Fjords are unique coastal landscape structures that require enhanced basic and applied biological research (Brattegard et al. Citation2011). In Norway, efforts have recently been made to study selected fjord systems more effectively and integratively. The current Thematic Issue presents results focusing on the Hardangerfjord in western Norway.
The Hardangerfjord is approximately 180 km long and 2–10 km wide and is the second largest fjord in Norway. It was formed by glacial activity, has two moraine sills of around 150 m depth in the outer parts and extends to a maximum depth of 830 m in the fjord basin. In the inner part the main fjord splits into several narrow side fjords, the longest being Sørfjord which is 40 km long. Several large rivers run into the fjord and in the side fjords the surface water is permanently brackish. The rich populations of fish and shellfish have provided food and work since the times of the first recorded settlement, about 8000 years ago.
The Hardangerfjord region provides good opportunities for industrial activities based on both the tributary freshwater systems and the main fjord. Consequently, the fjord basin may be affected by a number of local anthropogenic activities, such as agriculture, aquaculture, fisheries, hydroelectric power production, pollution from households and industry, and also through more indirect mechanisms such as climate change.
Following the beginning of hydroelectric power plant constructions, the production of carbide and later dicyanamide was begun in the innermost part of the Sørfjord in 1908; later, other industrial plants began production in the fjord. The industrial activity resulted in strong pollution by heavy metals in the inner parts of the Hardangerfjord (Ruus et al. Citation2012). The discharges of black lime waste resulted in extremely low oxygen concentrations, high nitrate levels and toxic flagellate blooms in the late 1990s (Aure & Pettersen Citation1998). Piles of black lime waste still remain in the fjord, but the ecosystems are in recovery (Ruus et al. Citation2006). The massive hydroelectric power production in many rivers has provided much public revenue for local communities. The production clearly affects the habitats and life of Atlantic salmon and brown trout, while less is known about its effect on the fjord ecosystem. The Hardangerfjord is at present one of the regions in Norway with the highest density of salmon farms, with a production that exceeded 80,000 tonnes in 2011 (Knut Johnsen, The Norwegian Directorate of Fisheries, pers. comm.) or about 10,000 times the production of wild Atlantic salmon in the fjord.
With high human activity in the coastal zone, the fast expansion of salmon farming along the Norwegian coast and particularly in the Hardangerfjord, the reports of massive salmon lice infection on anadromous brown trout (Salmo trutta), disease problems in many salmon farms and numerous of escapees in salmon rivers, there has been a strong concern among the public and management authorities for the wild salmon, the anadromous brown trout and the environment of the Hardangerfjord.
Coincidentally, there are reports of excessive growth of filamentous macroalgae in the middle part of the fjord. The interdisciplinary research programme ‘Ecological Processes and Impacts Governing the Resilience and Alternations in the Porsangerfjord and the Hardangerfjord – EPIGRAPH’ was therefore initiated by the Ministry of Fisheries and Coastal Affairs in 2008 to learn more about the interactions between human activity and the ecosystems of the fjords. To distinguish between changes caused by local salmon farming and more general, large-scale trends related to climatic changes, the project was designed as an interdisciplinary project with eight work packages: (1) Physical oceanography and its importance for fjord ecology; (2) Nutrients, fluorescence and benthos; (3) Temporal changes in macroalgal vegetation in the intertidal and shallow subtidal zone; (4) Valuable and sensitive habitats; (5) Zooplankton and sprat diet; (6) Fish resources and predation; (7) Population structure and genetic relationship between fjord and open water components of sprat; and (8) Interactions between salmon farming and wild anadromous salmonids. The project was then linked up to other supplementary projects conducted at the Institute of Marine Research, Bergen, such as monitoring of salmon lice on wild and farmed salmonids, oceanography, ongoing cruises with the research vessels FF Håkon Mosby and FF Brattstrøm, genetic impact on wild salmon from escapees and the running activity at the IMR field station in the Hardangerfjord. The investigations conducted 50 years earlier in the Hardangerfjord, published in a series of papers under a common heading ‘The natural history of the Hardangerfjord’ (see also Brattegard et al. Citation2011), provided a very useful baseline for some of these studies.
Complex topography divides the fjord into several branches and these may constitute local ‘systems’ where the hydrography, advection and zooplankton communities differ from the main fjord (Falkenhaug & Dalpadado Citation2014). The topography is also fundamental for the current patterns. The longitudinal currents almost follow ‘roads’ in the fjord and small eddies are formed in the wider part of the fjord (Asplin et al. Citation2014). Oceanographic variability on small spatial and temporal scales is documented. Tidal currents with unidirectional flow for only 4–5 h are not important as transport mechanism in the Hardangerfjord. Neither is the estuarine ‘counter-current’ typically described from shallow estuaries. This counter-current is weak in the relatively deep Hardangerfjord. The most important mechanism for transport of water masses in the Hardangerfjord is the current from internal forces typically generated in the coastal water outside the fjord. These processes create relatively long-lasting and deep currents in and out the fjord (Asplin et al. Citation2014). The main fjord exchanges water at approximately monthly intervals in the upper 50–100 m. This exchange of water is important for the resilience of the pelagic ecosystem in a long fjord with relatively large inputs of nutrients from fish farming and agriculture. This exchange of water also has implications for short-lived organisms in the pelagic system.
Sprat is an important consumer of zooplankton in the Hardangerfjord system and a conveyer of energy from zooplankton to higher trophic levels, e.g. sea trout. The diet of the Hardangerfjord sprat was diverse, but small harpacticoid copepods were important especially in the late season when larger copepods migrated to deeper water (Falkenhaug & Dalpadado Citation2014). Despite a strong increase in zooplankton biomass towards the outer parts of the fjord, higher abundance of sprat was recorded in the inner fjord branches, indicating that sprat distribution may be affected by other factors than food availability alone. During periods of lower availability of zooplankton, phytoplankton was recorded in the sprat diet. This is the first time herbivorous filter-feeding has been documented in sprat (Falkenhaug & Dalpadado Citation2014).
In the Oslofjord, conspicuous changes in macroalgal communities have been documented over time, with a loss of fucoids in the inner parts of the fjord concurrent with an increase of nutrient exposure (Bokn & Lein Citation1978). The recent investigation into the macroalgal communities in the Hardangerfjord showed a higher species richness compared with the 1950s (Husa et al. Citation2014a). Changes were most pronounced towards the inner parts of the fjord, with an increase in species richness of red algae, a phenomenon most likely caused by altered salinity due to hydroelectrical power production. Since the early 1990s there has been a pronounced increase in sea-surface temperature (SST) in the North Atlantic. A higher frequency of species with warm-water affinity was found compared to the observations made in the 1950s, indicating a possible effect of climate change (Husa et al. Citation2014a). The present and unaltered distribution of some dominating taxonomic groups (fucoids, kelp) showed that these groups are relatively resilient to recent impacts such as rise in SST and the current levels of nutrient inputs to the fjord from fish farming. Dense establishments of filamentous algae were recorded at many sites, in particular in the central part of the fjord. This phenomenon may be caused by local nutrient enrichment from fish farms in the area, climatic variations or by the massive urchin grazing on ephemeral seaweeds (kelp) providing space for opportunistic species (Husa et al. Citation2014a). Apart from the proximity of the fish farms, no clear biological effects of nutrient release from the salmon farming could be found. Nutrient levels and chlorophyll-a levels in the main fjord, measured during a three year period, showed no indications of elevated concentrations due to anthropogenic emissions (Husa et al. Citation2014b).
The deep water habitats of the Hardangerfjord were also studied during EPIGRAPH. Oxygen levels and benthic soft bottom infauna communities in deep water basins (500–800 m depth) in the fjord were in good environmental condition, except from a high abundance of opportunistic species in the outermost basin, which might be slightly impacted by the high salmon production (Husa et al. Citation2014b). Four types of sensitive habitats were additionally observed at depths between 100 and 300 m. These included cold-water coral reefs, sponge communities, sea pen and burrowing megafauna and soft-bottom coral gardens (Buhl-Mortensen & Buhl-Mortensen Citation2014; ). Signs of human impact were clearly evident as broken colonies of corals. Patches of silt were observed on coral reefs and sponge communities to a much higher degree than in comparable studies in the open ocean. Although this can be a result of natural processes, the impact from organic loading from fish farms cannot be excluded.
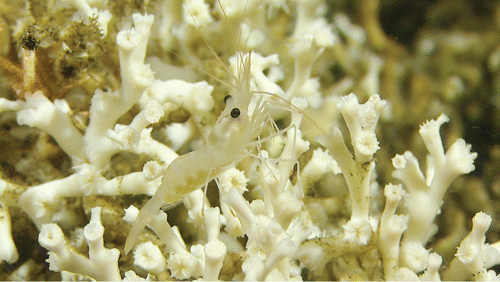
Also, the present demersal fish community was compared to findings in the 1950s using similar capture methods. The demersal fish community demonstrated resilience against environmental changes and the species composition has not varied much since 1950s. The species recorded in the 1950s were found during the present study, but the abundance of large rajids had decreased, possibly an effect of by-catches in commercial fisheries. Some new species were recorded compared to the 1950s. These were species with warm-water affinity, and close to their northern distribution limits (Bjørge Citation2012).
A pronounced change in human impact on the Hardangerfjord ecosystem since the 1950s is the rapid and comprehensive growth of the fish farming industry for salmonids during the recent decades. This development may have severe impacts on wild populations of sea trout and Atlantic salmon. Historically, the rivers of the Hardangerfjord had abundant populations of salmon and sea trout. These populations have declined significantly during recent decades and the current status gives reasons for concern. Vollset et al. (Citation2014) demonstrated a correlation between the location of rivers and the survival of salmon. The further a river was located from the open coast, the lower was the salmon survival. This is probably a fjord effect and not related to mortality during migration in the open ocean. Sea trout, which do not migrate out of the fjord, did not show similar spatial patterns in survival.
Infection of salmon lice is a problem in fish farms. Farmed fish can be chemically or biologically treated to reduce the problem. However, the salmon lice from fish farms are also infectious to wild salmon and sea trout. Skaala et al. (Citation2014a) caught all descending sea trout smolt in Guddalselva and tagged them. One group of smolt was treated chemically against salmon lice, and the control group was not. When catching ascending trout, Skaala et al. (Citation2014a) demonstrated that the treated trout had a twice as high and a significantly different survival rate compared to the control group. This is compelling evidence for salmon lice-induced mortality of sea trout populations in the Hardangerfjord.
It is the free-ranging, pelagic third copepodid stage of salmon lice that is infectious to salmon and sea trout. This stage has a life span of about three weeks, but is temperature-dependent. Using current patterns to simulate the dispersal of third-stage salmon lice from a source, Asplin et al. (Citation2014) showed that the highest concentrations of salmon lice released to the main fjord remained close (a few kilometres) to the source, but a smaller number of the lice were able to disperse widely.
What are the lessons we have learned from EPIGRAPH in the Hardangerfjord? First, the project has generated new information on ecological processes in the Hardangerfjord and on the relative importance of various impact factors of salmon farming on the ecosystem. Second, a time-series obtained through systematic, interdisciplinary monitoring of key components of the ecosystem and of impact factors is essential in regions with extensive human activity. These are important prerequisites for a successful, sustainable management of coastal waters and marine resources. As an example, the lack of a scientific, nationally coordinated monitoring programme for escaped farmed fish, with predictable funding, is a serious problem for management of fish farming as well as of wild salmon.
We have seen that components of the ecosystem have demonstrated resilience against environmental changes since the 1950s. The demersal fish community documented in the 1950s remains largely intact and a few warm-water species have colonized the fjord. The dominating macroalgal groups are also intact compared to the 1950s and the species richness has increased due to the occurrence of species with a warm-water affinity and a higher number of red algae penetrating into the inner parts of the fjord. However, important and iconic fauna elements such as Atlantic salmon and sea trout have not demonstrated resilience to recent changes in the Hardangerfjord area. The rapid expansion of fish farming has most probably had detrimental impacts on the wild populations of salmonids. To counteract this development, conservation and management action plans (e.g. Skaala et al. Citation2014b) have to be implemented. As an important first step, the dispersal of salmon lice from fish farms has to be curtailed.
To further monitor the development of the ecosystem in the Hardangerfjord, it is important that key elements of the EPIGRAPH programme are continued or repeated at intervals. To improve our understanding of the causal relationships behind the changes, it is important to conduct comparative studies in similar fjord systems where human impacts (e.g. from fish farming) are less pronounced or preferably do not exist. Human impacts in the fjord and coastal ecosystems are likely to intensify. Continued and expanded studies of coastal ecology are critical for precautionary management advice to ensure sustainable use of the coastal zone.
The investigations conducted 50 years earlier in the Hardangerfjord provided an important baseline for the present study of impacts, and likewise we hope that the results from EPIGRAPH (2008–2011) will represent a baseline for continued monitoring of the environment and future studies in the Hardangerfjord. We also believe that the knowledge will be more easily available for management authorities, scientists, for educational purposes and for the general public when it is presented as a bundled Thematic Issue than if the papers were published individually in separate journals.
Øystein Skaala, Kjersti Sjøtun, Einar Dahl,
Vivian Husa, Arne Bjørge
Guest Editors
Franz Uiblein
Editor
References
- Asplin L, Johnsen IA, Sandvik AD, Albretsen J, Sundfjord V, Aure J, et al. 2014. Dispersion of salmon lice in the Hardangerfjord. Marine Biology Research 10:216–225.
- Aure J, Pettersen R. 1998. Miljøundersøkelser i norske fjorder: Sørfjorden Hardanger. Fisken og Havet 11/1998. 15 pages. ( in Norwegian)
- Bjørge A. 2012. Sluttrapport til Forskingsrådet for programmet Ecological Processes and Impacts Governing the Resilience and Alternations in the Porsangerfjord and the Hardangerfjord (EPIGRAPH), Prosjektnr. 188955. Bergen: Havforskningsinstituttet. 8 pages. ( in Norwegian)
- Bokn T, Lein TE. 1978. Long-term changes in fucoid association of the inner Oslofjord, Norway. Norwegian Journal of Botany 25:9–14.
- Brattegard T, Høisæter T, Sjøtun, K, Fenchel T, Uiblein, F. 2011. Norwegian fjords: From natural history to ecosystem ecology and beyond. Marine Biology Research 7:421–24. 10.1080/17451000.2011.561355
- Buhl-Mortensen P, Buhl-Mortensen L. 2014. Diverse and vulnerable deep-water biotopes in the Hardangerfjord. Marine Biology Research 10:253–267.
- Falkenhaug T, Dalpadado P. 2014. Diet composition and food selectivity of sprat (Sprattus sprattus) in Hardangerfjord, Norway. Marine Biology Research 10:203–215.
- Husa V, Steen H, Sjøtun K. 2014a. Historical changes in macroalgal communities in Hardangerfjord (Norway). Marine Biology Research 10:226–240.
- Husa V, Kutti T, Ervik, A, Sjøtun K, Kupka Hansen P, Aure J. 2014b. Regional impact from fin-fish farming in an intensive production area (Hardangerfjord, Norway). Marine Biology Research 10:241–252.
- Ruus A, Molvær J, Schøyen M. 2006. Overvåkning av miljøforholdene i Sørfjorden i 2005. Oksygen, nitrogen og fosfor i vannmassene. SFT rapport 951/06. Oslo: Norsk Institutt for Vannforskning. 27 pages. ( in Norwegian)
- Ruus A, Kvassnes AJS, Skei J, Green N, Schøyen M. 2012. Monitoring of environmental quality in the Sørfjord 2011. Metals in the water masses, contaminants in organisms. NIVA-rapport 6399-2012. Oslo: Norsk Institutt for Vannforskning. 95 pages.
- Skaala Ø, Kålås S, Borgstrøm R. 2014a. Evidence of salmon lice-induced mortality of anadromous brown trout (Salmo trutta) in the Hardangerfjord, Norway. Marine Biology Research 10:279–288.
- Skaala Ø, Johnsen GH, Lo H, Borgstrøm R, Wennevik V, Hansen MM, et al. 2014b. A conservation plan for Atlantic salmon (Salmo salar) and anadromous brown trout (S. trutta) in a region with intensive industrial use. Marine Biology Research 10:308–322.
- Vollset KW, Skoglund H, Barlaup BT, Pulg U, Gabrielsen S-E, Wiers T, et al. 2014. Can river location within a fjord explain the density of Atlantic salmon and sea trout? Marine Biology Research 10:268–278.