1. Introduction
Contrary to the previous belief that immunological memory was exclusive to adaptive immunity, the concept of ‘trained immunity’ emerged, demonstrating that innate immune cells can also exhibit a memory-like response. Initially coined in 2011, trained immunity refers to the enhanced response of innate immune cells to subsequent encounters with antigens, facilitated by epigenetic modifications [Citation1].
Trained immunity can be adjusted to either amplify immune responses to combat infections and cancer, or to inhibit reactions contributing to autoimmune disorders and transplant rejection [Citation1]. This manipulation of trained immunity is regulated through various mechanisms, including interactions between ligands and receptors, as well as through metabolic and epigenetic changes. These mechanisms provide multiple therapeutic targets. Strategies aimed at either inhibiting trained immunity (such as in cases of autoimmune diseases or organ transplant) or promoting it (in scenarios like infections or oncology) hold significant promise for managing immune-related conditions [Citation1].
Asthma, which affects over 330 million people worldwide, is a chronic respiratory condition characterized by airway inflammation and narrowing [Citation2,Citation3]. The discovery of trained immunity has revolutionized our understanding as it suggests that early microbial exposure could modulate immune responses and influence asthma development and progression [Citation4]. However, in asthma, trained immunity may have a paradoxical effect, further complicating our understanding of this condition.
This editorial aims to discuss the current evidence regarding the link between asthma, trained immunity, and infection. Gaining insights into the complexity of this relationship could help in designing novel preventive strategies to mitigate the impact of early infections on asthma development, as well as in developing targeted therapies to modulate trained immunity profiles and alleviate chronic inflammation in asthmatics.
2. Current evidence; asthma, trained immunity, and infection
The relationship between asthma, immunity, and infection involves various immunological pathways, genetic and environmental factors, and interactions with the microbiome (). The current literature reveals a complex and multifaceted landscape.
Figure 1. The interplay between trained immunity, infection, and asthma. (this figure shows the intersection of three critical concepts: ‘trained immunity,’ ‘infection,’ and ‘asthma.’ ‘trained immunity’ is depicted as the immune system’s enhanced response to pathogens following prior exposure, potentially decreasing the susceptibility or severity of future infections. ‘Infection’ represents the entry and multiplication of pathogens in the host body, which can lead to disease. ‘Asthma’ signifies a chronic inflammatory disease of the airways. The overlap between these circles indicates that elements of trained immunity may influence the occurrence and severity of asthma and that infections can both trigger asthma exacerbations and influence the development of trained immunity).
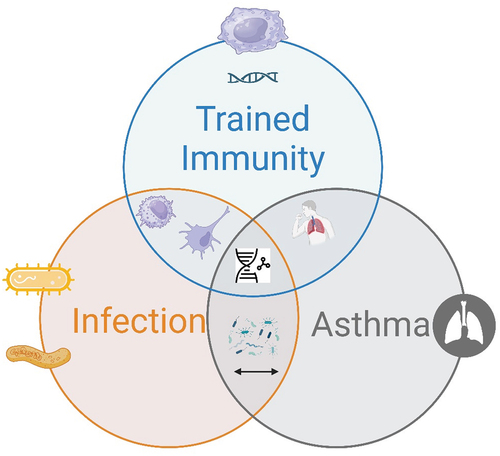
2.1. The role of trained immunity in asthma
While trained immunity possesses beneficial effects against pathogens, it may worsen inflammatory diseases by promoting disease progression through mechanisms such as inflammasome activation and epigenetic reprogramming [Citation5]. Specifically, atherosclerosis can be driven by such immune training via sterile inflammatory triggers, like oxidized LDL particles, through the NLRP3 inflammasome [Citation6]. Similarly, asthma, a complex inflammatory disease, involves acute and chronic inflammation triggered by various allergens and non-allergenic factors, including obesity and cigarette smoke [Citation7].
A previous study has identified specific inflammatory responses in monocyte-derived macrophages (MDM) from patients with house dust mite (HDM)-induced asthma and bone marrow-derived macrophages (BMDM) from HDM-exposed mice. These macrophages exhibit not only changes in gene expression but also produce higher levels of CCL17 and cysteinyl leukotrienes (cysLTs), crucial mediators in type 2 inflammatory reactions [Citation8]. This pattern of macrophage activation in asthma challenges differs notably from the traditional concept of trained immunity observed in infectious diseases or post-vaccination scenarios. The study suggests the necessity for further exploration into how these variations in immune response might intersect or communicate across various forms of trained immunity.
2.2. Infections, asthma, and trained immunity
Rhinovirus (RV), a prevalent cause of the common cold, plays a significant role in asthma exacerbations and development, particularly in children. RV infects airway epithelial cells, triggering immune responses that can exacerbate asthma. The virus evades immune defenses by downregulating interferon type I and its receptors and inducing immunosuppressive cytokines. Interferons are key in fighting viral infections, but RV manipulates the immune system to promote its replication and suppress effective antiviral responses [Citation9].
Early-life enterovirus infections have been shown to increase the risk of allergic diseases in children and exacerbate mite allergen-induced airway inflammation in adult mice. This effect has been attributed to sustained innate immune memory (trained immunity) in macrophages, which drives the differentiation of naive T helper cells toward Th2 and Th17 cells [Citation10]. Additionally, respiratory infections in infancy, combined with allergen sensitization, can lead to allergic asthma in childhood through trained immunity in lung macrophages, with metabolic reprogramming playing a crucial role [Citation11]. Kim reviewed the role of airway epithelial immunity in asthma exacerbation due to viral infections, noting differences in antiviral cytokine production between asthmatic patients and healthy subjects [Citation12]. Furthermore, prenatal maternal immune dysfunction may alter neonatal immune training through epigenetic mechanisms and lead to early-life airway colonization by asthmagenic microbiota, increasing the risk of childhood asthma development [Citation13].
2.3. Other factors influencing asthma
The relationship between farming environments and asthma incidence has garnered significant interest within the medical and scientific communities, offering insights into the complex interplay of environmental exposures and asthma development. The farming effect on asthma, often discussed in the context of the ‘hygiene hypothesis,’ suggests that exposure to a variety of microorganisms in farm environments during early life can have a protective effect against asthma and allergies. This hypothesis is supported by numerous studies that have observed lower rates of asthma among children raised on farms. Michelle M. Stein and colleagues found that the prevalence of asthma and allergic sensitization was 4 and 6 times as low in the Amish, whereas median endotoxin levels in Amish house dust was 6.8 times as high [Citation14]. Their findings suggest that the Amish environment provides protection against asthma by engaging and shaping the innate immune response.
The use of bacterial Immunotherapy, particularly bacterial lysates, for the prevention of wheezing illnesses is an area of significant research interest. Bacterial lysates are preparations made from inactivated bacteria, which are often pathogenic respiratory bacteria. These preparations have been used in various forms since the early 1950s, primarily in Europe and Asia, to prevent recurrent respiratory tract infections (RTIs) and, by extension, potentially reduce the incidence of wheezing episodes and asthma exacerbations. Bacterial lysates work by stimulating the immune system, thereby enhancing the body’s natural defenses against respiratory pathogens. In a randomized controlled trial, Antonio Nieto et al. [Citation15], reported that mucosal bacterial immunotherapy based on whole inactivated bacteria shows safety and clinical efficacy against recurrent wheezing attacks in children.
3. Expert opinion
Within the context of asthma, the concept of trained immunity offers both challenges and opportunities. The findings of this editorial highlight a dual-faceted role of trained immunity in asthma and infection interplay. On one hand, trained immunity is a promising avenue for reducing the severity and susceptibility to infections. Mihai G. Netea et al., argued that the utilizing trained immunity induced by specific vaccines, such as the Bacillus Calmette-Guérin (BCG), provides heterologous protection against a range of infections [Citation16]. This protection is mediated through epigenetic, transcriptional, and functional reprogramming of innate immune cells. Moreover, they suggested that the induction of trained immunity by whole-microorganism vaccines could serve as a pivotal strategy for diminishing both the susceptibility to and the severity of SARS-CoV-2 infections. On the other hand, its potential to exacerbate inflammatory conditions like asthma necessitates a nuanced approach to harnessing its benefits while mitigating adverse effects. The challenge lies in deciphering the mechanisms through which trained immunity influences asthma pathogenesis and infection dynamics, to tailor interventions that bolster protective immunity without aggravating asthma symptoms. Therefore, I believe that future research should focus on immune pathways that differentiate between the beneficial aspects of trained immunity and those that contribute to inflammatory exacerbations in asthma.
However, the findings of this editorial demonstrate several limitations. A major limitation is the variability in human studies, where genetic diversity, environmental factors, and lifestyle choices can significantly influence outcomes. Moreover, much of the current understanding is derived from animal models or in vitro studies, which, while informative, cannot fully replicate the complexity of human immune responses. Another critical weakness is the lack of longitudinal studies to definitively establish causal relationships between early microbial exposures, trained immunity development, and asthma onset and progression.
4. Conclusion
The interaction between asthma, trained immunity, and infection is complex, but with potential opportunities. This critical junction in immunological research highlights both the potential to harness trained immunity for protective purposes against infections, such as SARS-CoV-2, and the imperative to mitigate its adverse effects on asthma through targeted interventions.
Declaration of interests
The author have no relevant affiliations or financial involvement with any organization or entity with a financial interest in or financial conflict with the subject matter or materials discussed in the manuscript. This includes employment, consultancies, honoraria, stock ownership or options, expert testimony, grants or patents received or pending, or royalties.
Reviewer disclaimer
Peer reviewers on this manuscript have no relevant financial or other relationship to disclose.
Additional information
Funding
References
- Ochando J, Mulder WJM, Madsen JC, et al. Trained immunity — basic concepts and contributions to immunopathology. Nat Rev Nephrol. 2023;19(1):23–37. doi: 10.1038/s41581-022-00633-5
- Al Meslamani AZ. How does sleep influence asthma through immunity? Expert Rev Clin Immunol. 1–4. doi: 10.1080/1744666X.2023.2284213
- Al Meslamani AZ. What hinders individualized therapy plans for asthma patients? Expert Opin Pharmacother. 2023 Dec 12;24(18):1933–1936. doi: 10.1080/14656566.2023.2291078
- Wegmann M. Trained immunity in allergic asthma. J Allergy Clin Immunol. 2023 Jun 1;151(6):1471–1473. doi: 10.1016/j.jaci.2023.02.023
- Hartung F, Esser-von Bieren J. Trained immunity in type 2 immune responses. Mucosal Immunol. 2022;15(6):1158–1169. doi: 10.1038/s41385-022-00557-0
- Bekkering S, Quintin J, Joosten LAB, et al. Oxidized low-density lipoprotein induces long-term proinflammatory cytokine production and foam cell formation via epigenetic reprogramming of monocytes. Arterioscler Thromb Vasc Biol. 2014 Aug;34(8):1731–1738. doi: 10.1161/ATVBAHA.114.303887
- Kim HY, DeKruyff RH, Umetsu DT. The many paths to asthma: phenotype shaped by innate and adaptive immunity. Nat Immunol. 2010 Jul;11(7):577–584. doi: 10.1038/ni.1892
- Lechner A, Henkel FDR, Hartung F, et al. Macrophages acquire a TNF-dependent inflammatory memory in allergic asthma. J Allergy Clin Immunol. 2022 Jun;149(6):2078–2090. doi: 10.1016/j.jaci.2021.11.026
- Yang Z, Mitländer H, Vuorinen T, et al. Mechanism of rhinovirus immunity and asthma. Front Immunol. 2021;12:731846. doi: 10.3389/fimmu.2021.731846
- Malinczak CA, Lukacs NW, Fonseca W. Early-life respiratory syncytial virus infection, trained immunity and subsequent pulmonary diseases. Viruses. 2020;12(5):505. doi: 10.3390/v12050505
- Li H, Ma L, Li W, et al. Proline metabolism reprogramming of trained macrophages induced by early respiratory infection combined with allergen sensitization contributes to development of allergic asthma in childhood of mice. Front Immunol. 2022;13:977235. doi: 10.3389/fimmu.2022.977235
- Kim SR. Viral infection and airway epithelial immunity in asthma. Int J Mol Sci. 2022;23(17):9914. doi: 10.3390/ijms23179914
- DeVries A, McCauley K, Fadrosh D, et al. Maternal prenatal immunity, neonatal trained immunity, and early airway microbiota shape childhood asthma development. Allergy. 2022;77(12):3617–3628. doi: 10.1111/all.15442
- Stein MM, Hrusch CL, Gozdz J, et al. Innate immunity and asthma risk in Amish and Hutterite farm children. N Engl J Med. 2016 Aug;375(5):411–421. doi: 10.1056/NEJMoa1508749
- Nieto A, Mazón A, Nieto M, et al. Bacterial mucosal immunotherapy with MV130 prevents recurrent wheezing in children: a randomized, double-blind, placebo-controlled clinical trial. Am J Respir Crit Care Med. 2021 Aug;204(4):462–472. doi: 10.1164/rccm.202003-0520OC
- Netea MG, Giamarellos-Bourboulis EJ, Domínguez-Andrés J, et al. Trained immunity: a tool for reducing susceptibility to and the severity of SARS-CoV-2 infection. Cell.2020 May 28;181(5):969–977. doi: 10.1016/j.cell.2020.04.042