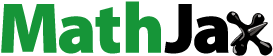
ABSTRACT
The use of enrichment and bedding materials in pig husbandry intends to comply with the animals’ behavioural needs to perform natural exploratory behaviour, which is strongly connected to foraging behaviour. It can thus be assumed that pigs will ingest a certain material quantity possibly posing a risk to animal health and food safety as previous studies identified contaminants in enrichment and bedding materials. However, risk assessment requires knowledge about the effective amount of ingested material. Voluntary material intake of pigs with free access to peat and disinfectant powder was estimated by measuring the tissue levels of toxic metals originating from the respective materials in 28 pigs (seven groups, n = 4) via inductively coupled plasma mass spectrometry and comparing the results to tissue levels of pigs fed with known amounts of metals. Additionally, as markers of consumption, n-alkanes and acid insoluble ash naturally occurring in the materials and titanium dioxide, added as an external marker to disinfectant powder, were analysed in pigs’ faeces. Tissue levels of toxic metals as well as marker analyses in pigs’ faeces could prove material consumption. Results revealed mean voluntary intake levels of peat and disinfectant powder by pigs up to 7% and 2% of the daily ration. Hence, a transfer of contained toxic metals into the food chain might occur. Although current maximum levels for toxic elements in animal tissues were not exceeded due to dietary inclusion of peat or disinfectant powder, dietary exposure through food of animal origin should be reduced to a possible minimum. This applies specifically for elements, where no health-based guidance values for humans could have been derived (e.g. arsenic). Thus, labelling guidelines for enrichment and bedding materials can be a perspective to limit the entry of toxic metals and trace elements into the environment.
1. Introduction
Pigs have an intrinsic need to perform exploratory behaviour which is highly related to foraging behaviour (Mayer et al. Citation2006). Like wild boar, domesticated pigs spend 70 to 80% of their activity time foraging (Wechsler et al. Citation1991; Mayer et al. Citation2006; Kauselmann et al. Citation2021). Consequently, it is evident that in pig husbandry, besides meeting nutritional and social requirements of pigs, the ability to express natural exploratory behaviour is majorly important in pigs’ satisfaction of needs. Thus, in the European Union (EU), the provision of appropriate materials for exploration, which ideally can be chewed and eaten as well as investigated and manipulated by pigs, are included in legal regulations for pig husbandry (EU Citation2009b, Citation2016). However, exploratory behaviour is not only directed towards enrichment materials presented for this purpose, but also towards bedding and disinfectant materials and further pen equipment in the pigs’ environment and can be even redirected towards pen-mates when the quality of provided enrichment materials is insufficient (Taylor et al. Citation2010). In a comprehensive review on pig behaviour, Kittawornrat and Zimmerman (Citation2011) elucidate that the initial exploration of an object or substrate by chewing is not necessarily followed by feeding behaviour. However, nutritional feedback might lead to consumption (Day et al. Citation1996). Early studies on soil ingestion by pigs kept on grass pasture identified a soil intake up to 8% of DM of the diet (Fries et al. Citation1982). In commercial deep-litter systems, pigs consume straw, rice hulls and sawdust as bedding material up to 14, 10 and 12% of their daily ration, respectively (van Barneveld Citation2012). Intake of recycled materials used for animal bedding was shown by Fernandes et al. (Citation2019), who identified contaminants originating from recycled materials in animal tissues. A recent study investigating pigs’ preference for peat, biochar, disinfectant powder and straw confirmed the consumption of all four materials (Koch et al. Citation2022). Although disinfectant powder turned out to be the least preferred material, its consumption by pigs supports the assumption that even exploratory chewing behaviour, which does not intend feeding, might lead to considerable intake (Kittawornrat and Zimmerman Citation2011).
The fact that pigs consume at least a small portion of materials in their environment becomes majorly important as researchers found levels of contaminants in bedding, enrichment and disinfectant materials which might pose a risk to animal and consumer health when ingested by pigs (Fernandes et al. Citation2019; Koch et al. Citation2021). To perform a proper risk assessment, knowledge about actual material intake by pigs is required. Previously, n-alkanes have been used to quantify intake of bedding materials by pigs (van Barneveld Citation2012). However, this approach is labour-intensive and not applicable for all types of material (e.g. disinfectant powder). Still, n-alkanes naturally occurring in peat have been recently used to qualitatively prove consumption of peat by pigs. Likewise, acid insoluble ash (AIA) has been identified as a qualitative marker for the intake of disinfectant powder (Koch et al. Citation2022).
The aim of this study was to identify material-specific marker substances that accumulate in the pig’s body without being rapidly excreted. By measuring the concentrations of the respective markers in the target tissues, it might be possible to draw conclusions on the amount of ingested material. Such a marker might be cadmium, which has been identified in various bedding materials beside other toxic metals (Koch et al. Citation2021). The accumulation of cadmium in porcine kidney and liver is an irreversible process in which tissue concentrations of cadmium can only be decreased by organ growth once exposure has stopped (Hoogenboom et al. Citation2014). In our study, we investigated the suitability of cadmium and other toxic metals in provided peat, disinfectant powder and animal tissues as a useful marker to quantify material intake by pigs. Based on the accumulation of cadmium, arsenic and lead in tissues of pigs fed a known material quantity, material intake of pigs with free access to the materials was estimated. As this study presents an approach which varies from commonly applied marker techniques quantifying the respective markers in animal faeces, it was unclear whether the study will reveal results suitable for the verification and quantification of voluntary intake of peat and disinfectant powder by pigs. Hence, internal material-specific markers (n-alkanes and AIA), known to be indicative for consumption of peat and disinfectant powder (Koch et al. Citation2022), as well as titanium dioxide (TiO2) as external marker in disinfectant powder (Jagger et al. Citation1992; Kavanagh et al. Citation2001) were additionally used with primary intention to prove actual material consumption. Secondly, the internal and external markers were used to quantify material intake in comparison to the “toxic metal approach”.
2. Materials and methods
2.1. Experimental design
A total of 28 crossbred (Danish Landrace x Yorkshire), male, castrated pigs were used in the study to investigate the voluntary intake of peat and disinfectant powder, respectively. The pigs (8 weeks of age, 24.0 ± 1.83 kg BW) were randomly allocated into seven groups with four pigs each. They were left to get used to the new environment for seven days. Each pen (10 and 13 m2 for two and four pigs, respectively) was equipped with a rubber lying mat providing enough space for all pigs lying simultaneously and a straw rack providing fresh straw daily (Supplementary Figure S1). Further enrichment materials (e.g. balls and wood) were provided and changed regularly. Additionally, in pens of the treatment groups a tub (203 × 80 × 18.5 cm) for material provision was set up. Pigs received their diet, adopted to their metabolic BW (110 g/kg0.75) (Kamphues et al. Citation2014) and meeting nutritional requirements (), in two meals per day and water ad libitum. After the seven-day adaptation period, the 12-week test period started. Accordingly, groups received different treatments ().
Figure 1. Experimental groups (4 pigs each) to quantify consumption of peat and disinfectant powder by pigs; no material treatment: negative control group (NC); material treatment: positive control groups – diet containing 10 and 20% peat (PC10 and PC20) and 3 and 5% disinfectant powder (PC3 and PC5), respectively, treatment groups – free access to peat (TPeat) and disinfectant powder (TPow), respectively.
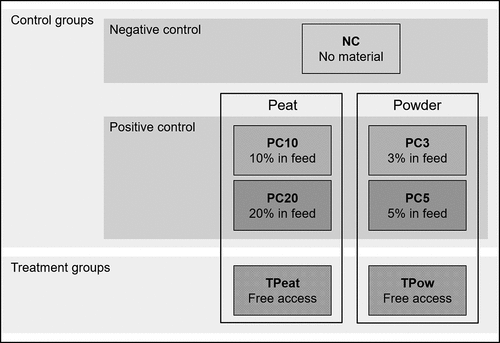
Table 1. Composition of the experimental diet (complete feed [CF]) for fattening pigs, content of proximate nutrients and metabolisable energy and content of proximate nutrients in peat and disinfectant powder.
The negative control group (NC) received neither material within the diet nor presented for voluntary intake; housing and feed did not differ during adaptation and test period. The four positive control groups received a diet with 10 and 20% peat (PC10 and PC20) and 3 and 5% disinfectant powder (PC3 and PC5), respectively; housing did not differ during adaptation and test period. The two treatment groups received the same diet as during the adaptation period, but peat (TPeat) and disinfectant powder (TPow) were presented in a tub for voluntary intake. For TPeat, 5, 10, 15 and 20 L of fresh peat (material density: ~570 g/L; particle size: <0.5 - >3 mm) were filled in the tub in the morning during week 1 to 3, 4 to 6, 7 to 9 and 10 to 12 of the test period, respectively. The peat is available on the market as rooting material for pigs to support their natural exploration behaviour and advertised to beneficially affect the animals’ digestive tract by providing natural ingredients (e.g. humic acids, bulk and trace elements). The amount of provided peat increased gradually, as the amount of contained toxic metals and trace elements would exceed maximum limits for complementary feedstuffs and peat () (EU Citation2002, Citation2017, Citation2019). Although these limits do not apply for bedding and enrichment materials, possible intoxication of pigs due to potentially complete consumption of peat in the tub was thus avoided.
Table 2. Content of toxic metals and trace elements as determined by ICP-MS in the experimental diet (complete feed [CF]) for fattening pigs as well as in peat and disinfectant powder provided in treatment groups (TPeat and TPow).
For TPow, 320 g of fresh disinfectant powder (material density: ~780 g/L; particle size: <0.5–3 mm) was filled in the tub in the morning (≙ 200 g/m2). The powder is available on the market to improve stable hygiene and air quality due to its water and ammonia-binding capacity. Furthermore, it is advertised to reduce pathogens without negatively affecting animal health. Likewise to peat, disinfectant powder contained elevated levels of toxic metals and trace elements (). However, the amount of presented material was too low to cause intoxication when ingested by pigs. Additionally, as indicator of material consumption and additional marker for quantification of material intake by pigs in the treatment group TPow, disinfectant powder presented in tubs was supplemented with TiO2 (Merck, Darmstadt, Germany) as an inert indigestible marker at 5 g/kg (Jagger et al. Citation1992; Pieper et al. Citation2016). Faecal samples were collected each week as group bulk samples. Therefore, the total amount of faeces per pen was collected prior to daily cleaning, manually mixed in a bucket and subsequently faecal samples taken and stored at −20°C until further analysis. After a total of 13 weeks (1 week adaptation, 12 weeks test period), pigs were slaughtered at a final BW of 102 ± 8.97 kg. Kidney (left body site), liver (right lateral lobe), muscle tissue (neck), bone tissue (metacarpus IV, left front leg) and faeces from the rectum were sampled and stored at −20°C until further analysis.
2.2. Sample analyses
2.2.1. Diet and materials
The experimental diet, peat and disinfectant powder were analysed for DM, crude protein, crude ash and crude fibre (Weender Analysis). Additionally, the experimental diet was analysed for content of starch (polarimetric method) in accordance with Commission Regulation (EC) No 152/2009 () (EU Citation2009a). Levels of the bulk elements calcium, phosphorus and sodium and trace elements iron, zinc, manganese, and copper in the experimental diet as well as in peat and disinfectant powder were determined by inductively coupled plasma mass spectrometry (ICP-MS) according to DIN EN ISO 17,294–2:2017–01 (). For analysis of cadmium, lead and arsenic, samples were prepared following DIN EN 13,805:2014–12 and the element content was determined by ICP-MS in accordance with DIN EN ISO 17,294–2:2017–01 and DIN EN 15,763:2010–04 ().
2.2.2. Analysis of n-alkanes
Feed, provided materials and faeces were analysed for n-alkanes following the same protocol as previously described (Koch et al. Citation2022). Briefly, (freeze-)dried samples were ground (≤0.5 mm) and lipid extracts from the samples purified and analysed for n-alkanes by gas chromatography coupled to a flame ionisation detector (GC-FID) using 1 µL injection volume on a Shimadzu GC-2010 (Shimadzu Corporation, Kryōto, Japan) according to Elwert et al. (Citation2004). The n-alkanes n-heptacosane (C27), n-nonacosane (C29), n-hentriacontane (C31) and n-tritriacontane (C33) have been identified in peat, straw, feed and faecal samples.
2.2.3. Analysis of acid insoluble ash
Feed, provided materials and faeces were analysed for AIA following the VDLUFA standard method 8.2 (VDLUFA Citation2012). Therefore, 5 g of dried sample material were incinerated and crude ash determined. The ash was boiled with 3N hydrochlorid acid and subsequently filtered. Filter and residue were dried and incinerated. Finally, the weight of the residue was calculated and expressed as a percentage of the sample weight.
2.2.4. Analysis of titanium dioxide
Disinfectant powder and faeces were analysed for TiO2 as described by Myers et al. (Citation2004). In brief, following acid digestion of 0.5 g freeze-dried sample material in concentrated sulphuric acid, 30% hydrogen peroxide was added, the sample was filtered and the absorbance measured at 410 nm using a UV spectrophotometer (Ultrospec 2100 pro photometer, Amersham Pharmacia Biotech Inc., Piscataway, NJ, USA).
2.2.5. Analysis of toxic metals in animal tissues
About 10 g sample material of partly defrosted kidney (cortex), liver and muscle tissue, respectively, were chopped on a Teflon surface using a ceramic knife. Bone samples were frozen in liquid nitrogen for about 10 s and subsequently crushed on a Teflon surface using a hammer. To prevent metal contamination from the hammer and loss of sample material during crushing, bone samples were wrapped in a microfibre cloth (lint-free). Thereafter, sample material was freeze-dried and sample material of kidney, liver and muscle tissue further homogenised using a conical Corning® 15 mL centrifuge tube. For microwave digestion, 0.3 g of the respective sample material was weighed into Teflon extraction vessels of the microwave device (Ultraclave II, MLS, Leutkirch im Allgäu, Germany). The sample material was mixed with 500 µL internal standard solution (containing yttrium ions [0.5 mg/L]), 3 mL purified water (Milli-Q water purification equipment [Merck Millipore, Darmstadt, Germany]) and 5 mL concentrated nitric acid (69%, self-distilled [Merck, Darmstadt, Germany]) for microwave digestion following the program according to Supplementary Table S1. Samples of kidney, liver and muscle tissue were prepared as triplicate aliquots. Since bone tissue could not be further homogenised after freeze-drying, sextuplicate sample aliquots were measured to ensure sufficient sample homogeneity. Digested samples were transferred to a 50 mL Corning® centrifuge tube and diluted to a final volume of 50 mL with 3.5% nitric acid (containing 200 µg/L gold ions). At least two blanks containing 500 µL internal standard solution, 3 mL purified water and 5 mL concentrated nitric acid were digested following the same protocol in each microwave digestion.
The release of cadmium, arsenic and lead was determined by ICP-MS (iCap Q ICP-MS, Thermo Fisher Scientific). Arsenic and cadmium were measured with the collision cell technology with kinetic energy discrimination (KED) using helium with 7% hydrogen as collision gas. Lead was measured in standard mode without collision gas. The elements, isotopes and their corresponding internal standards as well as element concentrations at calibration levels that were used for quantification are shown in Supplementary Tables S2 and S3, respectively. ICP-MS system parameters are summarised in Supplementary Table S4. To account for dilution inaccuracies during sample preparation, the internal yttrium standard was used to calculate a correction factor. A mixed internal standard solution (rhodium and bismuth [5 μg/L each] in 3.5% nitric acid containing 10% isopropanol) was used as the injection standard with final concentrations of 1 μg/L per ion, and the respective sample solution was diluted in 3.5% nitric acid immediately before nebulisation via the autosampler system (prepFAST [Elemental Service & Instruments GmbH, Mainz, Germany]). The autosampler system conducted duplicate determination and automatically diluted the samples to be measured at dilutions of 1:10 and 1:2 revealing four measured values per sample. Methods were validated and limits of detection (LOD) and quantification (LOQ) were calculated according to DIN 32,645:2008–11 using equidistant calibration levels ranging from lowest to highest concentrations (cadmium, arsenic, lead: 0.01–0.18 μg/L; or 0.04–0.36 µg/L for matrices with elevated background levels of the respective metals) (Supplementary Table S5). Therefore, digested samples of each sample matrix (kidney, liver muscle and bone tissue) of pigs in the negative control group (NC) were pooled and spiked with the respective analyte standards. To assess sample homogeneity for individual sample matrices, the standard uncertainty component for the within-laboratory reproducibility (see Supplementary Table S5) was determined and found to be in accordance with ISO 13,582 and the IUPAC Harmonized Protocol. Outliers were identified via Cochran and Grubbs tests. Outliers were not included in homogeneity assessments. Measured element concentrations that fell below the lowest calibration level were quantified if the recoveries of the matrix-calibration (at significantly lower calibration levels) used to determine LOD/LOQ showed recoveries between 80 and 120%. Data were processed with the Qtegra Software (version 2.10.3324.131, 64 Bit Thermo Fisher Scientific) and Microsoft Excel (version 16.56, 2021).
2.3. Statistical analyses
2.3.1. ICP-MS data
Duplicate measurements of sample preparations of the 1:10 and 1:2 dilutions were corrected by subtracting the mean of blanks measured during the same sequence. Subsequently, mean values of the triplicate and sextuplicate aliquots for kidney, liver, muscle and bone tissue, respectively, were calculated. Values below LOD and LOQ (Supplementary Table S5) were set to 0.5 LOD and 0.5 LOQ, respectively. If a combination of element/matrix/material revealed no quantifiable concentrations >LOQ for at least one group, this combination was excluded from evaluation. For linking element intake to element concentrations in tissue, linear regression models were fitted for each combination of element/matrix/material. Data from the NC, PC10 and PC20 groups were used to estimate peat intake, data from the NC, PC3 and PC5 groups were used to estimate powder intake. Fits with a p-value above 0.05 and with an adjusted R2 of less than 0.75 were not considered meaningful. These models were used to estimate the amount of ingested material for each animal, leading to several estimates of the same variable.
The amount of ingested material was also estimated group-wise for each combination element/matrix/material with a 95% confidence interval using the R-package “chemCal” (Ranke Citation2022). The total amount of material intake per pig (mean and individual values) for the respective material was divided by total amount of feed intake per pig during the test period (173 kg) to obtain material intake as percentage of the daily feed ration.
2.3.2. Calculation of material intake using n-alkane C27 and acid insoluble ash as internal markers
In the current study, the n-alkane C27 in pig faeces was identified as a biomarker reliably indicating peat intake by pigs (see below). Regression analysis across controls (C27 in faeces of pigs in the control groups NC, PC10 and PC20) revealed a coefficient of determination of R2 = 0.87 and thus reliably generated estimates for peat intake in the treatment group TPeat based on the respective content of C27 in the respective faecal samples (group bulk samples). The same procedure was conducted to estimate voluntary intake of disinfectant powder in the experimental group TPow using AIA in faecal samples of pigs in experimental groups NC, PC3, PC5 and TPow (R2 = 0.93).
2.3.3. Calculations of material intake using TiO2 as an external marker
Disinfectant powder was supplemented with TiO2 (5 g/kg) as an external marker to investigate whether common marker techniques (Jagger et al. Citation1992; Kavanagh et al. Citation2001) reveal results comparable to the toxic metal approach. Based on the total tract digestibility of organic matter in feed, the total amount of faeces per pig and day was calculated. Subsequently, based on the concentration of TiO2 in pigs’ faeces, the ingested amount of disinfectant powder was calculated.
Organic matter digestibility (OMD) and total amount of faeces (TF) per pig and day were calculated as follows:
where OMD (100 – crude ashD) and AIAD is the concentration of organic matter and acid insoluble ash in the diet and OMF (100 – crude ashF) and AIAF respective concentrations in faeces (values referred to DM).
Total material intake in g (MI[g]) was calculated as follows:
where TiO2 F and TiO2 DP is the concentration of titanium dioxide in faeces and disinfectant powder, respectively (values referred to DM).
Total material intake divided by feed intake (referred to original substance) revealed material intake as percentage of the daily ration (MI[%]):
3. Results
Feed intake was not affected by peat and disinfectant powder added to the diet in the positive control groups. Presentation of peat and disinfectant powder in control and test groups revealed no adverse effects. An overall average daily gain of 0.93 ± 0.10 kg and average daily feed intake of 2.14 ± 0.71 kg during the adaptation and test period revealed a feed conversion ratio of 2.34 ± 0.28. Final BW of pigs was lowest in group PC10 and PC20 most likely due to lower metabolisable energy in their diet (13.0–13.4 MJ/kg) compared to the other experimental groups (14.0–14.4 MJ/kg) (). Initial and final BW, average daily gain, average daily feed intake and feed conversion ratio for each experimental group are shown in Supplementary Table S6.
Concentration of toxic metals and trace elements in the experimental diet as well as peat and disinfectant powder are presented in . For all groups, the level of toxic metals in the experimental diet was below maximum contents for feed (EU Citation2002). However, content of arsenic and lead in peat (6.70 and 12.0 mg/kg [88% DM]) and content of cadmium and lead in disinfectant powder (7.70 and 58.0 mg/kg [88% DM]) exceeded maximum levels according to feed law regulations (EU Citation2002, Citation2017, Citation2019). Regarding the trace elements, the content of iron in peat and disinfectant powder (7,943 and 22,472 mg/kg [88% DM]) exceeded maximum levels as they are set for complete feed (EU Citation2003). Levels for copper in disinfectant powder as well as the experimental diets containing the respective material (126–4,135 mg/kg [88% DM]) exceeded European thresholds for complete feed (EU Citation2018).
3.1. Material intake estimations
3.1.1. Toxic metals in animal tissues
Linear regression analysis was performed using known total element intake (calculated: element concentration in feed [fresh weight] × total feed intake) (Supplementary Table S7) and element tissue levels of pigs in the experimental control groups NC, PC10 and PC20 as well as NC, PC3 and PC5 during the test period. Concentrations of cadmium, arsenic and lead in kidney, liver, muscle and bone tissue for each experimental group are shown in Supplementary Table S8. A regression across controls for the respective elements and animal tissues with a coefficient of determination R2≥0.75 and p-value <0.05 was assumed as suitable for material intake estimation. Thus, based on element tissue levels in the treatment groups TPeat and TPow (Supplementary Table S8) in relation to the control groups with known quantity of element intake, material intake of the four individual pigs in the respective treatment group was estimated. Regression analysis and material intake estimations using cadmium, which was set as main target value, as marker in kidney and liver are shown in . However, levels of cadmium in peat and accordingly in pig tissue were too low to reveal clear differences in feed mixtures for all control groups (NC, PC10 and PC20) ( and S8). Results of regression analysis and material intake estimations based on arsenic and lead accumulation in pig tissues are shown in Supplementary Figure S2. Mean material intake estimation ranged from 3.1–7.0% peat and 0.5–1.9% disinfectant powder of the daily ration, respectively (). However, individual material intake estimations revealed varying intake levels for each pig (Supplementary Figure S2 and S3).
Figure 2. Regression analysis using cadmium levels in kidney (a) and liver (b) of pigs in negative control (NC) and positive control groups for peat (Peat [PC10, PC20]) and disinfectant powder (Powder [PC3, PC5]); × - estimated intake levels based on regression equations from voluntary material consumption by individual pigs in the treatment groups (TPeat and TPow); R2 adjusted.
![Figure 2. Regression analysis using cadmium levels in kidney (a) and liver (b) of pigs in negative control (NC) and positive control groups for peat (Peat [PC10, PC20]) and disinfectant powder (Powder [PC3, PC5]); × - estimated intake levels based on regression equations from voluntary material consumption by individual pigs in the treatment groups (TPeat and TPow); R2 adjusted.](/cms/asset/32aaf00a-a380-46f5-b784-e9171d10dbe2/gaan_a_2175537_f0002_oc.jpg)
Table 3. Estimated material intake (mean ±95% confidence interval) of peat and disinfectant powder by pigs in the treatment groups.
Estimation of mean voluntary intake of peat was highest using arsenic as marker in kidney, liver and muscle and using lead as marker in kidney and liver. It was lower using arsenic and lead as marker in bone tissue (). However, individual intake estimations for each pig differed widely and were found in a range of 1.7–34.0 kg total peat intake per pig corresponding to 1.0%−19.7% of the daily ration (Supplementary Figure S3-A/C/E). Estimation of mean voluntary intake of disinfectant powder was at a similar level using cadmium and arsenic as a marker in kidney, liver and muscle (). Individual intake estimations for each pig differed in a range of 0.4–5.8 kg total disinfectant powder intake per pig corresponding to 0.2%−3.4% of the daily ration (Supplementary Figure S3-B/D/F).
3.1.2. Additional markers for material intake
Besides toxic metals in pig tissues, n-alkanes and AIA naturally occurring in peat and disinfectant powder were analysed in pig faeces to prove and further quantify material consumption by comparison of experimental groups. Also, analysis of TiO2, supplemented to disinfectant powder, in pig faeces was suitable to estimate disinfectant powder intake based on digestibility calculations. In brief, n-alkane and AIA analysis revealed material intake estimations in a range of 7.1–14.0% and 0.1–3.8% of the daily ration for peat and disinfectant powder, respectively (Supplementary Figure S4 and S5). Calculation of material intake using TiO2 as external marker in disinfectant powder revealed an intake level ranging from 0.5–2.7% of the daily ration (Supplementary Table S9). Generally, material intake estimations based on these parameters indicate similar results as obtained by toxic metal analysis. More detailed results regarding n-alkane-, AIA- and TiO2-analysis are available in the Supplementary Material S1-A.
4. Discussion
4.1. Material intake estimations
4.1.1. Toxic metals in animal tissues
Cadmium accumulation is highest in kidney and liver whereby Hoogenboom et al. (Citation2014) found cadmium levels in the kidney being four times as high as cadmium levels in liver (referred to wet weight). Our analyses showed levels of cadmium in kidney exceeding cadmium levels in liver by a factor of 7 up to 11 (referred to DM). An explanation for these elevated kidney levels could be that lead, which was also found at considerable levels in the materials and thus feed mixtures, increases cadmium tissue levels (Phillips et al. Citation2003). Absorption rate of cadmium in pigs is low (5% of dietary intake for organic and inorganic cadmium) (NRC Citation2005; Hoogenboom et al. Citation2014). However, it is excreted at a rate of <0.01% of the total body burden via urine and faeces. Hence, cadmium accumulation in tissues is considered to be a quasi-irreversible process and relative tissue cadmium levels would only decrease by further organ growth once exposure has stopped (NRC Citation2005; Hoogenboom et al. Citation2014). Muscle and bone tissues do not accumulate cadmium at high levels (NRC Citation2005) and likewise to Hoogenboom et al. (Citation2014) cadmium levels were below LOQ in muscle as well as in bone tissue. Cadmium concentration in peat was not suitable for material intake estimation but in disinfectant powder. Likewise, Hansen et al. (Citation1981), who analysed contaminants in pig tissues that originated from soil intake, found cadmium as an element to accumulate in relation to the contaminant-load of the ingested soil.
Arsenic in its inorganic form is highly toxic and absorption increases with water solubility (up to 90%), whereas organic arsenic is less well absorbed. Furthermore, absorption varies with animal age, health and species (EFSA Citation2005; Constable et al. Citation2016; Mandal Citation2017). However, Liao et al. (Citation2020) concluded on a generally low absorption rate as arsenic concentrations in faeces increased with the dietary level. Still, excretion and reabsorption of arsenic in the enterohepatic cycle may influence faecal element concentrations. Arsenic concentration is highest in kidney and liver and in tissues with high keratin levels (hair, nail, skin) due to the ability of arsenic to bind to sulphhydryl groups of keratin (NRC Citation2005) and lower in muscle tissue of pigs receiving the peat treatment (PC10, PC20 and TPeat) in accordance with previous studies (López-Alonso et al. Citation2007; Liao et al. Citation2020). However, arsenic levels in muscle of pigs receiving no (NC) and the disinfectant powder treatment (PC3, PC5 and TPow), respectively, tended to be higher than in liver. Similar results were reported in small mammals (Ismail and Roberts Citation1992). Although arsenic is readily excreted via urine and bile (NRC Citation2005), arsenic content in pig tissues was well suitable for material intake estimations.
Absorption of lead from the gastrointestinal tract varies widely with dose, solubility, age and health status of the animal but may reach up to 80% of the ingested dose. It is initially bound to proteins (e.g. metallothionein) in soft tissues with the kidney and brain being main target tissues (NRC Citation2005). Lead is redistributed from soft to bone tissue where it replaces calcium during bone growth and remodelling forming stable complexes with phosphate (NRC Citation2005). Because of a slow excretion rate of lead (half-life of about one month in soft tissues, longer in bone tissue), it accumulates in the body, especially during long-term exposure. Our results revealed highest levels of lead in bone and lowest levels in muscle tissue in accordance with other findings (Phillips et al. Citation2003; López-Alonso et al. Citation2007). Tissue levels of pigs in groups receiving the disinfectant powder treatment (NC, PC3, PC5 and TPow) did not reflect respective dietary and material element levels. One explanation might be a lower bioavailability of lead in disinfectant powder than in peat.
Studies investigating the intake of enrichment and bedding materials by pigs and other livestock are rare. To our knowledge, this is the first study investigating the quantitative intake of peat and disinfectant powder by pigs. A previous study investigated the consumption of straw, rice hulls and sawdust as bedding materials for pigs and found higher mean intake levels of these materials compared to our data (10–14% of the daily ration) (van Barneveld Citation2012). Here, calculations of peat intake for individual pigs revealed an intake up to 19.7% of the daily ration. Investigations on soil consumption by pigs might yield intake estimations comparable to that obtained with disinfectant powder, since both are inorganic materials. Pigs kept in bare soil ingested soil at a rate of 1.2–5.7% of DM intake (Fries et al. Citation1982), which is higher than in the current study. Still, maximum intake levels of disinfectant powder by individual pigs reached up to 3.4% of the daily ration.
As consumption of enrichment and bedding materials occurs, contaminants might transfer to animal products. Thus, early and recent studies showed a transfer of pesticides, heavy metals and persistent organic pollutants, such as polychlorinated biphenyls as a result of soil and bedding material consumption by pigs (Hansen et al. Citation1981; Fernandes et al. Citation2019). Fernandes et al. (Citation2019) thus emphasised the need of knowledge about material ingestion levels.
4.1.2. Additional markers for material intake
We found that results of material intake estimations of n-alkane, AIA- and TiO2-analysis were in a similar range as material intake estimations based on toxic metal analysis in pig tissues. Generally, the accordance of results of these multi-marker-investigations underlines the validity of the current intake estimations and suitability of different internal and external markers to estimate peat and disinfectant powder intake by pigs. However, analysis of n-alkanes, AIA and TiO2 in pig faeces are only representative for the 24 hours prior to faecal sampling. Thus, estimations based on element levels in pig tissues, which take the total test period into account, might be more reliable and proved average long-term consumption rates. A more detailed discussion on these additional markers for material intake is available in the Supplementary Material S1-B.
4.2. Feed and food safety
The levels of lead in peat and of cadmium and lead in disinfectant powder exceeded maximum limits as they are set for complementary and mineral feed, respectively (EU Citation2002, Citation2017). Although a transfer to animal tissues occurred for all toxic metals, maximum levels of cadmium and lead in meat (0.05 and 0.10 mg/kg), liver (0.50 mg/kg) and kidney (1.00 and 0.50 mg/kg) according to Commission Regulation (EC) No 1881/2006 on contaminant levels in foodstuffs were not exceeded (EU Citation2006). Assuming maximum element levels in peat and disinfectant powder according to Koch et al. (Citation2021), who analysed a large number of enrichment and bedding material samples for toxic metal and trace element contents, element intake by pigs during the fattening period may be calculated: Assuming a total feed intake of 200 kg per pig, an upper intake level of 7% peat and 2% disinfectant powder of the daily ration (corresponding to the total intake of 14 kg peat and 4 kg disinfectant powder) and DM contents of 42 and 98% for peat and disinfectant powder, respectively, the following amounts of toxic metals would be ingested by pigs during the fattening period: 5 and 11 mg cadmium, 50 and 21 mg arsenic and 232 and 790 mg lead for peat and disinfectant powder intake, respectively. In this case, total lead intake through the consumption of disinfectant powder would exceed the maximum lead intake in the current study. Despite a possible exceedance of current maximum levels for i.e. arsenic and lead in feed, it should be noted that health-based guidance level have yet been derived not for all of these elements (EFSA Citation2005). Thus, any dietary exposure should be reduced to a minimum (ALARA principle [As Low As Reasonable Achievable]).
Regarding the content of trace elements, peat and disinfectant powder contained high levels of iron and copper but only feed mixtures containing disinfectant powder exceeded maximum limits for copper in complete feed (EU Citation2018). Whether these high levels of iron and copper in both materials might be a concern for food safety of animal products may be subject of future research. However, trace elements underly strict homoeostatic regulation in the animal body and accumulation in edible tissues may not be the major concern (NRC Citation2005; EFSA Citation2016). Additionally, it should be focused on animal health effects (such as disturbance of mineral and trace element absorption and secondary copper deficiency at high iron levels) and environmental concerns of high copper levels (such as increased antibiotic resistance at high copper levels) (NRC Citation2005; EFSA Citation2016; Wang et al. Citation2020). The most effective way to minimise copper output from livestock farming into the food chain and the environment is to reduce maximum limits in animal feed (EFSA Citation2016). Although feed law regulations are not applicable for disinfectant powder, this material contributes to the daily ration of pigs and thus possibly interferes with feed and food safety and may also contribute to environmental pollution. As highlighted in the current and previous studies (van Barneveld Citation2012; Fernandes et al. Citation2019; Koch et al. Citation2022) enrichment and bedding materials are ingested by pigs and thus contribute to the daily ration. A classification as animal feed and application of respective regulations for feed safety might be a way to ensure animal and consumer health and further to reduce the contamination of the environment.
4.3. Further aspects
Voluntary material intake was higher and varied more for individual pigs for peat (1.0–19.7% of the daily ration) than for disinfectant powder (0.2–3.4% of the daily ration). Material intake resulting from an inadequate feeding regime can be excluded since all pigs received a balanced diet ad libitum and in accordance with nutritional and energy requirements of pigs. Additionally, previous studies found material intake to be independently from feed intake (van Barneveld Citation2012; Kauselmann et al. Citation2021). Peat is the preferred enrichment material of pigs, whereas disinfectant powder was the least preferred material (Pedersen et al. Citation2005; Koch et al. Citation2022). Exploration as part of foraging behaviour of pigs includes chewing and biting. Initial exploration does not necessarily intend feeding, but palatability and nutritional feedback of the material might lead to consumption (Day et al. Citation1996). Based on our results, we assume peat to be more palatable than disinfectant powder. Furthermore, peat is known to be of greater interest for pigs than disinfectant powder. Thus, the pigs’ preference for this material is likely to enhance consumption (Holm et al. Citation2008; Kauselmann et al. Citation2021; Koch et al. Citation2022). However, overall contaminant levels in disinfectant powder were higher than in peat in the current and previous studies (Koch et al. Citation2021). Furthermore, as intake levels of the respective material differed for individual pigs, mean intake levels as basis of exposure assessment of animals and further of consumers must be treated with caution. To ensure food safety, the highest intake levels should be considered for risk assessment.
5. Conclusion
Pigs consume up to 7% peat and 2% disinfectant powder of their daily ration (referred to mean intake levels). Thus, enrichment and bedding materials can considerably contribute to their diet. A transfer of undesirable substances originating from these materials into animal tissues is likely and might impact food safety. Exposure assessment for humans is a next step in risk assessment of enrichment and bedding materials in livestock housing. Further, labelling guidelines or classification of materials with intended use in livestock housing as animal feed might limit the entry of toxic metals and trace elements into the environment.
Ethics approval
Ethical approval for all procedures involving animal handling and treatment was obtained from the Regional Office for Health and Social Affairs Berlin (LaGeSo, Berlin, Germany; Registration number 0123/20). All procedures were carried out following institutional and national guidelines for the care and use of animals in accordance with the German legislation and the EU Directive 2010/63/EU for animal experiments.
Supplemental Material
Download PDF (1.6 MB)Acknowledgments
We would like to thank Carola Fischer-Tenhagen, Stefanie Banneke, Dirk Meyer and all animal keepers for their valuable support during the animal experiment. Many thanks also to Philipp Reichardt for excellent assistance in sample preparation related to ICP-MS analyses. We are also very grateful to Anja Lüth, Christoph Wilming and Lisa Klusmann for the provision of laboratory space and equipment for freeze-drying of sample material. Many thanks also to Dave Brüning of the state laboratory Berlin-Brandenburg for his support in acid insoluble ash analysis.
Disclosure statement
No potential conflict of interest was reported by the author(s).
Data availability statement
The datasets used and/or analysed during the current study are available from the corresponding author on reasonable request.
Supplementary material
Supplementary data for this article can be accessed at https://doi.org/10.1080/1745039X.2023.2175537.
Additional information
Funding
References
- Constable PD, Hinchcliff KW, Done SH, Grünberg W. 2016. Diseases of the alimentary tract: nonruminant. In: Constable PD, Hinchcliff KW, Done SH Grünberg W, editors. Veterinary medicine: a textbook of diseases cattle, horses, sheep, pigs, and goats. St. Louis, Missouri, USA: Elsevier; p. 175–435.
- Day JEL, Kyriazakis I, Lawrence AB. 1996. An investigation into the causation of chewing behaviour in growing pigs: the role of exploration and feeding motivation. Appl Anim Behav Sci. 48:47–59.
- EFSA. 2005. Opinion of the scientific panel on contaminants in the food chain on a request from the commission related to arsenic as undesirable substance in animal feed. EFSA J. 180:1–35.
- EFSA. 2016. Revision of the currently authorised maximum copper content in complete feed. EFSA J. 14:4563.
- Elwert C, Kluth H, Rodehutscord M. 2004. Effect of variable intake of alfalfa and wheat on faecal alkane recoveries and estimates of roughage intake in sheep. J Agric Sci. 142:213–223.
- EU. 2002. Directive 2002/32/EC of the European Parliament and of the council of 7 May 2002 on undesirable substances in animal feed. Off J Eur Commun. L140:10–21.
- EU. 2003. Commission regulation (EC) No 1334/2003 of 25 July 2003 amending the conditions for authorisation of a number of additives in feedingstuffs belonging to the group of trace elements. Off J Eur Union. L187:11–15.
- EU. 2006. Commission regulation (EC) No 1881/2006 of 19 December 2006 setting maximum levels for certain contaminants in foodstuffs. Off J Eur Union. L364:5–24.
- EU. 2009a. Commission regulation (EC) No 152/2009 of 27 January 2009 laying down the methods of sampling and analysis for the official control of feed. Off J Eur Union. L54:1–130.
- EU. 2009b. Council directive 2008/120/EC of 18 December 2008 laying down minimum standards for the protection of pigs (codified version). Off J Eur Union. L47:5–13.
- EU. 2016. Commission recommendation (EU) 2016/336 of 8 March 2016 on the application of council directive 2008/120/EC laying down minimum standards for the protection of pigs as regards measures to reduce the need for tail-docking. Off J Eur Union. L62:20–22.
- EU. 2017. Commission regulation (EU) 2017/2229 of 4 December 2017 amending annex I to directive 2002/32/EC of the European parliament and of the council as regards maximum levels for lead, mercury, melamine and decoquinate (text with EEA relevance). Off J Eur Union. L319:6–9.
- EU. 2018. Commission implementing regulation (EU) 2018/1039 of 23 July 2018 concerning the authorisation of copper(II) diacetate monohydrate, copper(II) carbonate dihydroxy monohydrate, Copper(II) chloride dihydrate, copper(II) oxide, copper(II) sulphate pentahydrate, Copper(II) chelate of amino acids hydrate, copper(II) chelate of protein hydrolysates, copper(II) chelate of glycine hydrate (solid) and copper(II) chelate of glycine hydrate (liquid) as feed additives for all animal species and amending regulations (EC) No 1334/2003, (EC) No 479/2006 and (EU) No 349/2010 and implementing regulations (EU) No 269/2012, (EU) No 1230/2014 and (EU) 2016/2261. Off J Eur Union. L186:3–24.
- EU. 2019. Commission regulation (EU) 2019/1869 of 7 November 2019 amending and correcting annex I to directive 2002/32/EC of the European parliament and of the council as regards maximum levels for certain undesirable substances in animal feed. Off J Eur Union. L289:32–36.
- Fernandes AR, Lake IR, Dowding A, Rose M, Jones NR, Petch R, Smith F, Panton S. 2019. The potential of recycled materials used in agriculture to contaminate food through uptake by livestock. Sci Total Environ. 667:359–370.
- Fries GF, Marrow GS, Snow PA. 1982. Soil ingestion by swine as a route of contaminant exposure. Environ Toxicol Chem. 1:201–204.
- Hansen LG, Washko PW, Tuinstra LGMT, Dorn SB, Hinesly TD. 1981. Polychlorinated biphenyl, pesticide, and heavy metal residues in swine foraging on sewage sludge amended soils. J Agric Food Chem. 29:1012–1017.
- Holm L, Jensen MB, Pedersen LJ, Ladewig J. 2008. The importance of a food feedback in rooting materials for pigs measured by double demand curves with and without a common scaling factor. Appl Anim Behav Sci. 111:68–84.
- Hoogenboom RLAP, Hattink J, van Polanen A, van Oostrom S, Verbunt JT, Traag WA, Kan KA, van Eijkeren JCH, De Boeck G, Zeilmaker MJ. 2014. Carryover of cadmium from feed in growing pigs. Food Addit Contam. 32:68–79.
- Ismail A, Roberts RD. 1992. Arsenic in small mammals. Environ Technol. 13:1091–1095.
- Jagger S, Wiseman J, Cole DJA, Craigon J. 1992. Evaluation of inert markers for the determination of ileal and faecal apparent digestibility values in the pig. Br J Nutr. 68:729–739.
- Kamphues J, Wolf P, Coenen M, Eder K, Iben C, Kienzle E, Liesegang A, Männer K, Zebeli Q, Zentek J. 2014. Ernährung verschiedener Spezies (Schweine). In: Kamphues J, Wolf P, Coenen M, Eder K, Iben C, Kienzle E, Liesegang A, Männer K, Zebeli Q, Zentek J, editors. Supplemente zur Tierernährung für Studium und Praxis. Hannover, Germany: M. & H. Schaper; p. 371.
- Kauselmann K, Krause ET, Glitz B, Gallmann E, Schrade H, Schrader L. 2021. Effect of plant-based enrichment materials on exploration in rearing and fattening pigs (Sus scrofa domesticus). Appl Anim Behav Sci. 236:105261.
- Kavanagh S, Lynch PB, O’mara F, Caffrey PJ. 2001. A comparison of total collection and marker technique for the measurement of apparent digestibility of diets for growing pigs. Animal Feed Sci Technol. 89:49–58.
- Kittawornrat A, Zimmerman JJ. 2011. Toward a better understanding of pig behavior and pig welfare. Animal Health Res Rev. 12:25–32.
- Koch F, Kowalczyk J, Mielke H, Schenkel H, Bachmann M, Zeyner A, Leinweber P, Pieper R. 2022. Preference and possible consumption of provided enrichment and bedding materials and disinfectant powder by growing pigs. Porcine Health Manage. 8:1.
- Koch F, Kowalczyk J, Wagner B, Klevenhusen F, Schenkel H, Lahrssen-Wiederholt M, Pieper R. 2021. Chemical analysis of materials used in pig housing with respect to the safety of products of animal origin. Animal. 15:100319.
- Liao SF, Hasan MS, Yang Z, Stevens AW, Brett J, Peng Z. 2020. Feeding arsenic-containing rice bran to growing pigs: growth performance, arsenic tissue distribution, and arsenic excretion. Int J Environ Res Public Health. 17:8530.
- López-Alonso M, Miranda M, Castillo C, Hernández J, García-Vaquero M, Benedito JL. 2007. Toxic and essential metals in liver, kidney and muscle of pigs at slaughter in Galicia, north-west Spain. Food Addit Contam. 24:943–954.
- Mandal P. 2017. An insight of environmental contamination of arsenic on animal health. Emerging Contam. 3:17–22.
- Mayer C, Hillmann E, Schrader L. 2006. Verhalten, Haltung, Bewertung von Haltungssystemen. In: Brade W Flachowsky G, editors. Schweinezucht und Schweinefleischerzeugung Empfehlungen für die Praxis. Braunschweig, Germany: FAL Agricultural Research; p. 94–108.
- Myers WD, Ludden PA, Nayigihugu V, Hess BW. 2004. Technical note: a procedure for the preparation and quantitative analysis of samples for titanium dioxide. J Anim Sci. 82:179–183.
- NRC. 2005. Mineral tolerance of animals. Washington, D.C., USA: The National Academic Press.
- Pedersen LJ, Holm L, Jensen MB, Jørgensen E. 2005. The strength of pigs’ preferences for different rooting materials measured using concurrent schedules of reinforcement. Appl Anim Behav Sci. 94:31–48.
- Phillips C, Győri Z, Kovács B. 2003. The effect of adding cadmium and lead alone or in combination to the diet of pigs on their growth, carcase composition and reproduction. J Sci Food Agric. 83:1357–1365.
- Pieper R, Taciak M, Pieper L, Święch E, Tuśnio A, Barszcz M, Vahjen W, Skomiał J, Zentek J. 2016. Comparison of the nutritional value of diets containing differentially processed blue sweet lupin seeds or soybean meal for growing pigs. Animal Feed Sci Technol. 221:79–86.
- Ranke J. 2022. chemCal: calibration functions for analytical chemistry. https://cran.r-project.org/package=chemCal.
- Taylor NR, Main DCJ, Mendl M, Edwards SA. 2010. Tail-biting: a new perspective. Vet J. 186:137–147.
- van Barneveld R. 2012. Nutritional strategies to minimise the influence of bedding material consumption on growth efficiency. Final Report APL Project 2005/2012. Canberra, Australia: Australian Pork Limited.
- VDLUFA, editor. 2012. Bestimmung von salzsäureunlöslicher Asche - Amtliche Methode. In: Method book volume 3 of VDLUFA: the chemical analysis of feedstuffs. Darmstadt, Germany: VDLUFA; p. 1–4.
- Wang Q, Liu L, Hou Z, Wang L, Ma D, Yang G, Guo S, Luo J, Qi L, Luo Y. 2020. Heavy metal copper accelerates the conjugative transfer of antibiotic resistance genes in freshwater microcosms. Sci Total Environ. 717:137055.
- Wechsler B, Schmid H, Moser H. 1991. Das Verhalten von Hausschweinen in einem Freigehege. In: Wechsler B, Schmid H Moser H, editors. Der Stolba-Familienstall für Hausschweine. Basel: Birkenhäuser; p. 9–20.