62].
A retrospective study published in 2013 reported on clinically useful efficacy of IFN beta-1a sc in juvenile RRMS patients in terms of a substantial reduction of the annualized relapse rate from 1.79 before to 0.47 on treatment [Citation33]. The FUTURE study [Citation22] revealed quality of life improvements in adolescent RRMS patients (n = 50) who received treatment with IFN beta-1a sc (22 μg) using the RebiSmart™ electronic autoinjection device. The parent-reported and the adolescent self-reported PedsQL4.0 scores (with the exception of emotional functioning), the psychosocial health summary score, and school functioning measures increased significantly over a 52-week period [Citation22].
4.2. IFN beta-1a sc and reproductive health
No effects of IFN beta-1a sc on male fertility, sexual potency, or libido are known from human and animal studies. Given a 3 : 1 (female/male) sex ratio of MS patients [Citation63,Citation64] and a median age at disease onset of approximately 33 years [Citation65], the therapeutic management during pregnancy and the breastfeeding period is a common issue [Citation66,Citation67]. The EU label of IFN beta-1a sc includes the explicit statement that it can be considered during pregnancy [Citation62,Citation68], and the US label includes data that help healthcare providers weigh treatment risks. Extensive experience from registries and post-marketing cohort studies support this notion: there is no evidence for an increased risk of severe congenital abnormalities or spontaneous abortions after exposure to IFN beta during the 6 months before conception or during pregnancy [Citation69–72]. If clinically required, IFN beta-1a sc may be considered during pregnancy [Citation73], e.g. in patients at risk of a rebound of disease activity after discontinuation of fingolimod [Citation74].
According to an evaluation of data from the German Multiple Sclerosis and Pregnancy Registry, exposure to IFN beta via breast milk does not increase the risk of common adverse outcomes in the first year of life. The authors concluded that IFN beta preparations can be used during the breastfeeding period [Citation75], a notion that is reflected in the current label [Citation62].
4.3. Use of IFN beta-1a sc after a first demyelinating event
In a post hoc evaluation of data from the REFLEX trial in patients with a first demyelinating event (i.e. a clinically isolated syndrome, CIS) [Citation30], early initiation of treatment with IFN beta-1a sc 44 µg three times weekly was associated with a higher likelihood of achieving the status NEDA-3 at 2, 3, and 5 years of therapy [Citation76]. The odds of NEDA-3 were higher with IFN beta-1a sc 44 µg injected three times weekly versus once weekly at year 3 (odds ratio [OR] 2.26; p = 0.024) and year 5 (OR 3.22; p = 0.048) – consistent with the studies EVIDENCE [Citation21] and PRISMS [Citation24].
Battaglini et al. used subtraction imaging analysis to evaluate the spatial distribution of inflammatory activity in the brain over two years after CIS [Citation77]. Treatment with IFN beta-1a sc reduced the development of new/enlarging lesions compared with placebo (mean number: 6.9 vs 10.9; p < 0.01), and this effect was primarily observed in brain regions with presence of high inflammatory activity, which included the anterior thalamic radiation.
4.4. Efficacy in transition from RRMS to SPMS
Regardless of the phenotypic categorization of the individual disease courses, diffuse neuroinflammation and neurodegeneration appear to be involved in the pathological mechanisms of any type of MS, while degenerative processes gain increased relevance once the disease has entered the progressive phase [Citation78–80]. In a post hoc analysis of data from the PRISMS and SPECTRIMS studies, treatment with IFN beta-1a sc delayed disability progression in a subgroup of MS patients who were in apparent transition from RRMS to SPMS [Citation81]. Therefore, patients with active disease and/or progressive worsening of disability may benefit from continued therapy with IFN beta-1a sc. Data from SPECTRIMS had also suggested a potential sex effect with a significant delay of confirmed disability progression in female but not male patients [Citation35], potentially due to hormonal factors [Citation82].
In Europe, IFN beta-1a sc is licensed for use in patients with relapsing MS, which subsumes RRMS and SPMS with relapses [Citation6,Citation14]. Similarly, according to the US Food and Drug Administration label, IFN beta-1a sc is indicated for the treatment of patients with relapsing forms of MS to decrease the frequency of clinical exacerbations and delay the accumulation of physical disability, whereas efficacy of IFN beta-1a sc in chronic progressive MS has not been established [Citation83].
4.5. Treatment of MS patients at higher age
Treatment with IFN beta preparations is comparatively common in older MS patients. In a recent analysis of data from 10 treatment centers participating in the MS-PATHS alliance, these drugs were the most commonly used DMTs in RRMS patients at ≥60 years of age (17.6%) [Citation84], an age group that is usually not represented in clinical studies [Citation48]. Based on data from a large patient cohort (n = 5340) treated with IFN beta-1a sc, Allignol et al. reported similarly high rates of relapse-free patients at 1 year after treatment initiation (94.1–95.4%) in patients of all age groups [Citation85]. While this result may be biased by the age-related decrease of inflammatory disease activity and the increasing risk of conversion to SPMS in older patients, it suggests that the effectiveness of IFN beta-1a sc on inflammatory disease activity is preserved with increasing age and also among higher-age groups in the real-world setting, while disability progression was not addressed in this study.
An analysis of the long-term effectiveness of IFN beta and glatiramer acetate demonstrated that the benefits seen in the short-term are maintained over a 10-year period [Citation86]. However, the treatment effect appeared to decrease over time, which is consistent with a meta-analysis showing that efficacies of immunomodulatory DMTs on disability progression are generally higher in younger patients than in older patients [Citation87]. The driving factor remains unclear, but subgroup analyses suggested better treatment effects in those patients treated earlier and at lower EDSS scores [Citation86].
Recently, treatment with IFN beta for >3 years has been shown to be associated with significantly reduced all-cause mortality by 56% in a large sample of patients over 10 years of follow-up as compared to no or minimal exposure to IFN beta (<6 months) [Citation88].
4.6. Neuropsychological aspects of MS
It is long-established that fatigue, cognition, and quality of life may be affected by the MS itself and the disease-modifying medication [Citation89,Citation90]. The CONFIDENCE study performed in 165 RRMS patients treated with IFN beta-1a in routine clinical practice provided evidence for a longitudinal association between depression and low cognitive status [Citation91]. A meta-analysis including 41 studies could not find any DMT that improved cognitive test performance more effectively than IFN beta [Citation92]. Cognitive game training was shown to have a beneficial effect on cognitive performance in IFN beta-treated MS patients suffering from mild cognitive impairment [Citation93]. Moreover, significantly better quality of life scores were reported for patients using IFN beta as compared to those taking teriflunomide with regard to the Mental Composite Score (MCS), the Patient-Reported Indices in MS (PRIMUS) assessment, and the Fatigue Severity Scale (FSS) [Citation94]. However, to our knowledge, no further evidence from controlled randomized studies regarding the effects of IFN beta-1a sc on neuropsychological parameters has been published since our first review on IFN beta-1a sc from 2018 [Citation1].
5. Recent information to be considered in the counseling of MS patients
5.1. Intracellular mechanisms of IFN beta signaling
The pathophysiology of RRMS is associated with an aberrant type I IFN response [Citation95], which is thought to be at least partly mediated by the downregulation of the stimulator of interferon genes (STING)/IFN beta-axis. IFN beta primarily works by activating the Janus kinase/signal transducers and activators of transcription (JAK-STAT) signaling pathway: binding of IFN beta to the type I IFN receptor causes the phosphorylation of STAT1 and STAT2 followed by the formation of STAT1-STAT2 heterodimers. These dimers translocate to the cell nucleus, bind to the IFN-stimulated response element (ISRE), and modulate the expression of ISRE-regulated genes [Citation8,Citation96]. The cellular response to IFN-mediated signals is highly complex and encompasses changes in the expression of more than 500 genes and microRNAs [Citation97–100] ().
Figure 2. Induction of type I interferon (IFN) responses and the JAK-STAT signaling pathway.
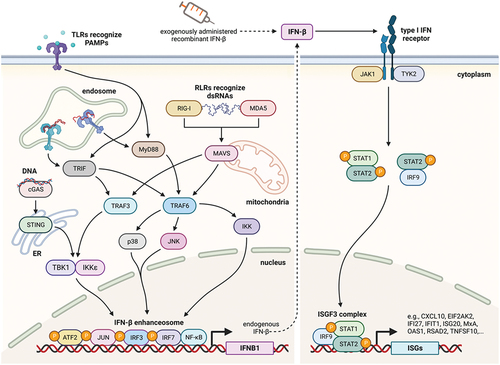
Figure 3. Mechanisms of action of IFN beta.
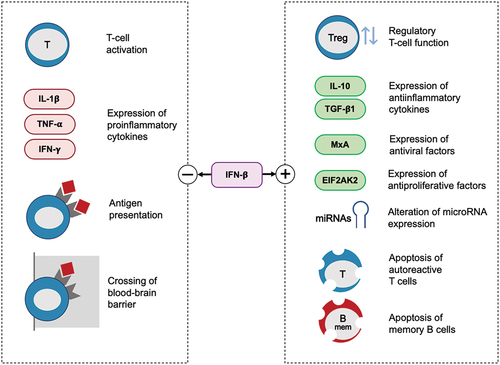
Recent evidence indicates that the type I IFN signaling can also be regulated by members of the Nod-like receptor (NLR) family [Citation104] and that the cross-talk between components of the JAK-STAT pathway and those of other pathways is complex [Citation105]. Hubel and coworkers used mass spectrometry to comprehensively explore the organization of the protein-interaction network between IFN-stimulated genes and other cellular proteins, thereby illuminating a wide range of biological activities [Citation106].
5.2. Insights regarding the mechanisms of action of interferon beta
The type I IFN signaling system has a major role in homeostasis and function of the immune system. The early interest in IFNs as a therapeutic option in MS was primarily motivated by their antiviral activities. Subsequently, as the immunomodulatory and antiproliferative properties of IFNs were discovered, the focus shifted to these regulatory features. Treatment with IFN beta thus rebalances the immune system in MS patients via highly pleiotropic effects, acting on various immune cells and molecular mediators, as previously described elsewhere [Citation107–112]. IFN beta modulates the levels of matrix metalloproteinases, adhesion molecules, and integrins, thus preventing T cell/endothelial cell adhesion and the migration of leukocytes across the blood-brain barrier [Citation111]. These mechanisms are thought to reduce inflammation and further damage to the central nervous system, even though various effects remain to been fully elucidated.
IFN beta is known to reduce the number of activated T cells. It promotes the expansion of regulatory T cells and supports their ability to suppress immune responses. Moreover, it increases the production of anti-inflammatory cytokines and decreases the production of pro-inflammatory cytokines. It also affects antigen presentation to T cells and promotes apoptosis of memory B cells (). The latter was shown to be induced in response to IFN beta therapy via a mechanism requiring Fas (FS-7-associated surface antigen/CD95)-receptor/TACI (transmembrane activator and calcium-modulator and cyclophilin ligand interactor) signaling and enhanced production of apoptosis markers (such as annexin-V and caspase-3) [Citation112]. Given the mounting evidence of a pivotal role of B cells in the immunopathology of MS, targeting these cells is increasingly perceived as a key feature of DMTs [Citation113].
Recently published research illustrated specific previously unrecognized effects of IFN beta that may be implicated in its therapeutic potential as well (). In a transcriptome profiling study performed in blood cells, long-term treatment with IFN beta was – among a multitude of other effects – associated with enhanced expression of genes involved in the oligodendroglia-protective integrated stress response and neuroprotection [Citation114]. The functional viability of oligodendroglia is vital for myelin integrity, and remyelination is explored as an approach to restore or preserve neurological functions in MS [Citation120].
Table 2. Effects of IFN beta with recently described potential therapeutic relevance in multiple sclerosis.
Effects on microglial and dendritic cell functions were discussed as further potential modes of action [Citation115,Citation116], consistent with the observation of anti-inflammatory and positive cognitive effects of IFN beta-1a in a rat model of Alzheimer’s disease and in a human pilot study [Citation121,Citation122].
The well-established antiviral effects of IFN beta are increasingly discussed as adjunct therapeutic mechanisms of action [Citation13]. Recent research appears to support the long-suspected role of EBV in the pathogenesis of MS, increasingly pointing to late infection with EBV – at an age of 10 years or later – as a crucial trigger and driver of the development of MS [Citation11,Citation123–125]. EBV increases the survival of memory B cells [Citation126,Citation127] and causes long-lasting changes in host cytokine responses [Citation124]. While the precise mechanisms of the involvement of EBV – and potentially other herpes viruses – in MS pathology remain to be fully elucidated, it may involve cross-reactions of immune responses against the viral antigen EBNA1 with autoantigens in the CNS [Citation128–130].
Disparate compositions of the intestinal microbiome have also been observed in untreated MS patients versus healthy controls [Citation131] and in patients with PPMS versus patients with RRMS [Citation132]. The involvement of the gut-microbiota-brain axis and alterations of the microbiome are increasingly discussed in the context of the pathogenesis of MS [Citation133]. Recently, cross-sectional investigations on the intestinal microbiome in MS patients suggested that the therapeutic activity of IFN beta may involve changes in the composition and diversity of microbial communities inside the gut [Citation117]. These changes may at least partially be mediated via the IFN beta-induced upregulation of short-chain fatty acid transporters located in the intestinal mucosa [Citation131,Citation134].
In vitro data further suggest that IFN beta supports the survival and immunomodulatory functions of induced regulatory T cells that stem from naïve CD4+ cells [Citation118]. According to another recent investigation, IFN beta treatment is associated with the expansion of regulatory T-cell subsets with high suppressive activity [Citation135]. As this effect was found to be most pronounced in clinically stable patients, it may constitute a therapeutic mechanism of action.
One study suggests that the initiation of treatment with IFN beta-1a sc is associated with improvements in the vascular reactivity of brain blood vessels – a critical factor for neuronal integrity [Citation119]. This effect may be mediated by reduced inflammation and modulation of vasodilatory mediators. In this way, IFN beta may help mitigate neurodegenerative processes.
5.3. Predictive identification of treatment responders
Myxovirus resistance protein A (MxA) expression in blood is a marker of IFN beta bioactivity. In a cohort of 116 patients with early RRMS, low baseline MxA mRNA levels were strongly associated with the occurrence of ≥ 9 T2 lesions (p = 0.012) and a higher number of relapses (p = 0.029) during long-term follow-up (median: 11 years) [Citation136]. Although a previous study could only show an association between low MxA mRNA levels and the relapse rate (follow-up: 5 years) in patients treated with intramuscular IFN beta-1a [Citation137], these data may support the involvement of low levels of endogenous IFN beta in the occurrence of MS disease activity. This notion is in keeping with the previous observation that patients with a higher capacity for MxA induction at 3 months of therapy with IFN beta are more likely to respond to this treatment option [Citation138].
In an analysis of data generated during the REFLEX and REFLEXION trials on IFN beta-1a sc in patients with a first demyelinating event, neopterin, soluble TRAIL (tumor necrosis factor-related apoptosis-inducing ligand), and IP-10 (interferon gamma inducible protein-10) measured in blood were confirmed as pharmacodynamic biomarkers associated with the effect of long-term treatment over 5 years [Citation139]. Similarly, in patients with SPMS, treatment response (i.e. stabilization of the EDSS score for at least 2 years) was shown to correlate with the expression levels of a set of IFN-inducible genes [Citation140]. It was also shown that IFN beta administration induces the production of the soluble IFN beta receptor isoform (sIFNAR2) in RRMS and that higher levels of sIFNAR2 might be associated with a reduced therapeutic response [Citation141]. Thus, levels of sIFNAR2 could be measured to monitor an effective response to IFN beta therapy.
Neutralizing antibodies (NAbs) directed against the therapeutic agent may appear in a subset of treated patients with any protein-based drugs, including recombinant IFN beta. NAbs directed against IFN beta may first appear as late as 2 years after treatment initiation, and they may disappear over time if NAb titers remain low [Citation142]. Pivotal studies of IFN beta preparations in MS reported negative effects of NAbs in patients with longer treatment duration [Citation143], and there is consensus that high persistent titers of NAbs indicate the loss of biological effectiveness and an absence of therapeutic efficacy, suggesting a change of treatment [Citation143,Citation144]. However, the biological factors predisposing an individual to develop NAbs remain to be further elucidated [Citation145,Citation146].
Several pharmacogenetic studies have been performed to identify single-nucleotide polymorphisms with potential influence on the individual response to IFN beta therapy [Citation147–150]. In a systematic review by Hocevar et al., 40 studies were identified that investigated the association between genetic variation and treatment response to IFN beta (5 genome-wide association studies and 35 candidate gene studies) [Citation151]. Although the studies often lack consistency – due to various study designs, differences in definitions of responders and non-responders, insufficient sample sizes and small effect sizes – there is evidence of a polygenic nature of IFN beta treatment response.
5.4. Injection devices and the adherence to the prescribed regimen
For the DMT of chronic diseases including MS, adherence to the prescribed regimen is a critical success factor regarding long-term disease outcomes [Citation152], including durable treatment response and resource utilization [Citation153–156]. In patients on treatment with frequently injected drugs, adherence is supported by the use of dedicated injection devices, which provide feedback based on the monitoring of actual drug applications [Citation34]. For IFN beta-1a sc, several injection devices have been developed and introduced since its market introduction.
In the prospective observational study REBIFLECT [Citation29], quantitative adherence to the prescribed regimen of three injections per week (using the RebiSmart® 2.0 device) was high and stable over time: a mean 97.9% of prescribed doses was injected over 2 years. Using the identical definition and the same device, the CONFIDENCE study group reported ~ 99% adherence among the patients who remained in the study (56 out of 165 patients after 36 months) [Citation91]. Further data from recent prospective observational studies (READOUTsmart [Citation157], GEPAT-SMART [Citation158], DORADA [Citation159] and others [Citation160–162]) also suggest favorable patient experiences with the dedicated injection devices (RebiSmart® and RebiSmart® 2.0) that provide an electronic documentation of injections. However, the data from these observational studies should be interpreted with caution due to substantial patient attrition rates over time, which also varied across the studies.
A recently introduced third-generation injection device (RebiSmart® 3.0) enables the wireless transfer of injection data to mobile electronic devices, thus providing seamless real-time integration of injection data into comprehensive eHealth systems [Citation163]. Overall, this approach may translate into optimized efficacy and enable longer periods of disease stability before a therapeutic escalation is required.
5.5. Use of IFN beta-1a sc as a bridging treatment
The long-term use of highly effective MS therapies may be limited by undesired effects on immunocompetence, e.g. reduced immunoglobulin levels on treatment with drugs directed against the CD20 lymphocyte surface antigen [Citation164] or the time-dependent increase of the risk of progressive multifocal leukoencephalopathy in patients treated with natalizumab [Citation165]. Concerns about drug exposure during pregnancy still prevail for some DMTs [Citation166] (see section 4.2). Therefore, temporary or definitive de-escalation approaches using drugs with less sweeping effects, including IFN beta-1a sc, have been repeatedly considered and tested [Citation167,Citation169,Citation170]. In patients who stop high-efficacy therapies associated with rebound activity after discontinuation [Citation74], bridging with injectable immunomodulatory drugs (IFN beta or glatiramer acetate) may be considered as an option [Citation171].
5.6. Potential benefits of IFN beta-1a regarding vaccine responses
While no associations between clinically overt infections and the risk of an MS relapse were observed in a recent prospective study [Citation172], protecting MS patients from infections is still important to prevent potentially serious and prolonged sequelae [Citation168]. Overall, IFN beta-1a sc is among the DMTs with the least detrimental effects on vaccine efficacy, as immunization with inactivated vaccines generally produces robust immune responses in IFN beta-treated patients [Citation173]. Interferon beta preparations therefore are considered the de facto reference standard for studies on vaccine responses in MS patients on treatment with immunomodulatory drugs. In contrast, antibody production could be impaired in MS patients treated with disease-modifying drugs that affect the numbers and/or functions of B cells, including anti-CD20 antibodies [Citation174].
In a prospective study on the vaccination against influenza virus in MS patients, no significant differences in the rates of protection against the H1N1 subtype were observed in participants treated with IFN beta-1a as compared with control subjects at 3, 6, and 12 months [Citation175]. This result is consistent with findings from other studies on the efficacy of influenza vaccines, indicating that treatment with IFN beta-1a sc does not attenuate humoral or cellular responses [Citation176–178].
The coronavirus disease-2019 (COVID-19) pandemic caused by the severe acute respiratory syndrome coronavirus 2 (SARS-CoV-2) prompted an unprecedented acceleration in the development and worldwide use of novel vaccines [Citation179,Citation180]. The humoral response to the BNT162b2 mRNA vaccine was enhanced in MS patients treated with IFN beta-1a sc according to a recent investigation in 150 subjects [Citation181]: median anti-SARS-CoV-2 spike IgG titers were significantly higher in subjects receiving IFN beta-1a sc versus healthy volunteers and versus MS patients treated with cladribine, fingolimod, natalizumab, ocrelizumab or teriflunomide.
5.7. Risk of infection and disease severity of COVID-19
In a population-based study, MS patients were less likely to develop COVID-19 while being treated with IFN beta versus other MS DMTs (p < 0.001), except for glatiramer acetate [Citation182]. Moreover, the course of the disease may be more benign in patients with ongoing use of IFN beta [Citation183]: the fatality rate of COVID-19 in patients receiving chronic treatment with IFN beta-1a sc appears to be relatively low versus external population-based comparator groups [Citation184]. A large case-control study involving more than 2300 MS patients supported the notion that ongoing therapy with IFN beta is protective against the development of severe COVID-19 [Citation185]. According to a network meta-analysis, IFN beta was the DMT associated with the lowest risk of severe COVID-19 [Citation186]. In another meta-analysis, a higher proportion of patients being treated with IFN beta in a given cohort was significantly associated with lower COVID-19-related mortality (p < 0.001) [Citation187].
IFN beta appears to be critically involved in the physiological antiviral immune response to SARS-CoV-2 [Citation188]. Cell culture experiments had already indicated in 2004 that IFN beta-1a potently inhibits the replication of SARS-CoV-1 [Citation189]. Conversely, autoantibodies directed against type I IFNs [Citation190,Citation191] and lower IFN beta expression [Citation192] were associated with substantially elevated vulnerability to COVID-19 infection. This finding may be of therapeutic interest as the viral spike proteins of some variant subtypes failed to induce endogenous IFN beta production [Citation193]. Further research will elucidate the potential of IFN beta-1a sc in infectious diseases with an immune component amenable to cytokine-based interventions.
6. Conclusions
IFN beta-1a sc has accompanied patients with MS for the time span of a full human generation, and the drug continues to provide benefit for generations: from the first MS symptoms to SPMS, from young children to pregnant women and elderly adults with MS. The first successful therapeutic studies in patients with CIS and SPMS were conducted with IFN beta preparations, and recent studies on this therapeutic agent still deliver remarkable new insights. Based on extensive data from clinical studies and more recent post hoc analyses, IFN beta-1a sc remains a mainstay in the DMT of MS [Citation16–18].
Treatment with IFN beta-1a sc delayed the disability progression in MS patients who were in apparent transition from RRMS to SPMS [Citation81]. Thus, patients with both active disease and/or progressive worsening of disability may benefit from continued therapy with IFN beta-1a sc. If clinically required, treatment with IFN beta-1a sc may be considered individually during pregnancy [Citation68,Citation73], and the breastfeeding period [Citation75], supporting its usefulness for female patients of the younger generation. Recent molecular investigations enabled the identification of additional players mediating the effects of IFN beta and of previously unrecognized biological effects that may contribute to beneficial therapy outcomes.
While more than 25 years of research and application underscore the usefulness of IFN beta-1a sc in one of the most prevalent chronic immune-mediated diseases, it is up to the current generation of researchers and physicians to further explore and fully exploit the potential of this pleiotropic immune regulator in autoimmunity and other areas of disease.
7. Expert opinion
IFN beta-1a sc has been used for two and a half decades as a first-line therapy in patients with MS. It is well established that IFN beta therapy reduces relapse rate, disability worsening, and lesion formation, thereby enhancing patients’ quality of life. IFN beta-1a is therefore still frequently used in relapsing forms of MS, and it was shown to provide treatment benefits also for patients with SPMS who are still experiencing relapses. Moreover, IFN beta-1a may be useful as a de-escalation therapy once disease activity has been stabilized with another DMT. It is thus eligible for the treatment of MS patients with a wide range of ages, disease stages, and comorbidities – while real-world data on treatment efficacy in older patients remain limited.
The continued role for IFN beta-1a in clinical practice is further supported by the long history of favorable safety outcomes with comparatively mild side effects. Common side effects such as flu-like symptoms and injection site reactions are manageable through patient education and mitigation strategies. The safety profile in the pediatric MS population is similar to that in adults. Although a variety of DMTs with different risk-benefit profiles are now available, IFN beta-1a sc therefore continues to be the preferred choice for certain subpopulations of patients, including women who are planning to become pregnant as well as those breastfeeding. Advanced electronic devices for subcutaneous self-injection increase patient satisfaction and support adherence and cost-effective use of the medication.
Further research is needed to identify predictors of response to IFN beta therapy that may guide personalized treatment decisions for MS patients. Early intervention, i.e. starting in the period following CIS, is believed to be important for long-term beneficial outcomes. However, the mechanisms of waning efficacy in a subgroup of aging patients remain poorly understood. New technological developments, such as ultra-sensitive protein analyses, single-cell transcriptome sequencing, and metabolite profiling, may help to identify molecular biomarkers for predicting the clinical response to IFN-beta therapy. Advances in pharmacogenetics also offer the potential for more personalized treatment approaches in MS but still require thorough examination.
Research on the mechanisms of IFN beta provided valuable insights into the pathophysiology of MS that also informed the development of further treatments. IFN beta therapy alleviates disease progression by modulating both humoral and cellular immune responses, but we still lack a comprehensive knowledge of the diverse biological modes of action of endogenous and therapeutic IFN beta in MS. Additional investigations are required to better understand the involved signaling pathways and their cross-talks that modulate innate and adaptive immune responses and mediate neuroprotective effects by reducing the influx of pathogenic immune cells into the CNS.
IFN beta remains an important treatment option in MS for the foreseeable future, while the therapeutic landscape is constantly evolving. Studies of newer treatments for MS will continue to rely on IFN beta-1a sc as a reference for comparison. Further research efforts will be dedicated to the search for genetic, immunologic, environmental, and lifestyle factors that may influence the individual response to treatment. Advances in precision phenotyping and personalized medicine may hold promise for the future of IFN beta treatment in MS via the identification of patients with a higher likelihood of a substantial benefit.
Article highlights
Subcutaneously (sc) applied interferon beta-1a (IFN beta-1a sc) has been in continuous clinical use for 25 years as a disease-modifying treatment in patients with multiple sclerosis (MS).
IFN beta-1a sc continues to provide benefit in a broad range of situations from the first symptoms of MS to secondary progressive MS and from young children to elderly adults with MS. It may be also used during pregnancy and the breastfeeding period if clinically required.
Adherence to the prescribed regimen of IFN beta-1a sc is supported by the use of dedicated injection devices, which provide feedback to the patient based on the monitoring of actual drug applications.
IFN beta primarily works by activating the Janus kinase/signal transducers and activators of transcription (JAK-STAT) signaling pathway, which modulates a wide range of biological activities, including antiviral defense and immune cell function.
Recently, cross-sectional investigations on the intestinal microbiome in MS patients suggested that the therapeutic activity of IFN beta may involve changes in the composition and diversity of microbial communities inside the gut.
Vaccination of MS patients being treated with IFN beta generally produces humoral and T-cell responses comparable to those observed in healthy individuals.
Ongoing use of IFN beta is associated with a lower risk of severe COVID-19 than other disease-modifying treatments.
Declaration of interest
U Zettl received research support as well as speaking fees and travel funds from Alexion, Almirall, Bayer HealthCare, Biogen, Bristol Myers Squibb, Janssen, Merck Healthcare, Novartis, Roche, Sanofi Genzyme, Teva, the European Union, the German Federal Ministry of Education and Research, the German Federal Ministry for Economic Affairs and Climate Protection, and the German Research Foundation. P Rommer received honoraria for consultancy/speaking from Alexion, Almirall, Amicus, Biogen, Merck Healthcare, Novartis, Roche, Sandoz, Sanofi-Genzyme, and TEVA. He has received research grants from Amicus, Biogen, Merck Healthcare, and Roche. L Cepek received speaking fees and travel funds from Alexion, Allergan, Almirall, Bayer HealthCare, Biogen, Bristol Myers Squibb, Celgene, Eli Lilly and Company, Lundbeck, Merck Healthcare, Merz, Novartis, Roche, Sanofi-Genzyme and Teva. O Aktas received – with approval of the Heinrich Heine University, Düsseldorf – advisor fees, travel funds, and honoraria from Alexion, Almirall, Bayer HealthCare, Biogen, Horizon, MedImmune, Merck Healthcare, Novartis, Roche, Teva, the German Federal Ministry of Education and Research, and the German Research Foundation. T Wagner and J Richter are employees of Merck Healthcare Germany GmbH, Weiterstadt, Germany. P Oschmann received research support as well as speaking fees and travel fees from Alexion, Bayer HealthCare, Biogen, Janssen, Merck Healthcare, Novartis, Pfizer, Roche, Sanofi Genzyme, and Teva. B Elias-Hamp received research support from Merck Healthcare and Novartis as well as compensation for activities (e.g. speaking fees and travel funds) from Almirall, Bayer HealthCare, Biogen, Bristol Myers Squibb, Farco-Pharma, Janssen, MedDay, Merck Healthcare, Novartis, Roche, Sandoz/Hexal, Sanofi Genzyme, and Teva. K Gehring received compensation fees for activities as speaker and advisor as well as travel funds from Alexion, Almirall, Aristo, Bayer HealthCare, Biogen, Bristol Myers Squibb, Ipsen, Janssen, Merck Healthcare, Novartis, Roche, Sanofi Genzyme, and Teva. A Chan received compensations for activities with Actelion, Alexion, Almirall, Bayer HealthCare, Biogen, Celgene, Genzyme, Merck Healthcare, Novartis, Roche, and Teva – all used for hospital-based research funding. He received research support from Biogen, Genzyme, Roche, and UCB. A. Chan serves as an associate editor for the European Journal of Neurology (Wiley). M Hecker received speaking fees and travel funds from Bayer HealthCare, Biogen, Merck Healthcare, Novartis, and Teva. The authors have no other relevant affiliations or financial involvement with any organization or entity with a financial interest in or financial conflict with the subject matter or materials discussed in the manuscript apart from those disclosed.
Reviewer disclosures
Peer reviewers on this manuscript have no relevant financial or other relationships to disclose.
Author contributions
U Zettl, T Wagner, and M Hecker conceptualized the manuscript. U Zettl, P Rommer, and M Hecker contributed to the interpretation of published material, the writing of the manuscript, and the preparation of figures and tables. O Aktas, T Wagner, J Richter, P Oschmann, L Cepek, B Elias-Hamp, K Gehring, and A Chan complemented and critically revised the contents. All authors have read and approved the final version of the manuscript for publication.
Acknowledgments
Medical writing support was provided by Markus Fischer (Fischer BioMedical, Homburg/Saar, Germany) and funded by Merck Healthcare Germany GmbH, Weiterstadt, Germany, the healthcare business of Merck KGaA, Darmstadt, Germany (CrossRef Funder ID:10.13039/100009945).
Additional information
Funding
References
- Zettl UK, Hecker M, Aktas O. Interferon β-1a and β-1b for patients with multiple sclerosis: updates to current knowledge. Expert Rev Clin Immunol. 2018;14:137–153. PubMed: 29318902.
- Isaacs A, Lindenmann J. Virus interference I, the interferon. Proc Royal Soc B. 1957;147:258–267. PubMed: 13465720.
- Dorr RT. Interferon-alpha in malignant and viral diseases. A review. Drugs. 1993;45(2):177–211. PubMed: 7681371. doi: 10.2165/00003495-199345020-00003
- Jacobs L, O’Malley J, Freeman A, et al. Intrathecal interferon reduces exacerbations of multiple sclerosis. Science. 1981;214(4524):1026–1028. PubMed: 4026626. doi: 10.1126/science.6171035
- Melendez-Torres GJ, Armoiry X, Court R, et al. Comparative effectiveness of beta-interferons and glatiramer acetate for relapsing-remitting multiple sclerosis: systematic review and network meta-analysis of trials including recommended dosages. BMC Neurol. 2018;18(1):162. PubMed: 30285675. doi: 10.1186/s12883-018-1162-9
- Bayas A, Christ M, Faissner S, et al. Disease-modifying therapies for relapsing/active secondary progressive multiple sclerosis - a review of population-specific evidence from randomized clinical trials. Ther Adv Neurol Disord. 2023;16:17562864221146836. PubMed: 36710720. doi: 10.1177/17562864221146836
- Pohl D, Rostasy K, Gärtner J, et al. Treatment of early onset multiple sclerosis with subcutaneous interferon beta-1a. Neurology. 2005;64(5):888–890. PubMed: 15753430. doi: 10.1212/01.WNL.0000153570.33845.6A
- Borden EC, Sen GC, Uze G, et al. Interferons at age 50: past, current and future impact on biomedicine. Nat Rev Drug Discov. 2007;6(12):975–990. PubMed: 18049472. doi: 10.1038/nrd2422
- Raftopoulou S, Rapti A, Karathanasis D, et al. The role of type I IFN in autoimmune and autoinflammatory diseases with CNS involvement. Front Neurol. 2022;13:1026449. PubMed: 36438941. doi: 10.3389/fneur.2022.1026449
- Ascherio A, Munger KL. Environmental risk factors for multiple sclerosis. Part I: the role of infection. Ann Neurol. 2007;61(4):288–299. PubMed: 17444504. doi: 10.1002/ana.21117
- Bjornevik K, Cortese M, Healy BC, et al. Longitudinal analysis reveals high prevalence of Epstein-Barr virus associated with multiple sclerosis. Science. 2022;375(6578):296–301. PubMed: 35025605. doi: 10.1126/science.abj8222
- Abrahamyan S, Eberspächer B, Hoshi MM, et al. Complete Epstein-Barr virus seropositivity in a large cohort of patients with early multiple sclerosis. J Neurol Neurosurg Psychiatry. 2020;91(7):681–686. PubMed: 32371533. doi: 10.1136/jnnp-2020-322941
- Sedaghat N, Etemadifar M. Revisiting the antiviral theory to explain interferon-beta’s effectiveness for relapsing multiple sclerosis. Mult Scler Relat Disord. 2022;67:104155. PubMed: 36116383. doi: 10.1016/j.msard.2022.104155
- European Medicines Agency. EPAR product information Rebif. Annex I Summary of product charateristics. https://www.ema.europa.eu/en/medicines/human/EPAR/rebif
- Merck Healthcare, data on file.
- Hauser SL, Cree BAC. Treatment of multiple sclerosis: a review. Am J Med. 2020;133(12):1380–1390. PubMed: 32682869. doi: 10.1016/j.amjmed.2020.05.049
- Wiendl H, Gold R, Berger T, et al. Multiple sclerosis therapy Consensus group (MSTCG): position statement on disease-modifying therapies for multiple sclerosis (white paper). Ther Adv Neurol Disord. 2021;14:17562864211039648. PubMed: 34422112. doi: 10.1177/17562864211039648
- Goldschmidt CH, Hua LH. Re-Evaluating the use of IFN-β and relapsing multiple sclerosis: safety, efficacy and place in therapy. Degener Neurol Neuromuscul Dis. 2020;10:29–38. PubMed: 7326221. doi: 10.2147/DNND.S224912
- Patti F, Morra VB, Amato MP, et al. Subcutaneous interferon β-1a may Protect against cognitive impairment in patients with relapsing–remitting multiple sclerosis: 5-year follow-up of the COGIMUS study. PLoS One. 2013;8(8):e74111. PubMed: 24137499. doi: 10.1371/journal.pone.0074111
- Comi G, Filippi M, Barkhof F, et al. Effect of early interferon treatment on conversion to definite multiple sclerosis: a randomised study. Lancet. 2001;357(9268):1576–1582. PubMed: 11377645. doi: 10.1016/S0140-6736(00)04725-5
- Panitch H, Goodin DS, Francis G, et al. Randomized, comparative study of interferon -1a treatment regimens in MS: the EVIDENCE trial. Neurology. 2002;59(10):1496–1506. PubMed: 12451188. doi: 10.1212/01.WNL.0000034080.43681.DA
- Ghezzi A, Bianchi A, Baroncini D, et al. A multicenter, observational, prospective study of self- and parent-reported quality of life in adolescent multiple sclerosis patients self-administering interferon-β1a using RebiSmart™—the FUTURE study. Neurol Sci. 2017;38(11):1999–2005. PubMed: 28831635. doi: 10.1007/s10072-017-3091-6
- De Stefano N, Curtin F, Stubinski B, et al. Rapid benefits of a new formulation of subcutaneous interferon beta-1a in relapsing—remitting multiple sclerosis. Mult Scler. 2010;16(7):888–892. PubMed: 20200197. doi: 10.1177/1352458510362442
- Ebers GC, PRISMS Study Group. Randomised double-blind placebo-controlled study of interferon β-1a in relapsing/remitting multiple sclerosis. The Lancet. 1998;352(9139):1498–1504. PubMed: 9820297. doi: 10.1016/S0140-6736(98)03334-0
- Kappos L, Kuhle J, Multanen J, et al. Factors influencing long-term outcomes in relapsing–remitting multiple sclerosis: PRISMS-15. J Neurol Neurosurg Psychiatry. 2015;86(11):1202–1207. PubMed: 26374702. doi: 10.1136/jnnp-2014-310024
- Kappos L, Traboulsee A, Constantinescu C, et al. Long-term subcutaneous interferon beta-1a therapy in patients with relapsing-remitting MS. Neurology. 2006;67(6):944–953. PubMed: 17000959. doi: 10.1212/01.wnl.0000237994.95410.ce
- Li DK, Paty DW. Magnetic resonance imaging results of the PRISMS trial: a randomized, double-blind, placebo-controlled study of interferon-beta1a in relapsing-remitting multiple sclerosis. Ann Neurol. 1999;46(2):197–206. PubMed: 10443885. doi: 10.1002/1531-8249(199908)46:2<197:aid-ana9>3.0.co;2-p
- PRISMS Study Group and the University of British Columbia MS/MRI Analysis Group. PRISMS-4: Long-term efficacy of interferon- -1a in relapsing MS. Neurology. 2001;56(12):1628–1636. PubMed: 11425926. doi: 10.1212/WNL.56.12.1628
- Rieckmann P, Ziemssen T, Penner IK, et al. Adherence to subcutaneous interferon beta-1a in multiple sclerosis patients receiving periodic feedback on drug use by discussion of readouts of their Rebismart® injector: results of the prospective cohort study REBIFLECT. Adv Ther. 2022;39(6):2749–2760. PubMed: 35428903. doi: 10.1007/s12325-022-02100-w
- Comi G, De Stefano N, Freedman MS, et al. Comparison of two dosing frequencies of subcutaneous interferon beta-1a in patients with a first clinical demyelinating event suggestive of multiple sclerosis (REFLEX): a phase 3 randomised controlled trial. Lancet Neurol. 2012;11(1):33–41. PubMed: 22146409. doi: 10.1016/S1474-4422(11)70262-9
- Comi G, De Stefano N, Freedman MS, et al. Subcutaneous interferon β-1a in the treatment of clinically isolated syndromes: 3-year and 5-year results of the phase III dosing frequency-blind multicentre REFLEXION study. J Neurol Neurosurg Psychiatry. 2017;88(4):285–294. PubMed: 28039317. doi: 10.1136/jnnp-2016-314843
- Mikol DD, Barkhof F, Chang P, et al. Comparison of subcutaneous interferon beta-1a with glatiramer acetate in patients with relapsing multiple sclerosis (the Rebif vs glatiramer acetate in relapsing MS disease [REGARD] study): a multicentre, randomised, parallel, open-label trial. Lancet Neurol. 2008;7(10):903–914. PubMed: 18789766. doi: 10.1016/S1474-4422(08)70200-X
- Tenembaum SN, Banwell B, Pohl D, et al. Subcutaneous interferon beta-1a in pediatric multiple sclerosis: a retrospective study. J Child Neurol. 2013;28(7):849–856. PubMed: 23666046. doi: 10.1177/0883073813488828
- Bayas A, Ouallet JC, Kallmann B, et al. Adherence to, and effectiveness of, subcutaneous interferon β-1a administered by RebiSmart® in patients with relapsing multiple sclerosis: results of the 1-year, observational SMART study. Expert Opin Drug Deliv. 2015;12(8):1239–1250. PubMed: 26098143. doi: 10.1517/17425247.2015.1057567
- Secondary Progressive Efficacy Clinical Trial of Recombinant Interferon-Beta-1a in MS (SPECTRIMS) Study Group. Randomized controlled trial of interferon-beta-1a in secondary progressive MS: clinical results. Neurology. 2001;56(11):1496–1504. PubMed: 11402106. doi: 10.1212/WNL.56.11.1496
- Trial Investigators CAMMS223, Coles AJ, Compston DA, et al. Alemtuzumab vs. interferon beta-1a in early multiple sclerosis. N Engl J Med. 2008;359:1786–1801. PubMed: 18946064.
- Cohen JA, Coles AJ, Arnold DL, et al. Alemtuzumab versus interferon beta 1a as first-line treatment for patients with relapsing-remitting multiple sclerosis: a randomised controlled phase 3 trial. Lancet. 2012;380(9856):1819–1828. PubMed: 23122652. doi: 10.1016/S0140-6736(12)61769-3
- Coles AJ, Twyman CL, Arnold DL, et al. Alemtuzumab for patients with relapsing multiple sclerosis after disease-modifying therapy: a randomised controlled phase 3 trial. Lancet. 2012;380(9856):1829–1839. PubMed: 23122650. doi: 10.1016/S0140-6736(12)61768-1
- Hauser SL, Bar-Or A, Comi G, et al. Ocrelizumab versus interferon beta-1a in relapsing multiple sclerosis. N Engl J Med. 2017;376(3):221–234. PubMed: 28002679. doi: 10.1056/NEJMoa1601277
- Vermersch P, Czlonkowska A, Grimaldi LM, et al. Teriflunomide versus subcutaneous interferon beta-1a in patients with relapsing multiple sclerosis: a randomised, controlled phase 3 trial. Mult Scler. 2014;20(6):705–716. PubMed: 24126064. doi: 10.1177/1352458513507821
- Holstiege J, Akmatov MK, Klimke K, et al. Trends in administrative prevalence of multiple sclerosis and utilization patterns of disease modifying drugs in Germany. Mult Scler Relat Disord. 2022;59:103534. PubMed: 35092945. doi: 10.1016/j.msard.2022.103534
- Rotstein D, Solomon JM, Sormani MP, et al. Association of no evidence of disease activity with no long-term disability progression in multiple sclerosis: a systematic review and meta-analysis. Neurology. 2022;9:e200032. PubMed: 36224046.
- Traboulsee A, DKB L, Cascione M, et al. Effect of interferon beta-1a subcutaneously three times weekly on clinical and radiological measures and no evidence of disease activity status in patients with relapsing–remitting multiple sclerosis at year 1. BMC Neurol. 2018;18(1):143. PubMed: 30217172. doi: 10.1186/s12883-018-1145-x
- Gärtner J, Hauser SL, Bar-Or A, et al. Efficacy and safety of ofatumumab in recently diagnosed, treatment-naive patients with multiple sclerosis: Results from ASCLEPIOS I and II. Mult Scler. 2022;28(10):1562–1575. PubMed: 35266417. doi: 10.1177/13524585221078825
- Nicholas R, Straube S, Schmidli H, et al. Trends in annualized relapse rates in relapsing–remitting multiple sclerosis and consequences for clinical trial design. Mult Scler. 2011;17(10):1211–1217. PubMed: 21586489. doi: 10.1177/1352458511406309
- Freedman MS, Comi G, De Stefano N, et al. Moving toward earlier treatment of multiple sclerosis: findings from a decade of clinical trials and implications for clinical practice. Mult Scler Relat Disord. 2014;3(2):147–155. PubMed: 25878002. doi: 10.1016/j.msard.2013.07.001
- Sorensen PS, Sellebjerg F, Hartung HP, et al. The apparently milder course of multiple sclerosis: changes in the diagnostic criteria, therapy and natural history. Brain. 2020;143(9):2637–2652. PubMed: 32710096. doi: 10.1093/brain/awaa145
- Jalusic KO, Ellenberger D, Rommer P, et al. Effect of applying inclusion and exclusion criteria of phase III clinical trials to multiple sclerosis patients in routine clinical care. Mult Scler. 2021;27(12):1852–1863. PubMed: 33467978. doi: 10.1177/1352458520985118
- De Stefano N, Sormani MP, Stubinski B, et al. Efficacy and safety of subcutaneous interferon beta-1a in relapsing–remitting multiple sclerosis: further outcomes from the IMPROVE study. Journal Of The Neurological Sciences. 2012;312(1–2):97–101. PubMed: 21880336. doi: 10.1016/j.jns.2011.08.013
- Vrenken H, Battaglini M, de Vos ML, et al. Temporal evolution of new T1-weighted hypo-intense lesions and central brain atrophy in patients with a first clinical demyelinating event treated with subcutaneous interferon β-1a. J Neurol. 2023;270(4):2271–2282. PubMed: 36723685. doi: 10.1007/s00415-022-11554-5
- Sormani MP, Freedman MS, Aldridge J, et al. MAGNIMS score predicts long-term clinical disease activity-free status and confirmed disability progression in patients treated with subcutaneous interferon beta-1a. Mult Scler Relat Disord. 2021;49:102790. PubMed: 33571946. doi: 10.1016/j.msard.2021.102790
- Baumhefner RW, Leng M. Standard dose weekly intramuscular beta interferon-1a may be inadequate for some patients with multiple sclerosis: a 19-year clinical experience using twice a week dosage. Neurol Ther. 2022;11(3):1399–1408. PubMed: 35796951. doi: 10.1007/s40120-022-00377-1
- Kappos L, Wolinsky JS, Giovannoni G, et al. Contribution of relapse-independent progression vs relapse-associated worsening to overall confirmed disability accumulation in typical relapsing multiple sclerosis in a pooled analysis of 2 randomized clinical trials. JAMA Neurol. 2020;77(9):1132–1140. PubMed: 32511687. doi: 10.1001/jamaneurol.2020.1568
- Turner B, Cree BAC, Kappos L, et al. Ocrelizumab efficacy in subgroups of patients with relapsing multiple sclerosis. J Neurol. 2019;266(5):1182–1193. PubMed: 30820738. doi: 10.1007/s00415-019-09248-6
- Samjoo IA, Drudge C, Walsh S, et al. Comparative efficacy of therapies for relapsing multiple sclerosis: a systematic review and network meta-analysis. J Comp Eff Res. 2023;12(7):e230016. PubMed: 37265062. doi: 10.57264/cer-2023-0016
- Rudick RA, Stuart WH, Calabresi PA, et al. SENTINEL investigators. Natalizumab plus interferon beta-1a for relapsing multiple sclerosis. N Engl J Med. 2006;354(9):911–923. PubMed: 16510745. doi: 10.1056/NEJMoa044396
- Montalban X, Leist TP, Cohen BA, et al. Cladribine tablets added to IFN-β in active relapsing MS: the ONWARD study. Neurol(r) Neuroimmunol Neuroinflammation. 2018;5(5):e477. PubMed: 30027104. doi: 10.1212/NXI.0000000000000477
- Frahm N, Peters M, Bätzing J, et al. Treatment patterns in pediatric patients with multiple sclerosis in Germany-a nationwide claim-based analysis. Ther Adv Neurol Disord. 2021;14:17562864211048336. PubMed: 34646362. doi: 10.1177/17562864211048336
- Krupp LB, Pohl D, Ghezzi A, et al. Subcutaneous interferon β-1a in pediatric patients with multiple sclerosis: regional differences in clinical features, disease management, and treatment outcomes in an international retrospective study. J Neurol Sci [ PubMed: 27000217]. 2016;363:33–38. doi: 10.1016/j.jns.2016.01.023
- Krysko KM, Graves J, Rensel M, et al. Use of newer disease-modifying therapies in pediatric multiple sclerosis in the US. Neurology. 2018;91(19):e1778–1787. PubMed: 30333163. doi: 10.1212/WNL.0000000000006471
- Tenembaum SN, Segura MJ. Interferon beta-1a treatment in childhood and juvenile-onset multiple sclerosis. Neurology. 2006;67(3):511–513. PubMed: 16775230. doi: 10.1212/01.wnl.0000231137.24467.aa
- European Medicines Agency. Summary of product characteristics. Rebif 22 micrograms solution for injection; (accessed Apr 8, 2023).
- Filippi M, Bar-Or A, Piehl F, et al. Multiple sclerosis. Nat Rev Dis Primers. 2018;4(1):43. PubMed: 30410033. doi: 10.1038/s41572-018-0041-4
- Wallin MT, Culpepper WJ, Campbell JD, et al. The prevalence of MS in the United States: a population-based estimate using health claims data. Neurology. 2019;92(10):e1029–e1040. PubMed: 30770430. doi: 10.1212/WNL.0000000000007035
- Romero-Pinel L, Bau L, Matas E, et al. The age at onset of relapsing-remitting multiple sclerosis has increased over the last five decades. Mult Scler Relat Disord. 2022;68:104103. PubMed: 36029708. doi: 10.1016/j.msard.2022.104103
- Haker MC, Frahm N, Hecker M, et al. Therapy of women with multiple sclerosis: an analysis of the use of drugs that may have adverse effects on the unborn child in the event of (unplanned) pregnancy. Ther Adv Drug Saf. 2023;14:20420986221143830. PubMed: 37007872. doi: 10.1177/20420986221143830
- Frahm N, Hecker M, Langhorst SE, et al. The risk of polypharmacy, comorbidities and drug-drug interactions in women of childbearing age with multiple sclerosis. Ther Adv Neurol Disord. 2020;13:1756286420969501. PubMed: 33425014. doi: 10.1177/1756286420969501
- European Medicines Agency. Summary of product characteristics. Rebif 44 micrograms solution for injection; (accessed Apr 8, 2023).
- Thiel S, Langer-Gould A, Rockhoff M, et al. Interferon-beta exposure during first trimester is safe in women with multiple sclerosis—A prospective cohort study from the German multiple sclerosis and pregnancy registry. Mult Scler. 2016;22(6):801–809. PubMed: 26920382. doi: 10.1177/1352458516634872
- Hellwig K, Geissbuehler Y, Sabidó M, et al. Pregnancy outcomes in interferon-beta-exposed patients with multiple sclerosis: results from the European interferon-beta pregnancy registry. J Neurol. 2020;267:1715–1723. PubMed: 32100126. doi: 10.1007/s00415-020-09762-y
- Korjagina M, Hakkarainen KM, Burkill S, et al. Prevalence of adverse pregnancy outcomes after exposure to interferon beta prior to or during pregnancy in women with MS: stratification by maternal and newborn characteristics in a register-based cohort study in Finland and Sweden. Mult Scler Relat Disord. 2021;48:102694. PubMed: 33429303. doi: 10.1016/j.msard.2020.102694
- Hakkarainen KM, Juuti R, Burkill S, et al. Pregnancy outcomes after exposure to interferon beta: a register-based cohort study among women with MS in Finland and Sweden. Ther Adv Neurol Disord. 2020;13:1756286420951072. PubMed: 33101459. doi: 10.1177/1756286420951072
- Krysko KM, Bove R, Dobson R, et al. Treatment of women with multiple sclerosis planning pregnancy. Curr Treat Options Neurol. 2021;23(4):11. PubMed: 33814892. doi: 10.1007/s11940-021-00666-4
- Bianco A, Lucchini M, Totaro R, et al. Disease reactivation after fingolimod discontinuation in pregnant multiple sclerosis patients. Neurotherapeutics. 2021;18(4):2598–2607. PubMed: 34494237. doi: 10.1007/s13311-021-01106-6
- Ciplea AI, Langer-Gould A, Stahl A, et al. Safety of potential breast milk exposure to IFN-β or glatiramer acetate. Neurol Neuroimmunol Neuroinflamm. 2020;7(4):e757. PubMed: 32434802. doi: 10.1212/NXI.0000000000000757
- Freedman MS, Comi G, Coyle PK, et al. No evidence of disease activity status in patients treated with early vs. delayed subcutaneous interferon β-1a. Mult Scler Relat Disord. PubMed: 31864098 2019;39:101891. doi: 10.1016/j.msard.2019.101891
- Battaglini M, Vrenken H, Tappa Brocci R, et al. Evolution from a first clinical demyelinating event to multiple sclerosis in the REFLEX trial: regional susceptibility in the conversion to multiple sclerosis at disease onset and its amenability to subcutaneous interferon beta-1a. Eur J Neurol. 2022;29(7):2024–2035. PubMed: 35274413. doi: 10.1111/ene.15314
- Dimitriou NG, Meuth SG, Martinez-Lapiscina EH, et al. Treatment of patients with multiple sclerosis transitioning between relapsing and progressive disease. CNS Drugs. 2023;37(1):69–92. PubMed: 36598730. doi: 10.1007/s40263-022-00977-3
- Reich DS, Lucchinetti CF, Calabresi PA, et al. Multiple sclerosis. N Engl J Med. 2018;378(2):169–180. PubMed: 29320652. doi: 10.1056/NEJMra1401483
- Lassmann H. Pathogenic mechanisms associated with different clinical courses of multiple sclerosis. Front Immunol. 2019;9:3116. PubMed: 30687321. doi: 10.3389/fimmu.2018.03116
- Freedman MS, Brod S, Singer BA, et al. Clinical and MRI efficacy of sc IFN β-1a tiw in patients with relapsing MS appearing to transition to secondary progressive MS: post hoc analyses of PRISMS and SPECTRIMS. J Neurol. 2020;267(1):64–75. PubMed: 31559532. doi: 10.1007/s00415-019-09532-5
- Voskuhl RR. The effect of sex on multiple sclerosis risk and disease progression. Mult Scler. 2020;26(5):554–560. PubMed: 31965884. doi: 10.1177/1352458519892491
- US Food and Drug Administration. accessed Apr 24, 2023. www.accessdata.fda.gov/drugsatfda_docs;103780s5121lbl.pdf
- Hua LH, Hersh CM, Tian F, et al. Clinical characteristics of a large multi-center cohort of people with multiple sclerosis over age 60. Mult Scler Relat Disord. 2021;47:102637. PubMed: 33276238. doi: 10.1016/j.msard.2020.102637
- Allignol A, Boutmy E, Sabidó Espin M, et al. Effectiveness, healthcare resource utilization and adherence to subcutaneous interferon beta-1a according to age in patients with multiple sclerosis: a cohort study using a US claims database. Front Neurol. 2021;12:676585. PubMed: 34381411. doi: 10.3389/fneur.2021.676585
- Palace J, Duddy M, Lawton M, et al. Assessing the long-term effectiveness of interferon-beta and glatiramer acetate in multiple sclerosis: final 10-year results from the UK multiple sclerosis risk-sharing scheme. J Neurol Neurosurg Psychiatry. 2019;90(3):251–260. PubMed: 30242090. doi: 10.1136/jnnp-2018-318360
- Weideman AM, Tapia-Maltos MA, Johnson K, et al. Meta-analysis of the age-dependent efficacy of multiple sclerosis treatments. Front Neurol. 2017;8:577. PubMed: 29176956. doi: 10.3389/fneur.2017.00577
- Ng HS, Zhu F, Kingwell E, et al. Disease-modifying drugs for multiple sclerosis and association with survival. Neurol(r) Neuroimmunol Neuroinflammation. 2022;9(5):e200005. PubMed: 35701187. doi: 10.1212/NXI.0000000000200005
- Engel C, Greim B, Zettl UK. Diagnostics of cognitive dysfunctions in multiple sclerosis. J Neurol. 2007;254 Suppl 2(S2):II30–II34. PubMed: 17503126. doi: 10.1007/s00415-007-2009-2
- Greim B, Engel C, Apel A, et al. Fatigue in neuroimmunological diseases. J Neurol. 2007;254 Suppl 2(S2):II102–II106. PubMed: 17503116. doi: 10.1007/s00415-007-2025-2
- van Ballegooijen H, van der Hiele K, Enzinger C, et al. The longitudinal relationship between fatigue, depression, anxiety, disability, and adherence with cognitive status in patients with early multiple sclerosis treated with interferon beta-1a. eNeurologicalsci. 2022;28:100409. PubMed: 35733640. doi: 10.1016/j.ensci.2022.100409
- Landmeyer NC, Bürkner PC, Wiendl H, et al. Disease-modifying treatments and cognition in relapsing-remitting multiple sclerosis: a meta-analysis. Neurology. 2020;94(22):e2373–e2383. PubMed: 32430312. doi: 10.1212/WNL.0000000000009522
- Menascu S, Aloni R, Dolev M, et al. Targeted cognitive game training enhances cognitive performance in multiple sclerosis patients treated with interferon beta 1-a. J Neuroeng Rehabil. 2021;18(1):175. PubMed: 34924009. doi: 10.1186/s12984-021-00968-3
- Lanzillo R, Sparaco M, Lavorgna L, et al. A snapshot on patient-reported outcome measures of people with multiple sclerosis on first-line therapies in a real world setting. Neurol Sci. 2020;41(11):3235–3241. PubMed: 32388646. doi: 10.1007/s10072-020-04367-9
- Masanneck L, Eichler S, Vogelsang A, et al. The STING-IFN-β-dependent axis is markedly low in patients with relapsing-remitting multiple sclerosis. Int J Mol Sci. 2020;21(23):9249. PubMed: 33291536. doi: 10.3390/ijms21239249
- Platanitis E, Demiroz D, Schneller A, et al. A molecular switch from STAT2-IRF9 to ISGF3 underlies interferon-induced gene transcription. Nat Commun. 2019;10(1):2921. PubMed: 31266943. doi: 10.1038/s41467-019-10970-y
- Weinstock-Guttman B, Badgett D, Patrick K, et al. Genomic effects of IFN-β in multiple sclerosis patients. The Journal Of Immunology. 2003;171(5):2694–2702. PubMed: 12928423. doi: 10.4049/jimmunol.171.5.2694
- Goertsches RH, Zettl UK, Hecker M. Sieving treatment biomarkers from blood gene-expression profiles: a pharmacogenomic update on two types of multiple sclerosis therapy. Pharmacogenomics. 2011;12(3):423–432. PubMed: 21449680. doi: 10.2217/pgs.10.190
- Mohammed EM. Environmental influencers, MicroRNA, and multiple sclerosis. J Cent Nerv Syst Dis. 2020;12:1179573519894955. PubMed: 32009827. doi: 10.1177/1179573519894955
- Nuzziello N, Ciaccia L, Liguori M. Precision Medicine in neurodegenerative diseases: some promising tips coming from the microRnas’ world. Cells. 2019;9(1):75. PubMed: 31892254. doi: 10.3390/cells9010075
- Ablasser A, Goldeck M, Cavlar T, et al. cGAS produces a 2′-5′-linked cyclic dinucleotide second messenger that activates STING. Nature. 2013;498(7454):380–384. PubMed: 23722158. doi: 10.1038/nature12306
- Panne D, Maniatis T, Harrison SC. An atomic model of the interferon-β enhanceosome. Cell. 2007;129(6):1111–1123. PubMed: 17574024. doi: 10.1016/j.cell.2007.05.019
- Decout A, Katz JD, Venkatraman S, et al. The cGAS–STING pathway as a therapeutic target in inflammatory diseases. Nat Rev Immunol. 2021;21(9):548–569. PubMed: 33833439. doi: 10.1038/s41577-021-00524-z
- Kienes I, Weidl T, Mirza N, et al. Role of NLRs in the regulation of type I interferon signaling, host defense and tolerance to inflammation. Int J Mol Sci. 2021;22(3):1301. PubMed: 33525590. doi: 10.3390/ijms22031301
- Hu X, Li J, Fu M, et al. The JAK/STAT signaling pathway: from bench to clinic. Signal Transduct Target Ther. 2021;6(1):402. PubMed: 34824210. doi: 10.1038/s41392-021-00791-1
- Hubel P, Urban C, Bergant V, et al. A protein-interaction network of interferon-stimulated genes extends the innate immune system landscape. Nat Immunol. 2019;20(4):493–502. PubMed: 30833792. doi: 10.1038/s41590-019-0323-3
- Jakimovski D, Kolb C, Ramanathan M, et al. Interferon β for multiple sclerosis. Cold Spring Harb Perspect Med. 2018;8(11):a032003. PubMed: 29311124. doi: 10.1101/cshperspect.a032003
- Hecker M, Hartmann C, Kandulski O, et al. Interferon-beta therapy in multiple sclerosis: the short-term and long-term effects on the patients’ individual gene expression in peripheral blood. Mol Neurobiol. 2013;48(3):737–756. PubMed: 23636981. doi: 10.1007/s12035-013-8463-1
- Kasper LH, Reder AT. Immunomodulatory activity of interferon-beta. Ann Clin Transl Neurol. 2014;1(8):622–631. PubMed: 25356432. doi: 10.1002/acn3.84
- Rommer PS, Milo R, Han MH, et al. Immunological aspects of approved MS therapeutics. Front Immunol [ PubMed: 31354720]. 2019;10:1564. doi: 10.3389/fimmu.2019.01564
- Dhib-Jalbut S, Marks S. Interferon-beta mechanisms of action in multiple sclerosis. Neurology. 2010;74(Suppl 1):S17–S24. PubMed: 20038758. doi: 10.1212/WNL.0b013e3181c97d99
- Rizzo F, Giacomini E, Mechelli R, et al. Interferon-β therapy specifically reduces pathogenic memory B cells in multiple sclerosis patients by inducing a FAS-mediated apoptosis. Immunol Cell Biol. 2016;94(9):886–894. PubMed: 27265253. doi: 10.1038/icb.2016.55
- Baker D, Marta M, Pryce G, et al. Memory B cells are major targets for effective immunotherapy in relapsing multiple sclerosis. EBioMedicine [ PubMed: 28261400]. 2017;16:41–50. doi: 10.1016/j.ebiom.2017.01.042
- Feng X, Bao R, Li L, et al. Interferon-β corrects massive gene dysregulation in multiple sclerosis: short-term and long-term effects on immune regulation and neuroprotection. EBioMedicine. 2019;49:269–283. PubMed: 31648992. doi: 10.1016/j.ebiom.2019.09.059
- Scheu S, Ali S, Mann-Nüttel R, et al. Interferon β-mediated protective functions of microglia in central nervous system autoimmunity. IJMS. 2019;20(1):190. PubMed: 30621022. doi: 10.3390/ijms20010190
- Pennell LM, Fish EN. Interferon- β regulates dendritic cell activation and migration in experimental autoimmune encephalomyelitis. Immunology. 2017;152(3):439–450. PubMed: 28646573. doi: 10.1111/imm.12781
- Castillo-Álvarez F, Pérez-Matute P, Oteo JA, et al. The influence of interferon β-1b on gut microbiota composition in patients with multiple sclerosis. Neurología. 2021;36(7):495–503. PubMed: 34537163. doi: 10.1016/j.nrl.2018.04.006
- Nishiyama N, Nakahashi-Oda C, Shibuya A. Interferon-β promotes the survival and function of induced regulatory T cells. Cytokine. 2022;158:156009. PubMed: 34537163. doi: 10.1016/j.cyto.2022.156009
- Chiarelli AM, Villani A, Mascali D, et al. Cerebrovascular reactivity in multiple sclerosis is restored with reduced inflammation during immunomodulation. Sci Rep. 2022;12(1):15453. PubMed: 32299447. doi: 10.1038/s41598-022-19113-8
- Sun Y, Yu H, Guan Y. Glia connect inflammation and neurodegeneration in multiple sclerosis. Neurosci Bull. 2023;39(3):466–478. PubMed: 36853544. doi: 10.1007/s12264-023-01034-9
- Mudò G, Frinchi M, Nuzzo D, et al. Anti-inflammatory and cognitive effects of interferon-β1a (IFNβ1a) in a rat model of Alzheimer’s disease. J Neuroinflammation. 2019;16(1):44. PubMed: 30777084. doi: 10.1186/s12974-019-1417-4
- Grimaldi LM, Zappalà G, Iemolo F, et al. A pilot study on the use of interferon beta-1a in early Alzheimer’s disease subjects. J Neuroinflammation. 2014;11(1):30. PubMed: 24524367. doi: 10.1186/1742-2094-11-30
- Schneider-Hohendorf T, Gerdes LA, Pignolet B, et al. Broader Epstein–Barr virus–specific T cell receptor repertoire in patients with multiple sclerosis. Journal Of Experimental Medicine. 2022;219(11):e20220650. PubMed: 36048016. doi: 10.1084/jem.20220650
- Soldan SS, Lieberman PM. Epstein–Barr virus and multiple sclerosis. Nat Rev Microbiol. 2023;21(1):51–64. PubMed: 35931816. doi: 10.1038/s41579-022-00770-5
- Bjornevik K, Münz C, Cohen JI, et al. Epstein–Barr virus as a leading cause of multiple sclerosis: mechanisms and implications. Nat Rev Neurol. 2023;19(3):160–171. PubMed: 36759741. doi: 10.1038/s41582-023-00775-5
- Spender LC, Inman GJ. Inhibition of germinal centre apoptotic programmes by Epstein-Barr virus. Adv Hematol. 2011;2011:829525. PubMed: 22110506. doi: 10.1155/2011/829525
- Coleman CB, McGraw JE, Feldman ER, et al. A gammaherpesvirus Bcl-2 ortholog blocks B cell receptor-mediated apoptosis and promotes the survival of developing B cells in vivo. PLOS Pathog. 2014;10(2):e1003916. PubMed: 24516386. doi: 10.1371/journal.ppat.1003916
- Tschochner M, Leary S, Cooper D, et al. Identifying patient-specific Epstein-Barr nuclear antigen-1 genetic variation and potential autoreactive targets relevant to multiple sclerosis pathogenesis. PLoS One. 2016;11(2):e0147567. PubMed: 26849221. doi: 10.1371/journal.pone.0147567
- Lanz TV, Brewer RC, Ho PP, et al. Clonally expanded B cells in multiple sclerosis bind EBV EBNA1 and GlialCAM. Nature. 2022;603(7900):321–327. PubMed: 35073561. doi: 10.1038/s41586-022-04432-7
- Hecker M, Fitzner B, Wendt M, et al. High-density peptide microarray analysis of IgG autoantibody reactivities in serum and cerebrospinal fluid of multiple sclerosis patients. Mol & Cell Proteomics. 2016;15(4):1360–1380. PubMed: 26831522. doi: 10.1074/mcp.M115.051664
- Zhou X, Baumann R, Gao X, et al. Gut microbiome of multiple sclerosis patients and paired household healthy controls reveal associations with disease risk and course. Cell. 2022;185(19):3467–3486. PubMed: 36113426. doi: 10.1016/j.cell.2022.08.021
- Reynders T, Devolder L, Valles-Colomer M, et al. Gut microbiome variation is associated to multiple sclerosis phenotypic subtypes. Ann Clin Transl Neurol. 2020;7(4):406–419. PubMed: 32162850. doi: 10.1002/acn3.51004
- Ordoñez-Rodriguez A, Roman P, Rueda-Ruzafa L, et al. Changes in gut microbiota and multiple sclerosis: a systematic review. Int J Environ Res Public Health. 2023;20(5):4624. PubMed: 36901634. doi: 10.3390/ijerph20054624
- Preiningerova JL, Jiraskova-Zakostelska Z, Srinivasan A, et al. Multiple sclerosis and microbiome. Biomolecules. 2022;12(3):433. PubMed: 35327624. doi: 10.3390/biom12030433
- Chiarini M, Capra R, Serana F, et al. Simultaneous quantification of natural and inducible regulatory T-cell subsets during interferon-β therapy of multiple sclerosis patients. J Transl Med. 2020;18(1):169. PubMed: 32299447. doi: 10.1186/s12967-020-02329-5
- Coerver EME, Strijbis EMM, Petzold LF, et al. The association between blood MxA mRNA and long-term disease activity in early multiple sclerosis. Front Neurol. 2022;13:907245. PubMed: 36062003. doi: 10.3389/fneur.2022.907245
- Hundeshagen A, Hecker M, Paap BK, et al. Elevated type I interferon-like activity in a subset of multiple sclerosis patients: molecular basis and clinical relevance. J Neuroinflammation. 2012;9(1):140. PubMed: 22727118. doi: 10.1186/1742-2094-9-140
- Matas E, Bau L, Martínez-Iniesta M, et al. Absence of MxA induction is related to a poor clinical response to interferon beta treatment in multiple sclerosis patients. J Neurol. 2016;263(4):722–729. PubMed: 26872667. doi: 10.1007/s00415-016-8053-z
- Freedman MS, Wojcik J, Holmberg KH, et al. Pharmacodynamic biomarkers of long-term interferon beta-1a therapy in REFLEX and REFLEXION. J Neuroimmunol. 2021;360:577715. PubMed: 34536787. doi: 10.1016/j.jneuroim.2021.577715
- Gurevich M, Zilkha-Falb R, Menascu S, et al. Expression of peripheral blood interferon-inducible genes predicts treatment outcome in patients with secondary progressive multiple sclerosis treated with IFN beta-1a. European Committee for Treatment and Research in Multiple Sclerosis Annual Congress; 2022; Poster 353. Amsterdam.
- Aliaga-Gaspar P, Hurtado-Guerrero I, Ciano-Petersen NL, et al. Soluble receptor isoform of IFN beta (sIFNAR2) in multiple sclerosis patients and their association with the clinical response to IFN beta treatment. Front Immunol. 2021;12:778204. PubMed: 34975865. doi: 10.3389/fimmu.2021.778204
- Deisenhammer F. Neutralizing antibodies to interferon-β and other immunological treatments for multiple sclerosis. CNS Drugs. 2009;23(5):379–396. PubMed: 19453200. doi: 10.2165/00023210-200923050-00003
- Sorensen PS. Antidrug antibodies against biological treatments for multiple sclerosis. CNS Drugs. 2022;36(6):569–589. PubMed: 35590041. doi: 10.1007/s40263-022-00920-6
- Dunn N, Fogdell-Hahn A, Hillert J, et al. Long-term consequences of high titer neutralizing antibodies to interferon-β in multiple sclerosis. Front Immunol. 2020;11:583560. PubMed: 33178215. doi: 10.3389/fimmu.2020.583560
- Waddington KE, Papadaki A, Coelewij L, et al. Using serum metabolomics to predict development of anti-drug antibodies in multiple sclerosis patients treated with IFN beta. Front Immunol [ PubMed: 32765529]. 2020;11:1527. doi: 10.3389/fimmu.2020.01527
- van der Weele L, Pollastro S, van Schaik BDC, et al. Longitudinal analysis of anti-drug antibody development in multiple sclerosis patients treated with interferon beta-1a (Rebif™) using B cell receptor repertoire analysis. J Neuroimmunol. 2022;370:577932. PubMed: 35853357. doi: 10.1016/j.jneuroim.2022.577932
- Khorrami M, Saneipour M, Moridnia A, et al. Interdependency of NINJ2 gene expression and polymorphism with susceptibility and response to interferon beta in patients with multiple sclerosis. Int J Neurosci. 2022;20:1–6. PubMed: 35912872. doi: 10.1080/00207454.2022.2102979
- Carrasco-Campos MI, Pérez-Ramírez C, Macías-Cortés E, et al. Pharmacogenetic predictors of response to interferon beta therapy in multiple sclerosis. Mol Neurobiol. 2021;58(9):4716–4726. PubMed: 34169444. doi: 10.1007/s12035-021-02454-2
- Martínez-Aguilar L, Pérez-Ramírez C, Maldonado-Montoro MDM, et al. Effect of genetic polymorphisms on therapeutic response in multiple sclerosis relapsing-remitting patients treated with interferon-beta. Mutat Res Rev Mutat Res. 2020;785:108322. PubMed: 32800273. doi: 10.1016/j.mrrev.2020.108322
- Peroni S, Sorosina M, Malhotra S, et al. A pharmacogenetic study implicates NINJ2 in the response to interferon-β in multiple sclerosis. Mult Scler. 2020;26(9):1074–1082. PubMed: 31221001. doi: 10.1177/1352458519851428
- Hočevar K, Ristić S, Peterlin B. Pharmacogenomics of multiple sclerosis: a systematic review. Front Neurol. 2019;10:134. PubMed: 30863357. doi: 10.3389/fneur.2019.00134
- Klauer T, Zettl UK. Compliance, adherence, and the treatment of multiple sclerosis. J Neurol. 2008;255(Suppl 6):87–92. PubMed: 19300966. doi: 10.1007/s00415-008-6016-8
- Rabadi MH, Just K, Xu C. Impact of adherence with disease-modifying therapies on all-cause mortality rates among veterans with multiple sclerosis. Med Sci Monit. 2022;28:e938116. PubMed: 36131525. doi: 10.12659/MSM.938116
- Evans C, Marrie RA, Zhu F, et al. Adherence to disease-modifying therapies for multiple sclerosis and subsequent hospitalizations: MS adherence and hospitalizations. Pharmacoepidemiol Drug Saf. 2017;26(6):702–711. PubMed: 28370875. doi: 10.1002/pds.4207
- Burks J, Marshall TS, Ye X. Adherence to disease-modifying therapies and its impact on relapse, health resource utilization, and costs among patients with multiple sclerosis. Clinicoecon Outcomes Res. 2017;9:251–260. PubMed: 28496344. doi: 10.2147/CEOR.S130334
- Pardo G, Pineda ED, Ng CD, et al. The association between persistence and adherence to disease-modifying therapies and healthcare resource utilization and costs in patients with multiple sclerosis. J Health Econ Outcomes Res. 2022;9(1):111–116. PubMed: 35586512. doi: 10.36469/jheor.2022.33288
- Rieckmann P, Schwab M, Pöhlau D, et al. Adherence to subcutaneous IFN β-1a in multiple sclerosis: final analysis of the non-interventional study READOUTsmart using the dosing log and readout function of RebiSmart®. Adv Ther. 2019;36(1):175–186. PubMed: 30488338. doi: 10.1007/s12325-018-0839-1
- Deftereos SN, Koutlas E, Koutsouraki E, et al. Seasonal adherence to, and effectiveness of, subcutaneous interferon β-1a administered by RebiSmart® in patients with relapsing multiple sclerosis: results of the 1-year, observational GEPAT-SMART study. BMC Neurol. 2018;18(1):186. PubMed: 30400884. doi: 10.1186/s12883-018-1179-0
- Pavelek Z, Novotný M, Klímová B, et al. DORADA adherence study: full view into RebiSmart subdomains parameters in multiple sclerosis treatment. Curr Med Res Opin. 2021;37(4):589–596. PubMed: 33538199. doi: 10.1080/03007995.2021.1880886
- Sauri-Suárez S, Quiñones-Aguilar S, Contreras-Marín A, et al. Adherence to self-administering interferon-β1a using RebiSmart® device in Mexican patients with relapsing multiple sclerosis. PLoS One. 2020;15(4):e0230959. PubMed: 32310950. doi: 10.1371/journal.pone.0230959
- Vališ M, Šarláková J, Halúsková S, et al. An observational study demonstrating the adherence and ease of use of the injector device, RebiSmart®. Expert Opinion On Drug Delivery. 2022;17(5):719–724. PubMed: 35518208. doi: 10.1080/17425247.2020.1742694
- Devonshire VA, Feinstein A, Moriarty P. Adherence to interferon β-1a therapy using an electronic self-injector in multiple sclerosis: a multicentre, single-arm, observational, phase IV study. BMC Res Notes. 2016;9(1):148. PubMed: 26951043. doi: 10.1186/s13104-016-1948-z
- Arnaud L, Keiser M, Henninger E, et al. Evolution of the RebiSmart® autoinjector device in support of adherence to subcutaneous interferon beta-1a therapy for relapsing multiple sclerosis. European Committee for Treatment and Research in Multiple Sclerosis Annual Congress. Amsterdam; 2022. EP1079.
- Capasso N, Palladino R, Cerbone V, et al. Ocrelizumab effect on humoral and cellular immunity in multiple sclerosis and its clinical correlates: a 3-year observational study. J Neurol. 2023;270(1):272–282. PubMed: 36048265. doi: 10.1007/s00415-022-11350-1
- Sharma K, Tolaymat S, Yu H, et al. Progressive multifocal leukoencephalopathy in anti-CD20 and other monoclonal antibody (mAb) therapies used in multiple sclerosis: a review. J Neurol Sci. 2022;443:120459. PubMed: 36283150. doi: 10.1016/j.jns.2022.120459
- Vukusic S, Michel L, Leguy S, et al. Pregnancy with multiple sclerosis. Rev Neurol (Paris). 2021;177(3):180–194. PubMed: 32736812. doi: 10.1016/j.neurol.2020.05.005
- Vollmer BL, Wolf AB, Sillau S, et al. Evolution of disease modifying therapy benefits and risks: an argument for de-escalation as a treatment paradigm for patients with multiple sclerosis. Front Neurol. 2022;12:799138. PubMed: 35145470. doi: 10.3389/fneur.2021.799138
- Winkelmann A, Loebermann M, Reisinger EC, et al. Disease-modifying therapies and infectious risks in multiple sclerosis. Nat Rev Neurol. 2016;12(4):217–233. PubMed: 26943779. doi: 10.1038/nrneurol.2016.21
- Manzano A, Eskyté I, Ford HL, et al. Patient perspective on decisions to switch disease-modifying treatments in relapsing-remitting multiple sclerosis. Mult Scler Relat Disord. 2020;46:102507. PubMed: 32979733. doi: 10.1016/j.msard.2020.102507
- Almouzain L, Stevenson F, Chard D, et al. Switching treatments in clinically stable relapsing remitting multiple sclerosis patients planning for pregnancy. Mult Scler J Exp Transl Clin. 2021;7(1):20552173211001571. PubMed: 33796332. doi: 10.1177/20552173211001571
- Marfia GA, Centonze D, Salvetti M, et al. Bridging therapies with injectable immunomodulatory drugs in the management of multiple sclerosis: a Delphi survey of an Italian expert panel of neurologists. Front Neurol. 2022;13:898741. PubMed: 35911920. doi: 10.3389/fneur.2022.898741
- Miele G, Cepparulo S, Abbadessa G, et al. Clinically manifest infections do not increase the relapse risk in people with multiple sclerosis treated with disease-modifying therapies: a prospective study. JCM. 2023;12(3):1023. PubMed: 36769670.
- Winkelmann A, Loebermann M, Barnett M, et al. Vaccination and immunotherapies in neuroimmunological diseases. Nat Rev Neurol. 2022;18(5):289–306. PubMed: 35388213. doi: 10.1038/s41582-022-00646-5
- de Sèze J, Maillart E, Gueguen A, et al. Anti-CD20 therapies in multiple sclerosis: From pathology to the clinic. Front Immunol [ PubMed: 37033984]. 2023;14:1004795. doi: 10.3389/fimmu.2023.1004795
- Olberg HK, Eide GE, Cox RJ, et al. Antibody response to seasonal influenza vaccination in patients with multiple sclerosis receiving immunomodulatory therapy. Eur J Neurol. 2018;25(3):527–534. PubMed: 29205701. doi: 10.1111/ene.13537
- Schwid SR, Decker MD, Lopez-Bresnahan M, et al. Immune response to influenza vaccine is maintained in patients with multiple sclerosis receiving interferon beta-1a. Neurology. 2005;65(12):1964–1966. PubMed: 16380621. doi: 10.1212/01.wnl.0000188901.12700.e0
- Metze C, Winkelmann A, Loebermann M, et al. Immunogenicity and predictors of response to a single dose trivalent seasonal influenza vaccine in multiple sclerosis patients receiving disease-modifying therapies. CNS Neurosci Ther. 2019;25(2):245–254. PubMed: 30044050. doi: 10.1111/cns.13034
- Mehling M, Fritz S, Hafner P, et al. Preserved antigen-specific immune response in patients with multiple sclerosis responding to IFNβ-therapy. PLoS One. 2013;8(11):e78532. PubMed: 24223820. doi: 10.1371/journal.pone.0078532
- Monschein T, Hartung HP, Zrzavy T, et al. Vaccination and multiple sclerosis in the era of the COVID-19 pandemic. J Neurol Neurosurg Psychiatry. 2021;92(10):1033–1043. PubMed: 34353858. doi: 10.1136/jnnp-2021-326839
- Graña C, Ghosn L, Evrenoglou T, et al. Efficacy and safety of COVID-19 vaccines. Cochrane Database Of Systematic Reviews. 2022;(12): CD015477. PubMed: 36473651. doi: 10.1002/14651858.CD015477
- Maniscalco GT, Manzo V, Ferrara AL, et al. Interferon beta-1a treatment promotes SARS-CoV-2 mRNA vaccine response in multiple sclerosis subjects. Mult Scler Relat Disord. 2022;58:103455. PubMed: 349294550. doi: 10.1016/j.msard.2021.103455
- Reder AT, Centonze D, Naylor ML, et al. COVID-19 in patients with multiple sclerosis: associations with disease-modifying therapies. CNS Drugs. 2021;35(3):317–330. PubMed: 33743151. doi: 10.1007/s40263-021-00804-1
- Sosa JP, Ferreira Caceres MM, Ross Comptis J, et al. Effects of interferon beta in COVID-19 adult patients: systematic review. Infect Chemother. 2021;53(2):247–260. PubMed: 34216119. doi: 10.3947/ic.2021.0028
- Freedman MS, Jack D, Murgašová Z, et al. Outcomes of COVID-19 among patients treated with subcutaneous interferon beta-1a for multiple sclerosis. Mult Scler Relat Disord. 2021;56:103283. PubMed: 34619487. doi: 10.1016/j.msard.2021.103283
- Iaffaldano P, Lucisano G, Manni A, et al. Risk of getting COVID-19 in people with multiple sclerosis: a case-control study. Neurol(r) Neuroimmunol Neuroinflammation. 2022;9(2):e1141. PubMed: 35046084. doi: 10.1212/NXI.0000000000001141
- Barzegar M, Houshi S, Sadeghi E, et al. Association of disease-modifying therapies with COVID-19 susceptibility and severity in patients with multiple sclerosis: a systematic review and network meta-analysis. Mult Scler Int. 2022;9388813. Pub Med: 36187599. doi: 10.1155/2022/9388813
- Prosperini L, Tortorella C, Haggiag S, et al. Determinants of COVID-19-related lethality in multiple sclerosis: a meta-regression of observational studies. J Neurol. 2022;269(5):2275–2285. PubMed: 34984514. doi: 10.1007/s00415-021-10951-6
- Hartenian E, Nandakumar D, Lari A, et al. The molecular virology of coronaviruses. J Biol Chem. 2020;295(37):12910–12934. PubMed: 32661197. doi: 10.1074/jbc.REV120.013930
- Hensley LE, Fritz LE, Jahrling PB, et al. Interferon-β 1a and SARS coronavirus replication. Emerg Infect Dis. 2004;10(2):317–319. PubMed: 15030704. doi: 10.3201/eid1002.030482
- Manry J, Bastard P, Gervais A, et al. The risk of COVID-19 death is much greater and age dependent with type I IFN autoantibodies. Proc Natl Acad Sci, USA. 2022;119(21):e2200413119. PubMed: 35576468. doi: 10.1073/pnas.2200413119
- Bastard P, Gervais A, Le Voyer T, et al. Autoantibodies neutralizing type I IFNs are present in ~4% of uninfected individuals over 70 years old and account for ~20% of COVID-19 deaths. Sci Immunol. 2021;6(62):eabl4340. PubMed: 34413139. doi: 10.1126/sciimmunol.abl4340
- Maiti AK. The African-American population with a low allele frequency of SNP rs1990760 (T allele) in IFIH1 predicts less IFN-beta expression and potential vulnerability to COVID-19 infection. Immunogenetics. 2020;72(6–7):387–391. PubMed: 32737579. doi: 10.1007/s00251-020-01174-6
- Li X, Li W, Liu Z, et al. A comparative study of spike protein of SARS-CoV-2 and its variant Omicron (B.1.1.529) on some immune characteristics. Sci Rep. 2022;12(1):17058. PubMed: 36224298. doi: 10.1038/s41598-022-21690-7