Abstract
This study describes the distribution, species composition and environmental characteristics of benthic habitats and biotopes from 38 stations in the Hardangerfjord, based on observations using high-definition video. Faunal composition was primarily related to substrate composition, distance from open ocean and depth (water masses) by using Detrended Correspondence Analysis. Six different biotopes were observed at depths between 100 and 400 m: (1) Lophelia reef, (2) sponge garden, (3) seapen communities, (4) soft bottom coral garden, (5) hard bottom coral garden and (6) cerianthid stands. Three Lophelia reefs were observed between 140 and 230 m depth in the outer part of the fjord. Geodia spp. and other large sponges were abundant on morainic bottom, on sills. Seapen communities occurred on muddy bottoms mainly between 250 and 350 m depth. The soft bottom coral garden comprised the gorgonian Isidella lofotensis, locally with densities up to 167 colonies/100 m2. Hard bottom coral gardens with Paragorgia arborea and Paramuricea placomus had a much lower density with a maximum of 33 colonies/100 m2. Litter and lost fishing gear were observed on 49% of the locations. Lophelia and sponges were covered with silt to a much higher degree than previously observed offshore. Whether this is caused by naturally higher particle flux in fjords or is due to an increased input from anthropogenic sources cannot be concluded from the present study. In general, anthropogenic activities have lead to increased particle flux in fjords and a demand for monitoring sensitive deep-water habitats to detect related trends of declining biodiversity.
Introduction
The Hardangerfjord is known to house a rich benthic fauna (Grieg Citation1914; Tambs-Lyche Citation1958; Brattegard Citation1966; Beyer Citation1968). The first studies of benthic fauna in the Hardangerfjord were carried out in the middle and late 1800s in the inner part of the main fjord outside Utne. Many Norwegian (e.g. P. Chr. Asbjørnsen, D.C. Danielssen, M. Sars, and G.O. Sars) as well as German and Swedish zoologists (J. Verkrüzen, P.O. Aurivillius and C. Bovallius) contributed to these studies (Grieg Citation1914). Grieg (Citation1914) extended the geographical range of these studies and presented a list of all mammals, fishes and invertebrates known from the middle and inner part of the Hardangerfjord based on these earlier studies. Many biotopes characterized by single megafaunal taxa have been identified as being vulnerable to pressures from human activities such as bottom fishing (OSPAR Commission Citation2008). It has been well documented how bottom trawling and longlining can damage Lophelia reefs and coral gardens (Fosså et al. Citation2002; Mortensen et al. Citation2005). However, the effect of pressures other than physical impact, such as increased concentrations of inorganic or organic particles, is poorly known for these biotopes.
The Hardangerfjord possesses a unique composition of benthic communities which have been poorly investigated while human activities, such as fish farming, are increasing. The moraine in the outer part of the fjord between Huglo and Hille represents the main sill with depths between 150 and 200 m (Holtedahl Citation1975) and is known to house a rich fauna with coral reefs (Lophelia pertusa (Linnaeus, 1758)) (Tambs-Lyche Citation1958), gorgonian coral and deep-sea species (Grieg Citation1914). The inner basin has depths exceeding 500 m where the deep-sea crinoid Conocrinus lofotensis (Sars, 1868) and the bamboo coral Isidella lofotensis Sars, 1868 occur. These species are viewed as having a high conservation value and are the main reason why outer parts of the fjord have been suggested as a conservation area. However, there have been very few studies during the last 50 years and baseline mapping is needed to know how these and other species with a high conservation value are distributed in the fjord.
This study is part of the Epigraph project, which aims to describe the relationships and connectivity between different ecosystems in the fjord. Description of benthic habitats and biotopes is therefore an important part of the project. The main aim of this study was to investigate the distribution, species composition and environmental characteristics for a wide array of benthic habitats and biotopes, including those characterized by corals and sponges. Two sub-tasks for the study were (1) to compare megafauna distribution documented in this study with information reported by Grieg (Citation1914), and (2) to document coral reefs in the inner part of the Hardangerfjord, observed by Grieg (Citation1914) and Tambs-Lyche (Citation1958), but without an exact geographic location. The results provide baseline information that is vital for selection of suitable locations for monitoring and protection.
Material and methods
The study area
The Hardangerfjord is 179 km long and is the third largest fjord in the world. It represents one of the major salmon farming regions in the world, with an annual production of approximately 70,000 t (Taranger et al. Citation2011). The head of the fjord is located inside the Eidfjord (). The inner part of the Hardangerfjord, the Sørfjord is often regarded as a side fjord to the Hardangerfjord. This is the longest of the Hardangerfjord's side branches, measuring 38 km in length.
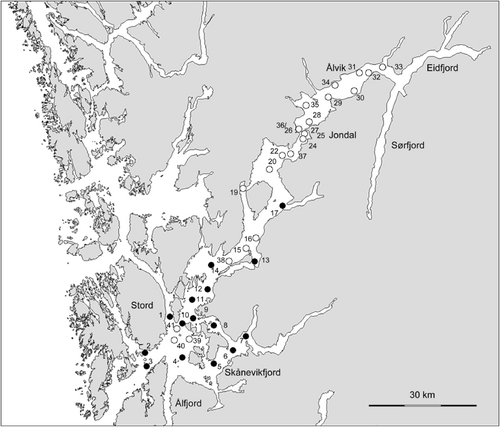
The geology and topography of the Hardangerfjord has been thoroughly described by Holtedahl (Citation1975). The fjord consists of three main basins separated by sills and bounded by steep walls on the sides. The area just outside the fjord represents the outer sill, with a depth of about 150 m, separating the fjord from the continental shelf. Inside the fjord a sill south of the Stord Island at 140 m depth () separates the outer fjord basin from the middle basin. The innermost sill is 190 m deep and situated south of the Huglo Island (east of st. 1). The outer and middle basins cover smaller areas than the larger inner basin. The middle basin represents a relatively wide area branching out into the sidefjords Ålfjord and Skåneviksfjord. The inner and deepest basin reaches about 890 m depth south of Ålvik (). It consists of three less-pronounced basins separated by sills deeper than 400 m. Most of the sediment accumulation in the fjord is fine material discharged by rivers, but slumps and turbidity currents have made a significant contribution since the last deglaciation (Holtedahl Citation1975). Holtedahl (Citation1975) estimated an average pelagic sedimentation rate of 5 mm per year. The sediments in the deep part of the basins vary between sandy clay and silty clay (Holtedahl Citation1975). Silt is the most common sediment fraction, contributing between 30 and 80%. The content of clay in the sediment increases with depth down to about 660 m, but shows a great variation. The content of sand varies greatly, but is highest shallower than 400 m.
Sampling stations
The location of the 38 investigated stations is shown in . Seven stations were selected based on historical information on the occurrence of Lophelia reefs provided by Tambs-Lyche (Citation1958) (sts. 1, 2, 3, 26, 27 and 36), and a local fisherman (st. 7). The remaining stations were selected semi-randomly from the inner to the outer parts of the fjord, covering depths from 50 to almost 500 m (maximum depth was given by the length of the cable to the video platform). The material consists of observations of megafauna (animals >5 cm) and substrate composition from two data sets: (1) real-time analysis of 46 video transects from all 38 stations, recorded during two cruises (2008 and 2009) with the RV Håkon Mosby (); (2) detailed quantitative analysis after these cruises of videos from 15 of the stations (1–14 and 17).
Table I. Station data for the surveyed locations, along with dominating bottom type, biotopes, and number of taxa observed in the field. Date format is dd.mm.yy. Positions are provided as decimal degrees.
Video recording
The seabed and its epifauna were documented with video recording using the video platform Campod. This is a tripod equipped with a high-definition colour video camera (Sony HDC-X300) for inspection purposes, and an analogue CCD video camera for navigation. It also has two parallel laser pointers (10 cm apart), for scaling of the imagery, and an altimeter to measure the height above the seabed.
Each video transect () was intended to be approximately 700 m long, but seven transects were shorter than this due to technical problems. The transects started and ended with the tripod standing on the seabed, enabling close-ups and visual scanning within an area of approximately 6 m2. Between start and end-points, Campod was towed behind the survey vessel at a speed of approximately 0.7 knots and controlled with the winch to provide a distance of around 1.5 m above the seabed. Geo-positioning of observations and of the track of the Campod was provided by a hydroacoustic positioning system (Simrad HIPAP and Eiva Navipac software) with a transponder mounted on the Campod. This system provided positions accurate to about 2% of the water depth. The length of the cable connecting the Campod to the winch was 500 m.
Real-time analysis of seabed observations
Real-time registration of observations of the seabed substrates and fauna were made along all video transects. Bottom types and organisms were identified and recorded in the field using the event-logging software ‘Campod Logger’ developed at the Institute of Marine Research. The bottom types were classified into 10 different categories (mud, sandy mud, sand, gravelly mud, gravelly sand, sandy gravel, gravel, boulders, bedrock and coral reef) modified from the Folk scale (Folk Citation1954).
Since registration of all occurrences of organisms is not practically possible in the field, the registration was carried out as described below. Navigational data from transponder and HIPAP (date, UTC time and positions) and depth were recorded automatically at 10-s intervals. Each transect was divided into five sequences: two locations (start and end of transect) with detailed inspection while Campod was parked on the seabed, and three consecutive sequences between 150 and 250 m long when Campod was towed between the start and end location. Each observed taxon was recorded only the first time that it occurred within each of the five sequences. Identified bottom type was recorded automatically at the same intervals until a change was observed. Then, the bottom type was manually changed to the new bottom type. This procedure provided a table of 204 samples (sequences) from the 46 video transects with positions, depth, bottom type and fauna. However, four of these did not contain any taxa.
Detailed quantitative analysis of video records
Thirteen video records obtained during the cruise in 2008 and two from 2009 were analysed in detail after the cruises using the software VideoNavigator (developed at the Institute of Marine Research). The analysis provided quantitative species data for 216 samples consisting of ~50 m long video sequences. Areas for the sequences were calculated based on distance travelled (from navigation data) and average field width. The field width was estimated from the ratio between measurements of the distance between two laser points on the video screen, and the width of the screen.
All organisms were identified to the lowest possible taxon and counted, or quantified as a percentage of seabed coverage following the method described by Mortensen & Buhl-Mortensen (Citation2005). Lebensspuren, burrows and encounters with lost fishing gear and litter were also counted. Abundance data for solitary organisms was standardized to number of individuals observed per 100 m2. In the detailed analysis the relative composition of ten bottom substrate classes (mud, sandy mud, sand, pebble, cobble, boulder, bedrock, coral rubble, dead Lophelia and live Lophelia) was estimated in addition to recording the occurrence of generic bottom types. The percentage cover of these classes was estimated subjectively at a scale of 5% intervals around six times per 50-m video sequence. The mean values of these were used for the sequences.
Detrended Correspondence Analysis (DCA)
To investigate similarities in fauna composition and to relate the identified fauna groups with controlling environmental factors, we applied Detrended Correspondence Analysis (DCA) (Hill & Gauch Citation1980) to three different data sets, using the software PC-Ord (McCune & Mefford Citation2006). DCA is an eigenanalysis ordination technique based on reciprocal averaging (Hill Citation1973). It can be considered an indirect gradient analysis, where environmental data are overlaid on the ordination plot. The basic approach is that DCA identifies groups of samples with similar species composition first and then assesses the correlation of the environmental variables in relation to these groups along the various axes in multidimensional space. The environmental variables included in the analysis were: percentage cover of the 10 bottom substrate classes mentioned above (only for the detailed analysis), depth (mean, maximum, and minimum), depth difference (as a measure of steepness), and distance to open ocean. In addition, latitude and longitude were included as an alternative indicator of distance to open ocean and/or other unaccounted variables with a geographic variation. Generic bottom types, defined according to the Folk scale, were used as a categorical variable for the two data sets from the real-time analyses. Only species found in more than four of the video sequences were included. This criterion left 88 taxa from the 46 video transects (700 m long), 86 taxa from the 196 video sequences (~200 m long) analysed in the field, and 78 taxa from the 216 video sequences (50 m long) from the 15 stations analysed quantitatively.
Results
General description of the seabed
The topography and composition of seabed substrates in the investigated parts of the fjord were highly variable (). Mud was the most common substrate with a presence in 43% of the 12,340 substrate records made in the field at 10-s intervals. Sandy mud and bedrock were equally common and were present in 17% of the records. Gravel occurred mainly on the two morainic sills (sts. 3 and 41) where it comprised 44% of all substrate records. Sand was not common and was only observed at st. 2, at around 260 m depth. Most sediment substrates occurred over a wide depth range, except for the morainic substrates associated with the sill at depths above 200 m, and sand (). Areas with boulders and gravel were most often associated with sloping bottom, but bedrock represented the steepest seabed with vertical walls more than 10 m high. Bedrock occurred along the sides of the fjord. At many locations an alternation between mud and bedrock was observed along the video transects. The mud was present on ledges and terraces on the rugged bedrock. The proportion of hard and soft sediments changed with the distance to the open ocean. This was most evident for mud, which increased inwards to the fjord. The correlation coefficient (r) for mud versus distance from the open ocean was 0.44 (p<0.005). The biotopes were more stratified with respect to depth with Lophelia reefs (140–233 m) and sponge gardens (105–325 m) as the two most shallow types (). Seapens had a wide depth distribution, but with a mean depth deeper than reefs and sponges. Hard and soft bottom coral gardens occurred below 300 m depth.
Taxa richness
In total (the two data sets combined) 195 taxa were observed, of which 94 were identified to species level. Fifty of the taxa were observed only once. The five most taxa-rich groups were: fish (44 taxa), sponges (31 taxa), cnidarians (26 taxa), crustaceans (22 taxa) and echinoderms (22 taxa) (Supplementary Table). Twelve of the sponge taxa did not represent an identifiable taxon but were described as different morphotypes. The crustacean taxa were dominated by decapods (13 taxa), and asteroids were the richest echinoderm group with 10 taxa. The five most common taxa recorded in the field occurred in more than 100 video sequences: Munida sp., Parastichopus tremulus (Gunnerus, 1767), Pandalidae indet., Psolus squamatus (O.F. Müller, 1776) and Phakellia ventilabrum (Linnaeus, 1767). Phakellia and Psolus occur on hard bottom (mainly bedrock), while the rest prevail on softer sediments. Based on the counting of individuals and colonies from the 15 video transects that were analysed quantitatively, 6 taxa were found to dominate in terms of abundance: Munida sp. (5646 ind.), axinellid sponges (2172 col.), cerianthid anemones (1436 ind.), Parastichopus tremulus (1057 ind.), Psolus squamatus (938 ind.; C) and Virgularia mirabilis (Müller, 1776) (882 col.). The brisingid ophiuroid Brisinga endecacnemos Asbjørnsen, 1856 (H) and the mushroom coral Anthomastus grandiflorus Verrill, 1878 were observed seven and eight times, respectively. These are deep-sea species rarely observed on the continental shelf. In this study Anthomastus was observed at three stations (22, 26, and 27) (), restricted to an area south of Jondal at depths between 339 and 418 m. Brisinga was observed at six stations (22, 27, 31, 32, 33, and 38) between 278 and 430 m in the inner third of the study area.
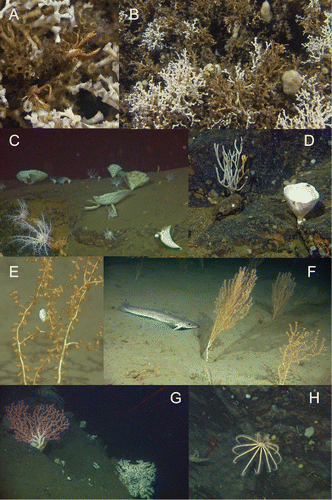
Biotopes
Six biotopes characterized by certain sessile megafaunal taxa were identified at depths between 100 and 400 m: (1) Lophelia reef, (2) sponge garden, (3) seapen and burrowing megafauna, (4) soft bottom coral garden, (5) hard bottom coral garden and (6) cerianthid stands ( and ). The five first of these are regarded as ‘threatened and/or declining habitats’ by OSPAR (OSPAR Commission Citation2008). Biotopes 4 and 5 belong to the ‘Coral garden’ habitat defined by OSPAR (Christensen Citation2010). However, we follow a recent definition introduced by the Norwegian red list of habitats (Kålås et al. Citation2006). The habitat termed cerianthid stands (named after the dominating anemone family Cerianthidae) is thought to support fish abundance (Fuller et al. Citation2008), and is included in this study because cerianthids appeared as a distinct habitat in some of the investigated areas.
Lophelia reefs. Living Lophelia pertusa (A,B) were observed at three stations (1–3) in the outer part of the fjord, and skeletons of dead Lophelia were observed at three additional stations (7, 13 and 37). With one exception (st. 13), the seabed at these stations consisted of coral skeletons over several metres along the seabed. At st. 13, only one block of Lophelia pertusa was observed; thus, this location cannot be termed a reef without further mapping in the nearby area. The reefs were located on sloping bottom with surrounding substrates alternating between gravelly sand and exposed bedrock. Live colonies of Lophelia occurred at depths between 145 and 232 m. Dead Lophelia had a wider depth distribution, from 81 to 242 m. At st. 2, close to land, live Lophelia occurred only as one small part of a single colony. In the area near Jondal (sts. 26, 27 and 36) attempts were made to locate a Lophelia reef based on positions interpreted from the map in the publication by Tambs-Lyche (Citation1958). No reefs were observed on this location.
Sponge gardens
Geodia spp. and other large Demospongia occurred with highest abundance on morainic hard bottoms associated with sills. The best example of this was found at sts. 10 and 41, where a mixture of Geodia spp., Aplysilla sulfurea Schulze, 1878, Phakellia sp. and Axinella infundibuliformis (Linnaeus, 1759) (C,D) together with unidentified sponges were observed in high densities. The highest local density of sponge colonies was 416 colonies/100 m2, and the mean density where they occurred was 45 colonies/100 m2.
Seapen fields
Five species of seapens were observed (Halipteris finmarchica (Sars, 1851), Funiculina quadrangularis (Pallas, 1766), Kophobelemnon stelliferum (Müller, 1776), Pennatula phosphorea Linnaeus, 1758 and Virgularia mirabilis). The maximum density locally was 133 colonies per 100 m2, with an average where they occurred of 17.5 colonies/100 m2. Funiculina, Kophobelemnon and Virgularia often occurred together and in areas dominated by Isidella lofotensis. Kophobelemnon contributed to most of the abundance of seapens. Norway lobster (Nephrops norvegicus (Linnaeus, 1758)) and squat lobster (Munida sp.) occurred on muddy bottoms, often together with seapens.
Coral gardens
Five species of gorgonians were observed (Isidella lofotensis, Clavularia borealis Koren & Danielsen, 1883, Paragorgia arborea (Linnaeus, 1758), Paramuricea placomus (Linnaeus, 1758) and Swiftia pallida Madsen, 1970). Except for Isidella lofotensis, these were observed on rocky bottom (boulder and bedrock) and dead coral, and are here termed ‘hard bottom coral garden’ (Figures 3–5). The maximum density of colonies was 33 per 100 m2. Where they occurred, the mean density of colonies was 15.4 per 100 m2. Paragorgia and Paramuricea were observed at all three live Lophelia reefs, but not on the two dead ones. Paragorgia was observed together with Paramuricea on five stations, and alone on four, whereas Paramuricea occurred alone on two stations (40 and 7). Isolated colonies of Swiftia pallida were observed at stations 1 and 10 and did not contribute significantly to the density of gorgonians. Clavularia borealis was observed only once, on the Lophelia reef at st. 3 at 141 m depth.
Isidella lofotensis (E,F) constituted the coral garden type we have termed ‘soft bottom coral garden’ and was recorded 33 times in the field from 10 locations with sandy muddy bottom, at depths between 243 and 349 m. The quantitative analysis revealed a maximum local density of 167 colonies/100 m2, but on average when present it had a density of 24.7 colonies/100 m2. Shrimps and amphipods were often observed on the branches.
Cerianthid stands
Unidentified cerianthids were most abundant at stations 12, 24 and 27_9, at depths between 346 and 414 m. These locations were all characterized by mud and sandy mud. The most typical taxa that co-occurred with cerianthids were pandalid shrimps, Parastichopus tremulus (Holothuroidea), Rhabdammina sp. (Foraminifera) and Stylocordyla borealis (Loven, 1868) (Demospongia).
Classification of locations
DCA was performed on the three data sets (presence/absence data from whole transects, from 200 m sequences, and results from video analysis with abundance data; Tables II and III and Figures –). Three gradients were observed in the faunal composition, related to substrate composition, distance from the open ocean and depth (water masses). The analysis of aggregated data for whole video transects () revealed five groups related to geographic location, substrate composition and depth ().
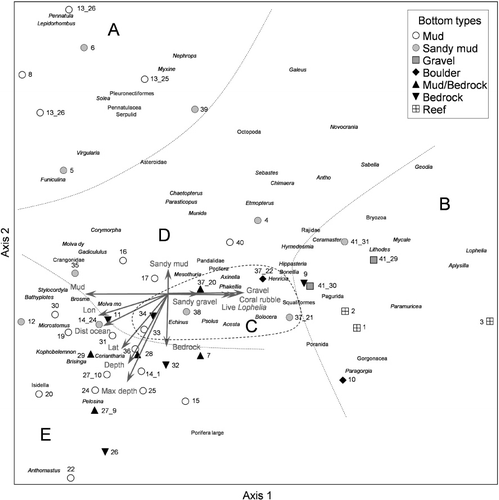
Table II. Correlation (Pearson and Kendall) between environmental variables and ordination axes from the DCA analysis of field observations based on semi-quantitative abundance data for 46 whole video transects and presence /absence data for 196 video sequences.
Group A: seven transects from six stations in the outer, easterly part of the fjord. These locations were characterized by soft substrates (mud and sandy mud) at depths between 69 and 355 m. Except for one location (st. 39) these were all located in side-fjords behind islands (). Typical taxa for these locations were seapens (Pennatula, Virgularia and Funiculina), flatfishes and Norway lobster ().
Group B: this group is also located in the outer fjord, but with coarser sediments than in group A. These eight transects from six stations were characterized as sponge gardens, hard bottom coral gardens, and Lophelia reefs during the field survey. Characteristic taxa were Lophelia, Aplysilla sulfurea, Geodia spp., Mycale lingua (Bowerbank, 1866), Paragorgia and Paramuricea.
Group C: this group consisted of five transects from three stations, characterized by mainly hard substrates (boulder and bedrock with mud), with exceptions for st. 36 with gravelly sandy mud and st. 37 with sandy mud and occurrence of dead Lophelia. All locations were shallower than 250 m. Typical taxa for this group were Psolus squamatus, axinellid sponges, Bolocera tuediae (Johnston, 1832) (Actiniaria), Acesta excavata (Fabricius, 1779) (Bivalvia) and Echinus sp. (Echinoidae).
Group D: six transects from six stations. These locations were characterized by soft sediments (mud and sandy mud) at depths shallower than 250 m. The characteristic taxa in this group were Munida sp. (Decapoda), Parastichopus and Corymorpha nutans M. Sars, 1835 (Hydrozoa).
Group E: this is a large group of 18 transects from 16 stations. They represent various sediments at depths deeper than 250 m. It was not possible to identify subgroups representing the different substrates in this analysis. Typical taxa for this group were Isidella, Kophobelemnon, Ceriantharia, Bathyplotes natans (M. Sars, 1868) (Holothuroidea), Brisinga and Anthomastus.
gives an overview of correlation coefficients between environmental variables and the ordination axes for the DCA plots of analyses based on field data ( and ). The correlation coefficients between environmental variables and the ordination axes for the abundance data analysis is presented separately in because in this analysis the substrate was analysed based on sediment grain size classes rather than generic bottom types (Folk scale). The strongest correlations found for the analysis of whole transects was the maximum depth of transects, with an r of −0.61. Among the sediment types the frequency of occurrence of mud and gravel along the video transects ranked highest, strongly correlated with the first axis. Of other factors, longitude and distance to the open ocean were most strongly correlated, both with axis one. For the video sequences analysed in the field (), the variables were more weakly correlated with the ordination axes (). Minimum depth was the strongest variable, with a correlation coefficient of 0.43. The sediment type that was best correlated was mud (r=0.35). Distance to ocean and longitude were equally well correlated with axis one.
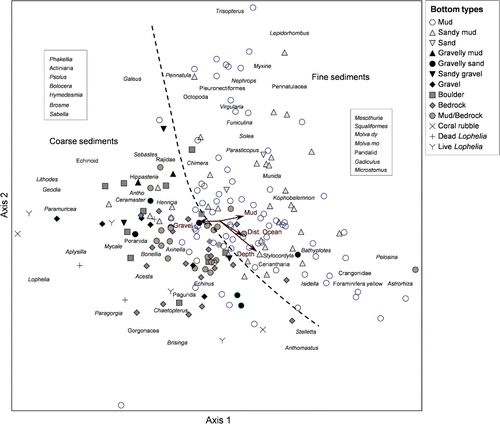
Table III. Correlation (Pearson and Kendall) between environmental variables and ordination axes from the DCA analysis of 216 video sequences (50 m long) from 15 stations.
shows the ordination plot for the DCA of the quantitatively analysed small (50 m) video sequences from 15 stations. In this analysis, the bottom types grouped quite clearly. The three variables that were best correlated with the axes were percentage cover of sandy mud, distance to open ocean and minimum depth within video sequences. Locations characterized by sandy mud could be divided into different depth groups.
Indications of human impacts
Broken colonies of Lophelia pertusa were observed on all three live reefs, but the damage was not extensive. One broken colony of Pargagorgia arborea was observed on bedrock. This damage was most likely caused by fishing with longline and gillnet. Tracks on the seabed were observed, but it is unclear whether these were caused by trawling or anchors. Litter and lost fishing gear were observed on 49% of the locations. The most common object was metal wire (). Wires may be lost during bottom trawling on rough bottom, but may also be deliberately thrown overboard when they have been worn out. Sightings of other man-made objects such as two bicycles, one ladder and one car tyre, in addition to other unidentified litter, strongly indicate that the fjord is still used as a private dumping site for various wastes. The lost fishing gear spotted comprised rope, longline and traps for Norway lobster. The highest amount of litter was found in the vicinity of the reef at st. 2. Patches of silt on Lophelia pertusa and different sponges were observed to a much higher degree than what has been found in studies offshore, regardless of the degree of fishing impact.
Table IV. Number of litter items observed at different stations.
Discussion
Methods
This study focuses on characterization of benthic habitats in the Hardangerfjord at depths below diving and on all types of seabed, including those that cannot be sampled with grabs. There are no earlier studies presenting quantitative data on the distribution of hard and mixed bottoms from this or other Norwegian fjords, making comparisons with other studies impossible. The review of the fauna of the Hardangerfjord by Grieg (Citation1914) is the best available source regarding the distribution of deep-water megafauna. However, this information is mainly based on collections made with triangular dredge and bycatch on long-line, only enabling qualitative comparisons. In this study we have provided the first data on abundance of megafauna that can be used as a baseline for future studies of temporal changes. This is particularly relevant for the long-lived taxa such as gorgonian corals, seapens, sponges and Lophelia pertusa. The gorgonians and seapens were quantified as numbers of colonies per 100 m2 and Lophelia pertusa as percentage cover. Both types of estimates were made within short sequences, mainly shorter than 5 m, and later aggregated into 50 m sequences. The quantitative data on sponges, however, are more complicated to use because they represent both percentage cover and number of colonies, depending on taxa and density (e.g. in places where various unidentifiable sponges occurred in high densities, they were quantified as percentage cover, whereas where they were more scattered, they were counted). Despite these difficulties in obtaining quantitative assessments for sponges, their relative abundance and local distribution may serve as coarse background data for assessing sponge abundances in future surveys. Unfortunately, many sponges could not be identified to any taxon below class, because species identification largely relies on spicule morphology. The number of morphotypes as an indicator of sponge diversity cannot be directly compared with other studies based on sampled specimens. Different species may have similar appearance, or one species may occur as different morphotypes. Our results on morphotypes, however, indicate that there is a higher species diversity of sponges than we were able to document in this study.
Biotopes
Two different types of coral garden (soft bottom and hard bottom coral gardens) were observed in the Hardangerfjord. Paragorgia aborea and Paramuricea placomus locally formed relatively dense stands on hard bottom (boulder and bedrock) in the outer part of the fjord, whereas Isidella lofotensis was more widespread and occurred in high densities on deep sandy mud. The latter species is relatively rare outside the deep open fjords in Norway (Kålås et al. Citation2006), while it is known to be relatively common in some Norwegian fjords. It is notable that the gorgonian Primnoa resedaeformis was not observed during this investigation. Grieg (Citation1914) also noted the absence of this species from the fjord. This may indicate a difference in environmental demands between Paragorgia and Primnoa, even though these species commonly co-occur elsewhere along the Norwegian coast. According to Grieg (Citation1914), Paragorgia and Paramuricea were smaller and less common in the inner part of the fjord compared to the outer and more exposed part of the fjord. This corresponds to what we observed. Our observed distribution of Isidella, however, differs from what Grieg (Citation1914) reports. According to Grieg (Citation1914), it only occurs in the outer part of the fjord, while we observed Isidella at nine stations from the middle part and up to 100 km into the fjord from the open ocean (st. 26).
There is no suggested threshold density for terming an area ‘coral garden’, and the observed densities of colonies vary between species, depending on colony size and environmental conditions (Mortensen & Buhl-Mortensen Citation2004, Citation2005; Mortensen et al. Citation2005, Citation2006; Christiansen Citation2010). With densities of up to 167 colonies/100 m2, Isidella clearly forms coral garden on deep soft bottom in the Hardangerfjord. The observations of shrimps and amphipods on Isidella indicate a rich associated fauna, as described for other gorgonians (Buhl-Mortensen & Mortensen Citation2004, Citation2005).
The morphology of the Lophelia reefs observed in this study differs from those described from the Norwegian shelf (Mortensen et al. Citation1995). An offshore reef is typically mound-shaped with a summit of live coral, surrounded by dead coral blocks and coral rubble near the ‘foot’ of the reef. The reefs in the Hardangerfjord correspond better to the term ‘coral hills’ as described by Dons (Citation1944). Such coral hills have been observed in several fjords during visual surveys carried out by the Norwegian mapping project MAREANO (www.mareano.no, unpublished results). The coral hills do not display the same zonation patterns as the mound-shaped reefs on the shelf. The living corals may occur patchily at different depths, separated by coral rubble and blocks of dead Lophelia. This may be caused by the varied topography of the bottom and/or more complex current patterns as a result of the local seabed topography. Most likely there are many more locations of Lophelia reef not yet discovered in the Hardangerfjord. It is known from earlier publications that there are coral reefs as far inside the fjord as Jondal (Grieg Citation1914; Tambs-Lyche Citation1958). However, we did not find reefs at these locations. These occurrences are only indicated with relatively coarse geographical positions, making further searching and mapping necessary. Without detailed bathymetry (i.e. from mapping with multibeam echosounder) discoveries of such reefs are mainly by chance.
Human impacts
The broken colonies of Pargagorgia arborea and Lophelia pertusa are most likely caused by fishing with longline and gillnet. Patches of silt were observed on Lophelia and different sponges to a much higher degree than what has been observed during comparable studies offshore. Whether this is a natural condition for the fjord or caused by organic input from industries (agriculture, sewage and fish farming) cannot be concluded based on these observations. It is important to establish monitoring of these sensitive deep-water habitats within fjords, where they are particularly exposed to impacts related to human activity, to detect changes in abundance and habitat quality which may be linked to increased impact from aquaculture and climate change. Regarding Lophelia pertusa as an indicator of environmental changes, the percentage cover of live tissue (polyps and coenchyme) at fixed locations may be the best parameter. Because of the difficulty of repeating exactly the same video transect over time, data aggregated over sequences of video will capture a high degree of natural variation and would require a set of replicates to capture a reliable mean value for the area.
Conclusions
This study confirms much of the general distribution patterns of megafauna as reported by Grieg (Citation1914), with the exception of Isidella lofotensis, which turns out to be more widespread than observed based on previous dredge sampling. The video survey indicates that organisms viewed as rare (based on sampling) frequently occur, but only with few individuals and/or in habitats inaccessible to traditional sampling gear (i.e. dredges and grabs) such as cliff overhangs. These species that are normally rare (occurring with patchy distribution of several kms) will disappear unnoticed using traditional sampling methods, and they may prove to be particularly sensitive to human impact. Compared with information on the occurrence of the species documented by Grieg (Citation1914, and references cited therein), the findings in this study indicate that the status of the deep water biotopes characterized by sessile megafauna is good in the Hardangerfjord, with an uncertainty related to the observation of high cover of detritus on Lophelia and sponges.
Editorial responsibility: John Zardus
smar_a_810759_sm1889.pdf
Download PDF (215 KB)Acknowledgement
This project was funded by the Ministry of Fisheries and Coastal Affairs and the Research Council of Norway (Ecological Processes and Impacts Governing the Resilience and Alternations in the Porsangerfjord and the Hardangerfjord, EPIGRAPH, project no. 188955/130).
References
- Beyer F. 1968. Zooplankton, zoobenthos, and bottom sediments as related to pollution and water exchange in the Oslofjord. Helgoländer Wissenschaftliche Meeresuntersuchungen 17:496–509. 10.1007/BF01611250
- Brattegard T. 1966. The natural history of the Hardangerfjord. 7. Horizontal distribution of the fauna of rocky shores. Sarsia 22:1–54.
- Buhl-Mortensen L, Mortensen PB. 2004. Symbiosis in deep-water corals. Symbiosis 37:33–61.
- Buhl-Mortensen L, Mortensen PB. 2005. Distribution and diversity of species associated with deep-sea gorgonian corals off Atlantic Canada. In: Freiwald A, Roberts JM, editors. Cold-water Corals and Ecosystems. Berlin: Springer, p 849–79.
- Christiansen S. 2010. Background Document for Coral Gardens. Publication Number: 486/2010. London: OSPAR. 39 pages.
- Dons C. 1944. Norges korallrev. Det Kongelige Norske Videnskabers Selskabs Forhandlinger 16:37–82. ( in Norwegian)
- Folk RL. 1954. The distinction between grain size and mineral composition in sedimentary rock nomenclature. Journal of Geology 62:344–59. 10.1086/626171
- Fosså JH, Mortensen PB, Furevik DM. 2002. The deep-water coral Lophelia pertusa in Norwegian waters: Distribution and fishery impacts. Hydrobiologia 471:1–12. 10.1023/A:1016504430684
- Fuller SD, Murillo Perez FJ, Wareham V, Kenchington E. 2008. Vulnerable Marine Ecosystems Dominated by Deep-Water Corals and Sponges in the NAFO Convention Area. Serial No. N5524. NAFO Scientific Council Research Document 08/22. Dartmouth, Nova Scotia: NAFO. 24 pages.
- Grieg JA. 1914. Bidrag til kundskapen om Hardangerfjordens fauna. Bergens Museums Aarbok 1913(1): 1–147. ( in Norwegian)
- Hill MO. 1973. Reciprocal averaging: An eigenvector method of ordination. Journal of Ecology 61:237–49. 10.2307/2258931
- Hill MO, Gauch HG. 1980. Detrended Correspondence Aanalysis: An improved ordination technique. Vegetatio 42:47–58. 10.1007/BF00048870
- Holtedahl H. 1975. The geology of the Hardangerfjord, West Norway. Norges geologiske undersøkelse 323:1–87.
- Kålås JA, Viken Å, Bakken T. 2006. 2006 Norwegian Red List. Trondheim, Norway: Skipnes AS. 416 pages.
- McCune B, Mefford MJ. 2006. PC-ORD: Multivariate analysis of ecological data. MJM Software, Gleneden Beach, Oregon. Computer Software.
- Mortensen PB, Buhl-Mortensen, L. 2004. Distribution of deep-water gorgonian corals in relation to benthic habitat features in the Northeast Channel (Atlantic Canada). Marine Biology 144:1223–38. 10.1007/s00227-003-1280-8
- Mortensen PB, Buhl-Mortensen L. 2005. Coral habitats in The Gully, a submarine canyon off Atlantic Canada. In: Freiwald A, Roberts JM, editors. Cold-water Corals and Ecosystems. Berlin: Springer, p 247–77.
- Mortensen PB, Hovland M, Brattegard T, Farestveit R. 1995. Deep water bioherms of the scleractinian coral Lophelia pertusa (L.) at 64°N on the Norwegian shelf: Structure and associated megafauna. Sarsia 80:145–58.
- Mortensen PB, Buhl-Mortensen L, Gordon Jr. DC, Fader GBJ, McKeown DL, Fenton DG. 2005. Effects of fisheries on deep-water gorgonian corals in the Northeast Channel, Nova Scotia (Canada). American Fisheries Society Symposium 41:369–82.
- Mortensen PB, Buhl-Mortensen L, Gordon Jr. DC. 2006. Distribution of deep-water corals in Atlantic Canada. In: Proceedings of the 10th International Coral Reef Symposium. Okinawa, Japan, June 28–July 2 2004. Tokyo: Japanese Coral Reef Society, p 1832–48.
- OSPAR Commission. 2008. OSPAR List of Threatened and/or Declining Species and Habitats. Reference number 2008-6. London: OSPAR. 4 pages.
- Tambs-Lyche H. 1958. Zoogeographical and faunistic studies on West Norwegian marine animals. Årbok for Universitetet i Bergen, Matematisk-naturvitenskapelig serie 7:3–24.
- Taranger GL, Svåsand T, Madhun AS, Boxaspen KK 2011. Risikovurdering – miljøvirkninger av norsk fiskeoppdrett. Fisken og Havet, Særnummer 3 – 2011. 99 pages. (in Norwegian)