Abstract
The phylogenetic relationships between Tc1 transposable elements have previously been reported for the genomes of some fish species. However, research in this field has been hindered by the low number of fish genome sequences available in databases. The application of a DNA microarray as a universal tool for Tc1 transposon sequence analysis in fish genomes is described here. A prototype oligonucleotide microarray was constructed and used to compare samples of genomic DNA isolated from selected fish species. These results, combined with earlier reported molecular analysis of Tc1 showed the usefulness of DNA microarray in the screening of transposon sequences.
Keywords:
Published in collaboration with the University of Bergen and the Institute of Marine Research, Norway, and the Marine Biological Laboratory, University of Copenhagen, Denmark
Introduction
Transposable elements (TEs) are important components of genomes (Almeidaa et al. Citation2007). TEs can constitute up to half of the genome mass in vertebrates (Zamudio & Bourc'his Citation2010). Class I elements are retro-elements, which are very common in genomes. Class II elements are DNA transposons and account for a small percentage of the genome. Their role in evolution and functioning of genomes is profound (Miller & Capy Citation2006; Munoz-Lopez & Garcia-Perez Citation2010). DNA transposons are characterized by high variability, which render potential for research and biotechnological applications. Therefore, methods for their detection in different taxa of organisms will be useful and should be improved.
The characteristic feature of DNA transposons is the presence of inverted terminal repeats (ITRs) that flank sequences encoding transposases. The Tc1-like/mariner superfamily of transposons is the most widely distributed group of transposons in animals from nematodes to vertebrates and fungi (Plasterk et al. Citation1999; Ogasawara et al. Citation2009). Tc1-like elements have 54 bp ITRs flanking the transposase gene (Leaver Citation2001; Pocwierz-Kotus et al. Citation2007). Transposons with an intact transposase gene are active and have the ability to excise themselves and move to another site in the genome. Deletions, insertions or base substitutions within this gene inactivate the transposon, which then becomes a permanent component of the genome (a genetic fossil).
Active transposons increase copy numbers within a genome and spread through populations afterwards by the processes of sexual reproduction (vertical transmission). Another form of transposon transmission – from a host species to a different, reproductively isolated species – is a horizontal transfer. A high sequence similarity between Tc1 transposons in some fish species can be related to horizontal transfer (Leaver Citation2001; Pocwierz-Kotus et al. Citation2007). However, it is not known how frequently the horizontal transfer of Tc1 elements in the evolutionary history of vertebrates, including fish, occurs.
A general problem of research in this field of animal relationships is the limited number of complete genomes in public repositories (Hammer et al. Citation2005; Kulski et al. Citation2005; Kurland Citation2005). Genome sequences of fish species are particularly under-represented in the vertebrate databases. The need for labour-intensive and time-consuming molecular characterization of transposons from many species is especially challenging in the study of transposon Tc1-like occurrence and horizontal transfer between different fish populations and species. One of the screening methods that can be applied in transposon identification across species is polymerase chain reaction (PCR) (Pocwierz-Kotus et al. Citation2007). However, it is not sufficient to identify all transposons, due to the frequent mutations that accumulate in the transposon sequences, which reduce primer specificity. Microarray technology can be used as a complementary and fast method to screen Tc1 transposable elements and to infer cases of Tc1 horizontal transfer between fish genomes. Microarrays enable genome-wide screening in a single experiment (Venkatasubbarao Citation2004).
DNA microarray construction is a simple procedure when the whole genome of the studied organism is known (Rouillard et al. Citation2002; Hyyrö et al. Citation2005). In this case, a set of highly specific oligonucleotides complementary to the unique fragments of the target sequences can be designed (Kane et al. Citation2000; Wang et al. Citation2003; Formanowicz et al. Citation2005). Otherwise, long PCR-amplified DNA fragments (cDNA) can be used as probes (Aharoni et al. Citation2001; Wu et al. Citation2001). cDNA usually gives strong hybridization signals, but has lower specificity than oligonucleotides. A compromise solution would be the construction of a mixed microarray, with both the oligonucleotide and long DNA probes. Such an approach (a mixed oligo/cDNA microarray) was applied by Wang et al. (Citation2003) to evaluate mRNA profiles in the rat heart and brain. A prototype DNA microarray is presented here, which was designed, constructed, and used for Tc1 transposon sequence identification in selected fish species inhabiting the Baltic Sea and Polish lakes. Species selection was based on the previous screen for Tc1-like sequences using PCR and Sanger sequencing (Pocwierz-Kotus et al. Citation2007).
Materials and methods
Bioinformatic analysis of Tc1-like sequences
The analysis encompassed 31 selected Tc1 transposon sequences from the genomes of 14 fish species (): seven sequences from the model species zebra fish (Danio rerio Hamilton, 1822), one from pearl danio (Danio albolineatus Blyth, 1860), four from salmon (Salmo salar Linnaeus, 1758), four from rainbow trout (Oncorhynchus mykiss Walbaum, 1792), one from Chinook salmon (Oncorhynchus tschawytscha Walbaum, 1792), three from pike (Esox lucius Linnaeus, 1758), one from carp (Cyprinus carpio Linnaeus, 1758), one from goldfish (Carassius auratus Linnaeus, 1758), one from harlequin rasbora (Rasbora heteromorpha Duncker, 1904), one from white cloud mountain minnow (Tanichthys albonubes Lin, 1932), one from kuhli loach (Acanthophthalmus kuhli Ladiges, 1934), three from plaice (Pleuronectes platessa Linnaeus, 1758), four from flounder (Platichthys flesus Linnaeus, 1758) and two from turbot (Scophthalmus maximus Linnaeus, 1758). The sequences were aligned using MEGA 3.1 software in order to identify conserved and variable regions.
Table I. List of oligonucleotide probes and transposon sequences.
Probe design
The microarray probes were designed on the basis of the 31 sequences described above. An algorithm elaborated in the Institute of Computing Science of Poznan University of Technology, Poland generated probes that complied with the following criteria: (1) probe length 45–55 nt; (2) probe melting temperature 70.5°C±1.5°C; (3) restrictions on composition: (a) nucleotides of one type should not compose more than 40% of the probe sequence, (b) blocks composed of more than eight nucleotides of the same type must not be present in the probe, (c) number of C/G nucleotides should be within the range of 30–70% of the probe length (Formanowicz et al. Citation2005, Citation2008). The candidate probes for which complex secondary structures were predicted using the mFold (Zuker et al. Citation1999) software were discarded.
Once a set of candidate probes had been designed for each of the 31 target Tc1-like sequences, the complementarity of each candidate probe to the remaining sequences was determined. To this end, each candidate probe was moved by one nucleotide along each non-target sequence and for all these comparisons Hamming distances (Garbarine & Rosen Citation2008) were calculated. The smallest value of the Hamming distance was used as a measure of probe complementarity to a given non-target sequence. Finally, the two probes with the largest scores and complementary to two different regions of each target sequence were selected from every set ().
Microarray printing
The oligonucleotide probes were dissolved in water and diluted in Nexterion Spot buffer (Schott Nexterion) to a 20 µM concentration. As a positive control, 16 long PCR probes of different length (200, 800 and 1600 bp) were used. PCR probes were amplified for flounder, plaice, turbot and pike genomic DNA templates using three combinations of universal primers recognizing Tc1-like sequences: APK1 and APK3 (product about 200 bp); APK1 and APK2 (product about 800 bp) in Pocwierz-Kotus et al. (2007); single primer – Leaver (2001): 5'-TACAGTGCCTTGCATAAGTATTCACC-3' (product about 1600 bp). The nomenclature of the probes is presented in . A negative control was a short oligonucleotide probe, specific for the unique plant pathogenesis-related gene (pr-10) (Handschuh et al. Citation2006), pr-10.2B_1 (5'-ACGAGCTCGTGCCGCTCATATATCCT-3').
Table II. Nomenclature of PCR probes.
Finally, a microarray with both PCR and oligo probes (68 probes in total) was constructed. All probes were printed in eight repeats on epoxide slides (Schott Nexterion) with a SpotArray 24 printing system (PerkinElmer) at room temperature and 65% air humidity. The whole microarray was printed in three copies on each slide. Substrates were immobilized and blocked after printing according to the manufacturer's recommendations.
Genomic DNA extraction and labelling
Samples were collected from the Baltic Sea and lakes in Poland except for non-local samples that were obtained from the fish market and an ornamental fish shop in Gdynia, Poland in 2005–2006. The following fish species were sampled: plaice Pleuronectes platessa, turbot Scophthalmus maximus (two specimens), pike Esox lucius, perch Perca fluviatalis, salmon Salmo salar (two specimens), sea trout Salmo trutta morpha trutta Linnaeus, 1758, brown trout Salmo trutta morpha fario, round goby Neogobius melanostomus Pallas, 1814, cod Gadus morhua Linnaeus, 1758, sprat Sprattus sprattus Linnaeus, 1758, carp Cyprinus carpio and zebra fish Danio rerio (two specimens). Genomic DNA was isolated from the fish tissue samples using the DNeasy tissue kit (Qiagen) and digested with Bsp143 restriction enzyme (Fermentas). Fragmented DNA was then purified and labelled with two fluorescent dyes (Alexa 555 and Alexa 647) using BioPrime Array CGH Genomic Labeling System (Invitrogen).
Microarray hybridization
First, samples of labelled genomic DNA were hybridized in a two-colour scheme to the microarrays at 50°C for 16 h in a buffer containing 5×SSC, 0.1% SDS and 0.1 mg/ml BSA and washed with the following buffers: 1×SSC/0.1% SDS; 1×SSC and 0.1×SSC. All hybridization and washing procedures were performed in an automatic hybridization station HybArray 12 (PerkinElmer). In the process of optimization, the following changes were introduced: step-down hybridization at three different temperatures (50°C/45°C/40°C; 5.5 h each) and buffers with higher concentrations of sodium chloride/sodium citrate and SDS: 2×SSC/0.2%SDS, 2×SSC and 0.2×SSC. After hybridization, slides were dried in a stream of gaseous nitrogen.
Scanning and analysis of microarrays
Dried substrates were immediately scanned in the ScanArray Express laser scanner (PerkinElmer) at a resolution of 5 µm and wavelengths of 543 and 633 nm. For scanning and quantification, the ScanArray Express ver. 3.0 software (PerkinElmer) was applied. The step-down hybridization data (spot median foreground intensity values) were exported to Excel for simple within-array normalization. Basal intensity values obtained for the plant-specific probe (negative control) were used for dye-bias correction. After normalization (double-channel normalization between arrays based on subtraction of control probe mean, followed by log2-transformation) using Genowiz 4.0 software (Ocimum Biosolutions), the probe intensity data was analysed using R (http://www.R-project.org; R Development Core Team Citation2008). The dendrograms were obtained with hierarchical clustering and the statistical support for branches was obtained using Weightless 3.72 (Sanjuan & Wróbel Citation2005; Czarna et al. Citation2006).
Results
A prototype DNA microarray was designed and constructed in order to identify Tc1-like transposons in fish genomes. The Tc1 microarray consisted of 68 probes including 52 oligonucleotide probes of about 50-nt length, specific for the 31 known Tc-like sequences from 14 fish species living in European seas and lakes. PCR primers were designed based on fragmented sequence data that were insufficient for long oligonucleotide probe selection. Sixteen PCR products (PCR probes) amplified from genomic DNA templates of pike, plaice, flounder and turbot were also spotted on the microarray, serving as positive controls. A negative control was a short oligonucleotide probe designed for a plant-specific gene from the pr-10 (pathogen-related) gene family.
The Tc1 microarray was used to screen 15 samples of genomic DNA from 12 different fish species. For three species (Danio rerio, Salmo salar and Scophthalmus maximus) replicate samples were available. At least two technical replicates were analysed for each sample, and the results were merged and averaged. In addition, half of the samples were cross-labelled using the dye-swap method. The temperature used in the first hybridization experiment (50°C) seemed to be optimal for long DNA probes (PCR probes). As a result of the optimization process, a step-down hybridization procedure with three subsequent temperature steps (50°C/45°C/40°C) and less stringent washing was applied. The hybridization results were satisfactory. On the majority of arrays almost all probes were detected but with different levels of intensity. Two samples (from Cyprinus carpio and Pleuronectes platessa) gave noticeably weaker signals than others.
Due to the size and specific design of the microarray, a normalization procedure (dye-bias correction) based on the negative control probe (specific for a plant gene and displaying background-level intensity) was implemented using the results of dye-swap experiments. The same probe was also used for normalisation between arrays. Raw data as well as normalized and log2-transformed data for merged replicate samples were obtained.
presents the probe intensity data with hierarchical clustering (using Manhattan distance and average linkage) of Tc1 profiles for different species. Some Tc1 profiles also clustered with one another when other distance measures were used (Euclidean distance, correlation distance) or linkage method (complete instead of average). This was the case for the profiles of four Salmonidae family members and two Danio rerio individuals. In contrast, two Scophthalmus maximus profiles were placed far apart as were the profiles of two representatives of Perciformes, Perca fluviatilis and Neogobius melanostomus. Two profiles (Cyprinus carpio and Pleuronectes platessa) were clearly separated from the rest. This was caused by the DNA samples from these two species binding weakly across most of the probes.
Figure 1. Hierarchical clustering of Tc1 transposon microarray profiles of selected fish genomes. Black indicates the highest intensity of hybridization, white indicates the lowest. Probe names and target sequence origins are listed on the right, hybridized fish samples are clustered at the top using Euclidean distance and average linkage. Median probe intensities were extracted from the ScanArray Express ver. 3.0 software (PerkinElmer), normalized within and between arrays and log2-transformed in Genowiz ver. 3.1 software (Ocimum Biosolutions). Replicate probes and samples were merged.
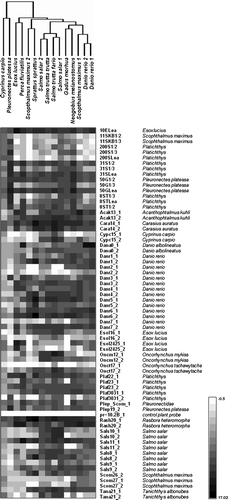
Hybridization results obtained for particular probes indicate which transposons can be more frequent in the genomes of the fish species under study. It was expected that long PCR probes would be less specific. In fact, long PCR probes, such as pike probe 10E Lea (1600 bp), plaice probes 50G Lea (1600 bp) and 50G 1/2 (800 bp), flounder probes 31S Lea (1600 bp) and 31S 1/2 (800 bp), showed similar high intensity in all hybridization experiments. In contrast, five PCR probes amplified using turbot and flounder genomic DNA as templates (11SKB 1/2, 11SKB 1/3, 200S 1/2, 200S 1/3, 200S Lea) gave no hybridization signal for the majority of samples. The only exception was the plaice sample that hybridized strongly to the PCR probes but very weakly to the oligo-probes.
Oligonucleotide probes are generally considered to be more specific than long DNA probes. In this study, the highest specificity was obtained for salmon and rainbow trout probes (Oncm12_1, Sals10_1, Sals10_2) that evidently hybridized only with salmon, brown and sea trout DNA. Another example is the Dana0_1 probe revealing an extremely high signal (exceeding the limits of scanning parameters) when hybridized with Danio DNA. Two probes designed for pike transposons (Esol2425_1 and Esol2425_2) showed similar results. They produced a very strong signal after hybridization with the labelled genomic DNA of pike and a much weaker signal or no signal at all after hybridization with the samples from other fish species.
The largest number of probes spotted on the Tc1 microarray were designed on the basis of Danio transposon sequences. Six hybridizations with zebra fish genomic DNA (technical replicates of two specimens) demonstrated that, irrespective of the hybridization conditions, the highest, out-of-scale-signals were always detected for Dana0_1, the oligonucleotide probe specific for the Tc1 sequence of Danio albolineatus. Other Danio-specific probes gave strong signals for both Danio rerio specimens, but also for other tested fish species, except for carp and plaice. Similar hybridization results were obtained for probes designed for other Cyprinidae family members: Rasbora heteromorpha (Rash20_2, Rash20_1), Carassius auratus (Cara14_1, Cara14_2) and Acanthophtalmus kuhli (Acak13_1, Acak13_2). These results clearly show that Cyprinidae Tc1-like transposons are common in species that are not necessarily closely related.
Comparison of hybridization profiles of particular samples indicated that some fish species, e.g. plaice or pike, displayed unique hybridization profiles. Plaice DNA strongly hybridized only to plaice and flounder PCR probes, plaice and flounder oligo-probes and only one oligo-probe for Danio rerio (Danr3_1). Pike DNA bound specifically to two out of three oligo-probes designed for pike (Esol2425_1, Esol2425_2) and to the pike PCR probe (10E Lea). This probe also hybridized with samples from all the tested fish species, but the highest signal was observed for pike DNA. In contrast to the plaice sample, pike DNA hybridized also to other oligo-probes that were designed for Cyprynidae and Pleuronectidae but which also showed a high intensity signal for samples from other fish species.
The most distinctive family was Salmonidae, represented by four specimens: two salmon (Salmo salar) samples and two samples from sea trout (Salmo trutta morpha trutta) and brown trout (Salmo trutta morpha fario). DNA from Salmonidae hybridized strongly with the probes designed for Tc1-like elements originating from Salmonidae, but also with others (e.g. the probe designed for Tanichthys albonubes, Tana21_2).
Ambiguous results were obtained for turbot (Scophthalmus maximus). Turbot DNA samples, isolated from two specimens, showed very different hybridization profiles. Moreover, none of these samples hybridized with turbot PCR probes. Only one turbot sample (Scophthalmus maximus 1) bound with the three turbot-specific oligonucleotide probes. The hybridization profile of this sample resembled the profiles showed by the two zebra fish specimens.
The Tc1 microarray did not contain probes specific for round goby (Neogobius melanostomus), cod (Gadus morhua), perch (Perca fluviatalis), sea trout (Salmo trutta morpha trutta), brown trout (Salmo trutta morpha fario) or sprat (Sprattus sprattus). However, their genomic DNA hybridised to the microarray, especially to the probes that hybridized strongly with most genomic samples, e.g. Rash20_1, Rash20_2, Sals11_1, Plaf22_1, Plaf23_2, Plaf3031_2, Danr6_2, Danr5_1, Cara14_1 and Acak13_2.
In the reported experiments, at least three groups of probes could be distinguished: (1) probes with low or background-level signal intensity (this was most evident for the negative control probe pr10.2B_1, and probe Onct17_1); (2) probes that gave high and almost uniform signal intensity across samples (for example, Sals11_1, Danr6_2, Rash20_1, Rash20_2, Cara14_1); and (3) probes with different signal intensity for different samples. Only the probes in the last group were useful in differentiating the Tc1 profile between the genomes.
shows the results of k-mean clustering of probes with k=3 using Manhattan distance. The clustering was repeated 100 times; A presents the separation observed in about 20% of the clustering runs, while B presents the separation in the majority of the runs. The clustering in A corresponds to the general outline presented above: the first cluster contains six probes with very low hybridization intensity, while the second has 41 probes with high signal intensity across samples. The third cluster (21 probes) contains probes that efficiently and exclusively hybridized with the DNA of Salmonidae (seven probes at the top of the cluster). In B, clusters 1 and 2 contain the probes with high hybridization intensity across almost all samples (26 and 22 probes, respectively), while cluster 3 has both the low-intensity probes and the seven probes that efficiently hybridized with the DNA of Salmonidae (20 probes in total).
Figure 2. Results of probe grouping using the k-means algorithm with Manhattan distance and k=3. Panels A and B show the clustering observed in about 20% and 80% of clustering runs, respectively. The species names have been abbreviated to the first genus and species letter. See and text for details on data preparation and analysis.
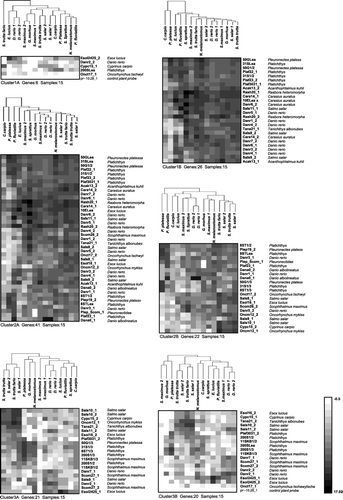
Separate hierarchical clustering of Tc1 profiles of each cluster clearly showed that the high-intensity probes (cluster 2 in A, clusters 1 and 2 in B) were responsible for the grouping of carp (Cyprinus carpio) and plaice (Pleuronectes platessa). Carp and plaice were the only two samples that had a hybridization signal noticeably lower for the high-intensity probe clusters which, in turn, were the only probes that had this grouping. This suggested that more informative hierarchical clustering could be obtained if high-intensity probes (and perhaps also low-intensity probes) were removed from the analysis.
The PCR probes proved to be less specific. Removing these probes did not affect the results of k-means clustering, with one exception: the probes Oncm12_1 and Sals9_1, previously on the very border of the second cluster in B and close to the probes in cluster 3 now moved together with these cluster 3 probes.
Following the observations on separate hierarchical clustering, two subsets were created of oligonucleotide probes: one containing 14 probes (cluster 3 from A without 7 PCR probes) and one containing 16 probes (cluster 3 from B without 6 PCR probes and with probes Oncm12_1 and Sals9_1). Hierarchical clustering using these two sets of probes () showed a more informative picture of the relationship between the Tc1 profiles. In particular, the spurious similarity between the carp and plaice profiles was removed, while the close relationships between the members of Salmonidae and between the Danio samples was preserved; the corresponding branches are significantly longer than zero (weighted least-squares likelihood ratio test at significance level 0.05). The profiles of two representatives of Perciformes, Perca fluviatilis and Neogobius melanostomus, moved together for the subset of probes that includes low intensity (B), but this grouping was not statistically significant.
Figure 3. Hierarchical clustering using Manhattan distance and average linking of fish Tc1 profiles using oligonucleotide probes in the cluster 3A in without 7 PCR probes (tree A) and using oligonucleotide probes in the cluster 3B in without 6 PCR probes and with probes Oncm12_1 and Sals9_1 (tree B). Statistically significant branches (weighted least squares likelihood ratio test p-value <0.05) are shown by thicker lines.
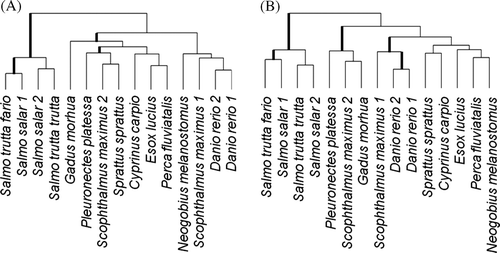
Discussion
This study is the first demonstration of microarray application for transposon identification in animal genomes. Oligonucleotide probes were constructed on the basis of known Tc1 sequences, which, in an earlier work, were characterized using PCR and sequencing (Pocwierz-Kotus et al. Citation2007). This earlier work showed that Tc1 transposase sequences could be almost identical within related fish, e.g. within Pleuronectiformes, and in some cases high nucleotide sequence similarity was observed in fish belonging to quite distantly related groups (round goby and perch). The results of hybridization presented here confirm these conclusions and demonstrate that microarray technology can be used in studies of transposon occurrence in fish genomes, and as a method of screening for transposable elements in phylogenetically related genomes.
However, unique Tc1-like transposon sequences can be present in some species. Such genome-specific transposons in pike (Pocwierz-Kotus et al. Citation2007) were observed. One of them lacked the 3′ terminal part of the transposase gene and apparently acquired its ITRs from a different subfamily of Tc1-like transposons through recombination. Such cases would be difficult to identify by PCR. Results presented here clearly show that sequences covered by two pike oligonucleotide probes (Esol2425_1, Esol2425_2) are highly specific for the pike genome. This example shows that hybridization can be a complementary method for detection of transposons that are not only mobile, but also potentially the most mutable elements of the genomes.
The results of the Tc1 microarray experiments suggest that Salmonidae genomes may carry transposons similar to those identified in Cypriniformes in addition to those previously found in Salmoniformes. This results in clustering of genomic DNA samples obtained from members of these two orders. The lowest signal intensities in this study were observed for the carp sample. This could potentially result from less efficient labelling, but it may reflect low representation of Tcl-like transposons in the carp genome. It coincides with the results of PCR screening showing that the Tc1-like transposons are widespread in various fish genomes, with the exception of halibut and carp (Pocwierz-Kotus et al. Citation2007).
In general, fish genomic DNA showed the strongest hybridization with the species-/family-specific probes, with two exceptions (carp and one turbot sample) for which hybridization to species-specific probes was not detectable. However, some oligonucleotide probes bound to DNA from different fish species. These probes, e.g. probes Rash20_1 and Rash20_2 (designed for harlequin rasbora), Cara14_1 (for crucian carp), Acak13_2 (for kuhli loach), Sals11_1 (for salmon) and Danr5_1 and Danr6_2 (for zebra fish), can serve as universal probes in future research, together with pike, flounder and plaice PCR probes. A high hybridization signal to these probes indicates that the transposon sequences they recognize occur in the genomes of distantly related species, perhaps resulting from horizontal transfer.
Hierarchical clustering of fish samples on the basis of Tc1 microarray hybridization data does not necessarily follow the taxonomic classification of fish species. For instance, both zebra fish samples always clustered together, but far from the carp, which belongs to the same family, Cyprinidae. Similarly, two turbot samples clustered separately from each other and from another pleuronectid species, plaice, although one of the samples (Scophthalmus maximus 2) showed a closer relationship to plaice when a subset of probes was used to obtain a dendrogram (B). However, only a small number of branches in the dendrograms obtained with these data had statistical support. A different Tc1 profile for the two turbot samples may suggest a recent or ongoing expansion of transposons, perhaps similar to those that are present in Danio. Round goby may present another interesting case. The sample showed high intensity of hybridization with the probes that gave high intensity in Danio and Scophthalmus maximus 1, which suggests that those transposons may have been recently active in Neogobius melanostomus genomes.
The post-hybridization division of microarray probes into clusters facilitates the distinguishing of probes with generally high or low intensity levels from the more complex pattern of probes reflecting evident and subtle differences between particular fish genomes. A comparison of the results obtained for oligonucleotide probe pairs designed on the basis of the same Tc1 sequence could be performed. Due to high sequence similarity in some cases, it was impossible to design two unique probes per sequence. Otherwise, each probe within a pair was designed to be complementary to another region of the same transposon sequence. Different regions can vary in their potential for secondary structure formation which affects their availability for hybridization. In addition, weak hybridization signals do not necessarily indicate low specificity or low quality of the probes, but can also result from changes (mutations, recombination) in the genome of the studied specimen, which reduces the complementarity of the genomic DNA to the particular probe. The results presented here can be useful in future research in which more probes, covering more Tc1-like transposase variants, should be included. However, this will not be possible without progress in fish gene/genome sequencing and mutation analysis.
A microarray based on the prototype presented here can serve not only as a tool for Tc1 transposon identification, but also for a rough estimation of transposon frequency in the genomes. Although microarrays generally are not reliable in a quantitative sense and it is not possible to derive transposon copy number from microarray data, the intensity level should reflect the frequency of the target sequence in the genome of investigated species (or more precisely, in the genome of a particular individual). This results from the mechanism of labelling. Transposons present in the genome in many copies will be labelled more efficiently than rare sequences – the frequency of rare transposon sequences can be underestimated when microarray data are used in frequency analysis.
In this work only fish genomes were analysed, but preliminary results show that DNA microarrays can be used in research on Tcl-like transposon families in eukaryotic genomes in general. This is a methodology that may help to explain the evolution of multiple Tc1 lineages in species for which full genome sequences will not be available for some time.
Editorial responsibility: Gavin Gouws
Acknowledgements
This study was funded by EC INCO grant ICA1-CT-2002-70022 and Polish Ministry of Science and Higher Education project N303 3870 33 and cGRASP to RW; the work of BW and JC was supported by projects N30403832/1819 and N303 291234.
Notes
Published in collaboration with the University of Bergen and the Institute of Marine Research, Norway, and the Marine Biological Laboratory, University of Copenhagen, Denmark
References
- Aharoni , A and Vorst , O. 2001 . DNA microarrays for functional plant genomics . Plant Molecular Biology , 48 : 99 – 118 .
- Almeidaa , LM , Silva , IT , Silva , WA Jr , Castroa , JP , Riggs , PK Cararetoa , CM . 2007 . The contribution of transposable elements to Bos taurus gene structure . Gene , 390 : 180 – 89 .
- Czarna , A , Sanjuan , R , Gonzalez-Candelas , F and Wróbel , B. 2006 . Topology testing of phylogenies using least squares methods . BMC Evolutionary Biology , 6 : 105
- Formanowicz , P , Handschuh , L , Urbaniak , R , Blazewicz , J and Figlerowicz , M. 2005 . Projektowanie mikromacierzy DNA . Na pograniczu biologii i chemii , 12 : 513 – 30 .
- Formanowicz , P , Urbaniak , R , Handschuh , L , Formanowicz , D and Figlerowicz , M. 2008 . Mikromacierze DNA – zasady projektowania sond . Biotechnologia , 4 : 54 – 67 .
- Garbarine E , Rosen G. 2008 . An information theoretic method of microarray probe design for genome classification Conference Proceedings, IEEE Engineering in Medicine and Biology Society , 3779 – 82
- Goodier , JL and Davidson , WS. 1994 . Tc1 transposon-like sequences are widely distributed in salmonids . Journal of Molecular Biology , 241 : 26 – 34 .
- Hammer , SE , Strehl , S and Hagemann , S. 2005 . Homologs of Drosophila P transposons were mobile in zebrafish but have been domesticated in a common ancestor of chicken and human . Molecular Biology and Evolution , 22 : 833 – 44 .
- Handschuh L , Zmienko A , Wloszczak W , Sikorski MM , Figlerowicz M. 2006 . Microarray hybridisation using Thermomixer comfort: The effect of different priming-strategies on signal intensity Eppendorf BioNews 25, Appl. Note 121, p. 3–4.
- Hyyrö , H , Juhola , M and Vihinen , M. 2005 . Genome wide selection of unique and valid oligonucleotides . Nucleic Acid Research , 33 ( 13 ) : 115
- Izsvak , Z , Ivics , Z and Hackett , PB. 1995 . Characterization of a Tc1-like transposable element in zebrafish (Danio rerio) . Molecular General Genetics , 247 : 312 – 22 .
- Ivics , Z , Izsvak , Z , Minter , A and Hackett , PB. 1996 . Identification of functional domains and evolution of Tc1-like transposable elements . Proceedings of the National Academy of Sciences of the USA , 93 : 5008 – 13 .
- Kane , MD , Jatkoe , TA , Stumpf , CR , Lu , J , Thomas , JD and Madore , SJ. 2000 . Assessment of the sensitivity and specificity of oligonucleotide (50mer) microarrays . Nucleic Acids Research , 28 : 4552 – 57 .
- Kulski , JK , Anzai , T and Inoko , H. 2005 . ERVK9, transposons and the evolution of MHC class I duplicons within the alpha-block of the human and chimpanzee . Cytogenetic and Genome Research , 110 : 181 – 92 .
- Kurland , CG. 2005 . What tangled web: Barriers to rampant horizontal gene transfer . Bioassays , 27 : 741 – 47 .
- Lam WL , Lee TS , Gilbert W. 1996 . Active transposition in zebrafish. Proceedings of the National Academy of Sciences of the USA 93:10 , 870 – 75
- Leaver , MJ. 2001 . A family of Tc1-like transposons from the genomes of fishes and frogs: evidence for horizontal transmission . Gene , 271 : 203 – 14 .
- Miller , WJ and Capy , P. 2006 . Applying mobile genetic elements for genome analysis and evolution . Molecular Biotechnology , 33 : 161 – 74 .
- Munoz-Lopez , M and Garcia-Perez , JL. 2010 . DNA transposons: Nature and applications in genomics . Current Genomics , 11 : 115 – 28 .
- Ogasawara , H , Obata , H , Hata , Y , Takahashi , S and Gomi , K. 2009 . Crawler, a novel Tc1/mariner-type transposable element in Aspergillus oryzae transposes under stress conditions . Fungal Genetics and Biology , 46 : 441 – 49 .
- Plasterk , RHA , Izsvak , Z and Ivics , Z. 1999 . Resident aliens the Tc1/mariner superfamily of transposable elements . Trends in Genetics , 15 : 326 – 32 .
- Pocwierz-Kotus , A , Burzynski , A and Wenne , R. 2007 . Family of Tc1-like elements from fish genomes and horizontal transfer . Gene , 390 : 243 – 51 .
- R Development Core Team 2008 . R: A language and environment for statistical computing R Foundation for Statistical Computing, Vienna, Austria Computer Program.
- Radice , AD , Bugaj , B , Fitch , DH and Emmons , SW. 1994 . Widespread occurrence of the Tc1 transposon family: Tc1-like transposons from teleost fish . Molecular General Genetics , 244 : 606 – 12 .
- Rouillard , J-M , Herbert , CJ and Zuker , M. 2002 . OligoArray: Genome-scale oligonucleotide design for microarrays . Bioinformatics , 18 : 486 – 87 .
- Sanjuan , R and Wróbel , B. 2005 . Weighted least-squares likelihood ratio test for branch testing in phylogenies reconstructed from distance measures . Systematic Biology , 54 : 218 – 29 .
- Venkatasubbarao , S. 2004 . Microarrays – Status and prospects . Trends in Biotechnology , 22 : 630 – 37 .
- Wang , H-Y , Malek , RL , Kwitek , AE , Greene , AS , Luu , TV Behbahani , B . 2003 . Assessing unmodified 70-mer oligonucleotide probe performance on glass-slide microarrays . Genome Biology , 4 : R5
- Wu , SH , Ramonell , K , Gollub , J and Somerville , S. 2001 . Plant gene expression profiling with DNA microarrays . Plant Physiology and Biochemistry , 39 : 917 – 26 .
- Zamudio , N and Bourc'his , D. 2010 . Transposable elements in the mammalian germline: A comfortable niche or a deadly trap? . Heredity , 105 : 92 – 104 .
- Zuker M , Mathews DH , Turner DH. 1999 . Algorithms and thermodynamics for RNA secondary structure prediction: A practical guide In Barciszewski J , Clark BFC RNA Biochemistry and Biotechnology, number 70, NATO Science Partnership Sub-Series: 3: High Technology. Chapter 2 Dordrecht : Kluwer Academic Publishers , p. 11 – 43