ABSTRACT
Global warming is causing changes in the food web structure and seasonal plankton dynamics. The Baltic Sea is one of the fastest-warming sea areas and warming consequently affects the timing and magnitude of phytoplankton blooms. Based on available Chlorophyll a data from nine years between 1979 and 2018, from the entrance to the Gulf of Finland, we studied the timing of the phytoplankton spring bloom in relation to spring seawater temperature. We found the peak of the bloom to occur earlier in years with higher spring seawater temperature. In warmer years, there was also a shorter time lag between phytoplankton and zooplankton biomass peaks. In addition, it seems as the spring bloom total biomass has decreased with time, with more extensive summer blooms during later years, in line with the general trend observed in the Baltic Sea. The spring bloom has traditionally been considered the most important part of the season, but we argue that the whole growth season should be investigated since summer blooms appear to increase with warming.
Introduction
In temperate areas, the onset of the phytoplankton spring bloom is determined by irradiance (Sommer and Lengfellner Citation2008) and is therefore affected by water column stability and in polar and high-latitude areas to variable extent also by sea ice (Sommer et al. Citation2012). Seasonal dynamics are further affected by nutrient availability and loss factors such as grazing by zooplankton, sinking and mortality (Sommer et al. Citation1986).
Ocean temperature is increasing globally, and the Baltic Sea is one of the fastest-warming sea areas (Belkin Citation2009) with a projected change of 2–3°C before the end of the century (Meier Citation2015). In addition, a prevailing highly positive North Atlantic Oscillation Index (NAO) over the Baltic Sea results in warmer and more humid winters with less sea ice and warmer seawater (BACC II Author Team Citation2015). Warming is causing changes to the pelagic food web both directly by regulating light-saturated growth rates of primary producers and indirectly through stratification of the water column, affecting nutrient availability (Winder and Sommer Citation2012; Spilling et al. Citation2018). The number of days with sea surface temperature above 17°C almost doubled between 1983 and 2014 and between 1998 and 2013 both light attenuation and near-surface Chlorophyll a (Chl a) increased in the central Baltic Sea (Kahru et al. Citation2016). Earlier, more prolonged spring blooms with lower average biomass during the past 20 years have been observed (Raateoja et al. Citation2005; Groetsch et al. Citation2016; Kahru et al. Citation2016; Hjerne et al. Citation2019; Wasmund et al. Citation2019).
Concurrent to the observed temperature increase, eutrophication has progressed in the Baltic Sea and is projected to accelerate since climate warming will increase freshwater and nutrient runoff from land (Meier et al. Citation2011). The spring phytoplankton community composition has shifted towards dominance of dinoflagellates over diatoms at least in eastern parts of the Baltic Sea (Klais et al. Citation2013). These drivers of phytoplankton production contribute to the magnitude and composition of organic matter available to pelagic grazers and export to the seafloor, with potentially strong impact on coastal food webs in areas characterized by strong pelagic-benthic interactions such as the Baltic Sea (Griffiths et al. Citation2017; Rodil et al. Citation2020).
A change in the timing of the spring bloom may lead to trophic decoupling between phytoplankton and zooplankton (Edwards and Richardson Citation2004). This was observed in a large temperate lake where Daphnia resting eggs were unaffected by temperature while the spring bloom occurred earlier (Winder and Schindler Citation2004). In Arctic areas, the match or mismatch between phytoplankton and copepods largely depend on the response of phytoplankton to environmental conditions (ice retreat, mixing depth) since the abundance of Calanus copepods largely is determined by the ascent of overwintering adults (e.g. Hansen et al. Citation2003). However, warming also affects zooplankton life cycle events, leading to changes in phenology, abundance and community structure at least in some systems (Richardson Citation2008). A strong association between mild winters (strong NAO) and high zooplankton biomasses in spring and early summer has been observed (Viitasalo et al. Citation1995; Hansson et al. Citation2010). Higher temperature in spring allows for earlier hatching of copepod eggs from the sediments and shorter development times (Feike et al. Citation2007), leading to a potential tighter coupling between phytoplankton and zooplankton grazers.
Evidence of climate-induced changes on plankton dynamics are accumulating, especially for the western and southern Baltic Sea, but corresponding analyses are lacking from the eastern Baltic Sea coastal areas. In the northern Baltic Sea, seawater temperature has increased, and ice cover has diminished during the past 85 years (Merkouriadi and Leppäranta Citation2014). The effects of rapid warming in recent years has not been addressed in this area, and re-evaluation of bloom timing and intensity under the current environmental conditions is needed for an improved understanding of marine ecosystem responses to climate change. We, therefore, studied the timing of the spring bloom in relation to spring seawater temperature in a coastal area of the eastern Baltic Sea, at the entrance to the Gulf of Finland. We used available data from years with sufficient and comparable sampling frequency on phytoplankton and zooplankton. We hypothesize that higher seawater temperature in spring in combination with less extensive sea ice cover leads to an earlier phytoplankton spring bloom peak. We further expect a tighter match between phytoplankton and zooplankton in years with higher spring seawater temperature.
Methods
The study area is situated east of the Hanko peninsula, at the Southwestern coast of Finland in the Baltic Sea. The area has a variable morphology, with a narrow depression leading from the open sea to the main sampling station Storfjärden (59° 51,3′N, 23° 15,9′E). The area has stagnant deep-water during summer and no tides or permanent halocline. Storfjärden receives inflow of fresh water from the River Svartån and periodically inflows of cold and saline water from the open Gulf of Finland. Maximum depth at Storfjärden sampling station is 34 m and at Storgadden 40 m (59°47,40′N, 23° 15,8′E), and the mean salinity is 6. Winter sea ice cover may last up to 4 months, with high inter-annual variability. While coastal ice typically forms annually in sheltered bays and the inner archipelago, years with no sea ice at the main sampling station occur regularly, reflecting the more exposed conditions of the open Gulf of Finland.
The spring bloom in the Northern Baltic Sea is dominated by cold-water diatoms and dinoflagellates. Under-ice blooms of a haptophyte (Hällfors and Niemi Citation1974) and dinoflagellates (Niemi and Åström Citation1987; Spilling Citation2007) have been observed but are not a regularly occurring phenomenon. Cyanobacteria commonly occur during late summer (July, August), in some years forming extensive surface blooms. The most common genera are Aphanizomenon, Nodularia, and Anabaena.
For analysing the timing of the spring bloom in relation to temperature, data available in the literature from the main station Storfjärden was assembled, covering the years 1979, 1983, 1984, 1985, 1992, 2000. These were combined with new and previously unpublished data from 2015, 2016 and 2018 (). Chl a was used as a proxy for phytoplankton biomass to determine the date when the peak of the phytoplankton spring bloom was observed, except for two years when no chlorophyll data was available and the timing of the bloom was determined from published data on primary production instead (). Sampling depths varied slightly between years, yet all observations cover the upper mixed layer and did not vary within the same year, which is most important for determining the peak of the spring bloom. One water sample was collected from each depth. These were filtered in triplicate samples through GF/F filters and Chlorophyll a was extracted using either methanol (in 1983 and 1984) or ethanol (1985–2018) as a solvent and measured with a spectrofluorometer or spectrophotometer. The methods did not vary within a year and are comparable. For analysing the magnitude and distribution of Chl a at station Storfjärden between spring and summer seasons, sufficient data were available from the years 1983, 1984, 1992, 2016 and 2018.
Table I. Description of data on phytoplankton used in this study with information on collection year, sampling station, methods, references and the time of ice retreat.
For analysing the time lag between phytoplankton and zooplankton peaks, we included observations from the Tvärminne area, Storfjärden (station XII) and Storgadden (station P3) from years when both phytoplankton and zooplankton data were available from the same station. During spring, mesozooplankton in the sampling area is comprised of the copepods Acartia sp., Eurytemora affinis and Temora longicornis (Koski et al. Citation1999). The peak of zooplankton occurrence was determined as the date of observed maximum biomass or abundance reported in the original data depending on which parameter was reported in individual years (). Data on biomass or abundance of mesozooplankton were obtained from publications for the years 1979, 1980, 1983, 1984, 1988, 1992 and combined with own, unpublished data from 2016 (). Most studies were based on weekly measurements (one net haul per occasion), with the exception of 1979 when biweekly measurements were applied and in 1983 when the sampling frequency was scarcer in early spring. Seawater temperature has been measured at the main station three times per month from April to December since 1926 (Merkouriadi and Leppäranta Citation2014). From 1970 to 2012 temperature was measured at discrete depths (Finnish Meteorological Institute). These data were supplemented by CTD data from 2013–2018 (Tvärminne Zoological Station). Measurements earlier than April are not as frequent since the main sampling station may be covered by ice. We used temperature measurements from April (beginning, middle and end of month at 0.5, 5, and 10 m depth) to calculate averages for the years covered by the biological parameters. The peak of the spring bloom usually occurs in April, after the ice break-up. Mean water temperature in April was therefore considered to adequately describe the water column conditions at the time of the spring bloom.
Table II. Description of data on zooplankton used in this study with information on collection year, sampling station, methods and references.
We used linear regression to analyse the effect of spring temperature on the timing of the spring bloom peak, as well as the lag time (days) between the peak of Chl a and zooplankton. Phytoplankton biomass in spring and summer, respectively, was estimated by time integration of Chl a for the five years with available and comparable data from spring and summer using the function Area under the curve in Graph Pad Prism 8. The threshold for bloom conditions was set to 3 µg Chl a l−1 as previously applied by Kahru et al. 2016 (i.e. not including summertime minimum in calculations). This threshold effectively separated the spring bloom from the summer bloom in all years. The spring bloom was defined as the period from March onwards when Chl a uninterruptedly exceeded the threshold. The summer bloom included multiple peaks above the threshold until Chl a finally did not exceed 3 ug l−1, typically in October.
Results
Average seawater temperature (0–10 m) in April increased between 1970 and 2018. Although years with average April temperature around 2°C also occurred early in the period, the frequency of warm years increased after 1990 (). Information on the timing of sea ice cover in this area is restricted to the years for which there are plankton observation (). Based on these observations, years with sea ice cover lasting until April were common until the late 1980s. Since the early 1990syears with no sea ice cover at the main station or ice cover of very short duration (breakup in January) have been common.
Figure 1. Temperature and time (day of year) of the spring bloom peak at Tvärminne Storfjärden. Temperature is the average seawater temperature between 0 and 10 m in April (temperature for 1997 is missing).
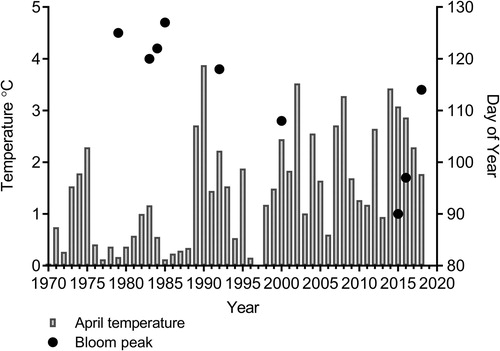
Chl a revealed a seasonal pattern with highest concentrations typically occurring in April followed by a sharp decline (). In most years Chl a increased again in June albeit to lower levels than in spring. While the summer bloom terminated in September in 1983, concentrations around 5 µg Chl a l−1 were observed as late as October in more recent years.
Figure 2. Annual time series of Chlorophyll a in the Tvärminne region, Southwestern Finland, based on data retrieved from publications listed in .
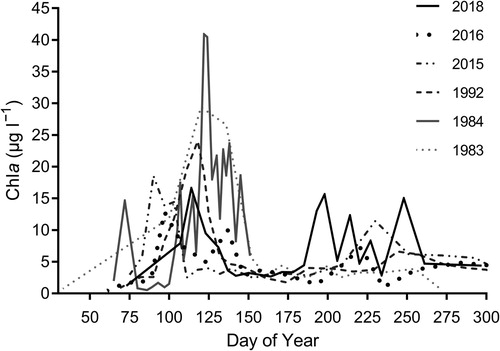
The peak of the spring bloom occurred earlier in years with warmer sea temperatures. We found a significant negative relationship between average seawater temperature in April and the time (day of year) of the spring bloom peak, in the upper mixed layer (t = −5.603, R2 = 0.79, P = .001), based on observations from years with available seasonal data from Tvärminne Storfjärden (1979, 1983, 1984, 1985, 1992, 2000, 2015, 2016 and 2018, ). Further, the bloom peak Chl a concentration was lower during more recent years (2016 and 2018), than in the 1980s. The peak concentration was 29.6 and 40.9 µg Chl a l−1 in 1983 and 1984 respectively, whereas below 20 µg Chl l−1 in 2016 and 2018. The spring in 2018 was cold whereas the summer was exceptionally warm with high (above 20°C) sea surface temperatures throughout the summer. The spring bloom Chl a concentration maximum was 16.8 µg Chl a l−1. Cyanobacteria blooms were observed at Storfjärden in July, and August and highest surface Chl a value for the whole season, 39.3 µg Chl a l−1 was observed on September 5th.
In 1983 there was no summer bloom (no values above 3 µg l−1), in 1992 the summer bloom corresponded to 27%, whereas in 2016, 63% of the total Chl a. Of all the years included in this study, 2018 showed exceptionally strong summer blooms that extended into the autumn, comprising as much as 65% of total Chl a (between April and October) ().
Figure 3. Time integrated biomass for spring and summer blooms in the Tvärminne area. Bloom conditions were defined as Chl a concentration exceeding 3 μg l−1. The spring bloom was defined as the period starting in March when Chl a uninterruptedly exceeded the threshold, i.e. one peak. The summer bloom included multiple peaks between June and October after which Chl a did not exceed the threshold. The biomasses of these peaks were pooled into one summer bloom. In 1983 Chl a did not exceed the threshold after the spring bloom. (* indicates missing summer data).
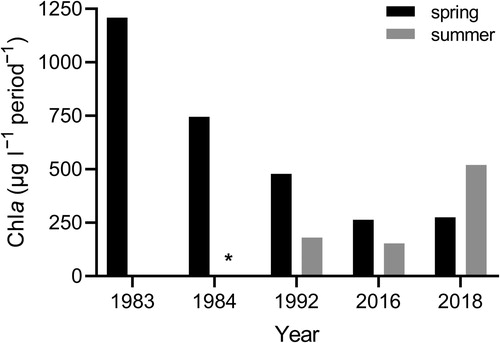
Using data from stations Storfjärden and Storgadden, we evaluated the coupling of phytoplankton and their consumers depending on water temperature. Although data from two stations were used in this analysis, phytoplankton and zooplankton were sampled at the same station in individual years. There was shorter lag (days) between the peak of the spring bloom Chl a concentration and the zooplankton biomass peak with warmer sea temperature (t = −3.047, R2 = 0.58, P = .03, ). The longest lag was in 1984 (40 days) whereas there was no lag in 2016.
Discussion
In the Baltic Sea, the phytoplankton spring bloom is affected by physical and hydrological factors such as light conditions and water stability. Seawater temperature affects the stratification of the water column as well as the metabolism of planktonic organisms. Ice cover lowers the light conditions, and although the bloom can start below the ice (Spilling Citation2007; Apollonio and Matrai Citation2011), the peak mostly occurs soon after ice breakup in the Baltic Sea. The warmer spring seawater temperature and consequently earlier peak of the spring phytoplankton bloom showed in this study, is probably at least partly associated with less sea ice or earlier ice break-up, indirectly affecting the light conditions. Ice coverage (Lehmann et al. Citation2011), the length of the ice season and maximum ice thickness have decreased during the past century. In our study area, the seawater temperature has increased on average by 1°C in 85 years (Merkouriadi and Leppäranta Citation2014). In 1979 and 1983–1985 the ice broke up in mid or late April whereas in 1992 and 2000 there was no sea ice and in 2016 the ice broke up already in January.
In the southwestern Baltic Sea, the spring bloom has advanced by 1.4 d yr−1 and the growing season increased by 127 days between 1988 and 2017 (Wasmund et al. Citation2019). The earlier start of the spring bloom was related to increased sunshine duration and the prolongation in autumn due to warmer seawater temperature. In the western Baltic Sea, off the Swedish coast, the advancement of the spring bloom was also likely caused by more sunshine and less windy conditions (Hjerne et al. Citation2019), rather than sea temperature (Hjerne et al. Citation2019). However, in the Gulf of Finland, the temperature is strongly connected to the prevailing climate forcing (Almén et al. Citation2017) which may increase the inter-annual variability and make temporal trends in plankton dynamics difficult to detect.
From the presented Chl a record from the western Gulf of Finland, it seems as the spring bloom total biomass has decreased with time in the Tvärminne area (), with more extensive summer blooms during later years. Although the available data is restricted for this area, it is in line with the general trend observed in the Baltic Sea (HELCOM Citation2013). Satellite observations suggest that Chl a maximum has shifted from spring to summer (Kahru et al. Citation2016). Although clouds and particulate organic matter can disturb the satellite measurements, there are already data from in situ measurements supporting these observed changes. Chl a levels in spring increased in the western Gulf of Finland between 1970s and the 1980s but decreased in the 1990s (Raateoja et al. Citation2005). Similarly, high spring bloom average biomass was observed between 1977 and 1989, while spring biomass was lower than the summer biomass after 1989, outside Askö, on the Swedish East coast (Hjerne et al. Citation2019). Surprisingly, Raateoja et al. (Citation2018) did not find a relationship between the intensity of the spring bloom and the wintertime DIN pool or any significant temporal trends related to nutrients in the western Gulf of Finland. Nutrients are available in plenty after winter, and the intensity of the bloom is probably more dependent on hydrography and water stability, whereas nutrients are more important for determining intensity and development of the summer bloom.
Lower phytoplankton peak biomass and lower mean biomass during the spring period can be explained by both warming and higher zooplankton densities due to a closer match in time and through faster growth rates of zooplankton (Sommer and Lengfellner Citation2008; Sommer and Lewandowska Citation2011; Velthuis et al. Citation2017). Temperature also affects zooplankton life cycle events towards earlier hatching from the sediments and shorter development times. We found a tighter match between the peak of the phytoplankton and zooplankton biomass peaks, which is in line with the experimental results of Aberle et al. (Citation2012) showing a shorter time lag between phyto- and microzooplankton related to temperature during spring. However, looking only at the peak date of Chl a and the date at which copepod biomass or abundance peaks does not fully reveal how well the two groups match, in terms of resource availability, as it does not consider the overlap of biomass over a longer period, or the actual grazing pressure. Grazing increases with warmer temperatures during the season, meaning that the peak in zooplankton biomass occurs earlier than the zooplankton-grazing peak. High biomass of grazers could conceivably reduce the maximum Chl a concentration during the course of the spring bloom (Sommer and Lewandowska Citation2011). Higher temperature has also been found to increase parasitic infections in phytoplankton (Frenken et al. Citation2016). Parasitism on phytoplankton has not been investigated in this area, but Elovaara et al. (Citation2020) observed that living cells accounted for ca 20–100% of the total phytoplankton community throughout the year, with higher proportion of living cells in spring (65%) than in summer (48%). These findings are comparable to observations during spring in the open Baltic Proper (Vanharanta et al. Citation2020).
Zooplankton is known to strongly regulate the export of organic matter in more oceanic systems (e.g. Wassmann Citation1998; Wexels Riser et al. Citation2002). Variable stocks of zooplankton in winter and temperature-related hatching of eggs may suggest variable top-down control of the spring bloom also in the Baltic Sea (Katajisto et al. Citation1998; Möllmann et al. Citation2000). Previous studies have emphasized phytoplankton community composition for regulating vertical export of particulate organic matter in the Baltic Sea (Heiskanen et al. Citation1998; Tamelander and Heiskanen Citation2004). A shift from diatom to dinoflagellate dominated spring blooms, caused by changes in winter harshness (Klais et al. Citation2013), could decrease sedimentation and increase nutrient recycling (Tamelander et al. Citation2017; Spilling et al. Citation2018). These studies together suggest that while phytoplankton community affects the composition of organic matter export, zooplankton may, to a variable extent impact the magnitude of particulate organic carbon export. The magnitude of the vertical flux of organic matter is highly dependent on the coupling between pelagic primary and secondary producers (Wassmann Citation1998). Furthermore, pelagic trophic interactions are strong drivers of the composition of the downward organic matter flux (Wexels Riser et al. Citation2002; Tamelander et al. Citation2012). Warming causes increased respiration (Lewandowska and Sommer Citation2010); however, more for zooplankton than for phytoplankton, further enhancing the effect of heterotrophic processes (Maar and Hansen Citation2011).
Organic matter derived from phytoplankton are important for benthic communities below the euphotic zone that strongly contribute to remineralization of carbon and nutrients in shallow coastal areas. In the Baltic Sea, the coupling between the pelagic and benthic food webs tend to be strong (Kopp et al. Citation2015; Griffiths et al. Citation2017; Rodil et al. Citation2020). Changes in community composition and timing of phytoplankton production may affect the food supply and food quality for benthic communities (Tamelander and Heiskanen Citation2004; Spilling and Lindström Citation2008). The spring bloom, mainly consisting of diatoms is crucial for benthic communities, whereas the toxic cyanobacteria N. spumigena do not improve food conditions for soft bottom fauna in the Baltic Sea (Nascimento et al. Citation2009). Another aspect of the changes in the composition and timing of phytoplankton is the effect on geochemical cycling of nutrients and carbon chemistry (Spilling et al. Citation2018). Decomposition of organic matter produced during summer and autumn that has settled to the seafloor consumes oxygen and releases carbon dioxide, leading to decreased pH, i.e. acidification of bottom waters (Sunda and Cai Citation2012). Hypoxic conditions during winter and high load of organic material and nutrients will then likely affect spring conditions and next year's phytoplankton production, which is usually not considered in models. The study site has not been affected by hypoxia due to regular wintertime mixing of the entire water column in near shore waters (Gammal et al. Citation2017). In deeper parts of the open Baltic Sea the permanent halocline at ca 70 m depth effectively reduces deep mixing, making deep-water hypoxia widespread (Carstensen et al. Citation2014). Deeper, sub-halocline areas are therefore likely to be particularly vulnerable to excessive organic matter loading of the sediment.
We acknowledge that only a limited number of years with comparable data were available for this synthesis. Frequency and resolution of the data vary between publications making more advanced or detailed analyses difficult. Models are increasingly used in management efforts to determine the impact of environmental change and human activities on ecosystem dynamics and are useful in a scientific context for evaluating the quantitative importance of ecosystem drivers. However, observations of key parameters from the system under study are required to validate such results, and to further improve model parameterization.
Conclusions
Our study of the timing of the phytoplankton spring bloom in a coastal area in the eastern Baltic Sea indicates similar trends as generally found for the northern parts of the Baltic Sea. Warmer sea temperature in spring advances the timing of the spring bloom. However, we also observed a shorter lag between phyto- and zooplankton in warm years. Although warming is causing a shorter lag between primary and secondary producers that could lead to less POC being sedimented to the seafloor, the changes in phytoplankton dynamics may counteract the effects when looking at the whole season as warming is causing a shift in primary production and phytoplankton biomass towards summer.
For a better assessment of the changes in coastal ecosystems, resources towards well-planned monitoring are needed. Long continuous datasets based on frequent enough sampling, are required to delineate not only the timing of biomass peaks but also bloom duration (amplitude and modality). Traditionally, the spring bloom has been thought of as the main carbon input to the pelagic (and benthic) system, and therefore the focus of most studies has been on the spring period, often ignoring the summer months. Our results point towards a shift in total biomass production towards the summer. However, in order to evaluate trophic and biogeochemical feedbacks in a system strongly affected by human-induced environmental change requires monitoring of production, grazing and export of organic matter in addition to stocks, as well as their dynamics over the whole seasonal cycle.
Acknowledgements
Funding was received from the Walter and Andrée de Nottbeck Foundation (A. K. A., T. T.) and the Swedish Cultural Foundation in Finland (T. T.). The authors appreciate the work of the authors of the articles from where historical data were obtained, and the Finnish Meteorological Institute and Tvärminne Zoological station for providing data on temperature. The authors kindly acknowledge Mirian Arellano for determining zooplankton biomass in 2016. Two anonymous reviewers are kindly acknowledged for comments that improved the quality of the manuscript.
Disclosure statement
No potential conflict of interest was reported by the author(s).
Additional information
Funding
References
- Aberle N, Bauer B, Lewandowska A, Gaedke U, Sommer U. 2012. Warming induces shifts in microzooplankton phenology and reduces time-lags between phytoplankton and protozoan production. Marine Biology. 159:2441–2453. DOI:10.1007/s00227-012-1947-0.
- Almén A-K, Glippa O, Petterson H, Alenius P, Engström-Öst J. 2017. Changes in wintertime pH and hydrography of the Gulf of Finland (Baltic Sea) with focus on depth layers. Environmental Monitoring and Assessment. 189:147. DOI:10.1007/s10661-017-5840-7.
- Apollonio S, Matrai P. 2011. Marine primary production in the Canadian Arctic, 1956, 1961–1963. Polar Biology. 34:767–774. DOI:10.1007/s00300-010-0928-3.
- BACC II Author Team. 2015. Second assessment of climate change for the Baltic Sea Basin. New York: Springer-Verlag. DOI:10.1007/978-3-319-16006-1.
- Belkin I. 2009. Rapid warming of large marine ecosystems. Progress in Oceanography. 81:207–213. DOI:10.1016/j.pocean.2009.04.011.
- Carstensen J, Andersen JH, Gustafsson BG, Conley DJ. 2014. Deoxygenation of the Baltic Sea during the last century. Proceedings of the National Academy of Sciences of the United States of America. 111:5628–5633. DOI:10.1073/pnas.1323156111.
- Edwards M, Richardson AJ. 2004. Impact of climate change on marine pelagic phenology and trophic mismatch. Nature. 430:881–884. DOI:10.1038/nature02808.
- Elovaara S, Degerlund M, Franklin D, Kaartokallio H, Tamelander T. 2020. Seasonal variation in estuarine phytoplankton viability and its relationship with carbon dynamics in the Baltic Sea. Hydrobiologia. 847:2485–2501. DOI:10.1007/s10750-020-04267-1.
- Feike M, Heerkloss R, Rieling T, Schubert H. 2007. Studies on the zooplankton community of a shallow lagoon of the southern Baltic Sea: long-term trends, seasonal changes, and relations with physical and chemical parameters. Hydrobiologia. 577:95–106. DOI:10.1007/s10750-006-0420-9.
- Forsskåhl M, Laakkonen A, Leppänen J-M, Niemi Å, Sundberg A, Tamelander G. 1982. Seasonal cycle of production and sedimentation of organic matter at the entrance to the Gulf of Finland. Netherlands Journal of Sea Research. 16:290–299. DOI:10.1016/0077-7579(82)90037-0.
- Frenken T, Velthuis M, Domis L, Stephan S, Aben R, Kosten S, van Donk E, Van de Vaal D. 2016. Warming accelerates termination of a phytoplankton spring bloom by fungal parasites. Global Change Biology. 22:299–309. DOI:10.1111/gcb.13095.
- Gammal J, Norkko J, Pilditch C, Norkko A. 2017. Coastal hypoxia and the importance of benthic macrofauna communities for ecosystem functioning. Estuaries and Coasts. 40:457–468. DOI:10.1007/s12237-016-0152-7.
- Griffiths JR, Kadin M, Nascimento FJA, Tamelander T, Törnroos A, Bonaglia S, Bonsdorff E, Brücherts V, et al. 2017. The importance of benthic-pelagic coupling for marine ecosystem functioning in a changing world. Global Change Biology. 23:2179–2196. DOI:10.1111/gcb.13642.
- Groetsch PMM, Simis SGH, Eleveld M, Peters SWM. 2016. Spring blooms in the Baltic Sea have weakened but lengthened from 2000 to 2014. Biogeosciences (online). 13:4959–4973. DOI:10.5194/bg-13-4959-2016.
- Hansen A, Nielsen T, Levinsen H, Madsen S, Thingstad F, Hansen B. 2003. Impact of changing ice cover on pelagic productivity and foodweb structure in Disko Bay. West Greenland: a dynamic model approach. Deep-Sea Research I. 50:171–187.
- Hansson S, Dippner JW, Larsson U. 2010. Climate effects on zooplankton biomasses in a coastal Baltic Sea area. Boreal Environmental Research. 15(3):370–374.
- Heiskanen A-S, Haapala J, Gundersen K. 1998. Sedimentation and pelagic retention of particulate C, N and P in the coastal northern Baltic Sea. Estuarine, Coastal and Shelf Science. 46:703–712.
- Hjerne O, Hajdu S, Larsson U, Downing AS, Winder M. 2019. Climate driven changes in timing, composition and magnitude of the Baltic sea phytoplankton spring bloom. Frontiers in Marine Science. 6:482. DOI:10.3389/fmars.2019.00482.
- HELCOM. 2013. Climate change in the Baltic Sea Area: HELCOM thematic assessment in 2013. Baltic Sea Environmental Proceedings No. 137.
- Hällfors G, Niemi Å. 1974. A Chrysochromulina (Haptophyceae) bloom under the ice in the Tvärminne Archipelago, southern coast of Finland. Memoranda Societatis pro Fauna et Flora Fennica. 50:89–104.
- Kahru M, Elmgren R, Savchuk OP. 2016. Changing seasonality of the Baltic Sea. Biogeosciences (online). 13:1009–1018. DOI 10.5194/bg-13-1009-2016.
- Katajisto T, Viitasalo M, Koski M. 1998. Seasonal occurrence and hatching of calanoid eggs in sediments of the northern Baltic Sea. Marine Ecology Progress Series. 163:133–143.
- Kivi K. 1986. Annual succession of pelagic protozoans and rotifers in the Tvärminne Storfjärden, SW coast of Finland. Ophelia Suppl. 4:101–110.
- Klais R, Tamminen T, Kremp A, Spilling K, Byoung WA, Hajdu S, Olli K. 2013. Spring phytoplankton communities shaped by interannual weather variability and dispersal limitation: Mechanisms of climate change effects on key coastal primary producers. Limnology and Oceanography. 58(2):753–762. DOI:10.4319/lo.2013.58.2.0753.
- Kopp D, Lefebvre S, Cachera M, Villanueva MC, Ernande B. 2015. Reorganization of a marine trophic network along an inshore-offshore gradient due to stronger pelagic-benthic coupling in coastal areas. Progress in Oceanography. 130:157–171. DOI:10.1016/j.pocean.2014.11.001.
- Koski M, Viitasalo M, Kuosa H. 1999. Seasonal development of mesozooplankton biomass and production on the SW coast of Finland. Ophelia. 50:69–91.
- Kuparinen J, Leppänen J-M, Sarvala J, Sundberg A, Virtanen A. 1984. Production and utilization of organic matter in a Baltic ecosystem off Tvärminne, southwest coast of Finland. Conseil International Pour L'Exploration de la Mer. Rapports et Proces-Verbaux des Reunions. 183:180–192.
- Lehmann A, Getzlaff K, Harlaß J. 2011. Detailed assessment of climate variability of the Baltic Sea area for the period 1958–2009. Climate Research. 46(2):185–196. DOI:10.3354/cr00876.
- Lewandowska A, Sommer U. 2010. Climate change and the spring bloom: a mesocosm study on the influence of light and temperature on phytoplankton and mesozooplankton. Marine Ecology Progress Series. 405:101–111. DOI:10.3354/meps0852.
- Lignell R. 1990. Excretion of organic carbon by phytoplankton: its relation to algal biomass, primary productivity, and bacterial secondary productivity in the Baltic Sea. Marine Ecology Progress Series. 68: 85–99.
- Lignell R, Heiskanen A-S, Kuosa H, Gundersen K, Kuuppo-Leinikki P, Pajuniemi R, Uitto A. 1993. Fate of phytoplankton spring bloom: sedimentation and carbon flow in the planktonic food web in the northern Baltic. Marine Ecology Progress Series. 94:239–252.
- Maar M, Hansen JLS. 2011. Increasing temperatures change pelagic trophodynamics and the balance between pelagic and benthic secondary production in a water column model of the Kattegat. Journal of Marine Systems. 85(1–2):57–70. DOI:10.1016/j.jmarsys.2010.11.006.
- Meier M, Eilola K, Almroth E. 2011. Climate-related changes in marine ecosystems simulated with a 3-dimensional coupled physical-biogeochemical model of the Baltic Sea. Climate Research. 48:31–55. DOI:10.3354/cr00968.
- Meier M. 2015. Projected change–marine Physics. In: The BACC II Author Team, editor. Second assessment of climate change for the Baltic Sea basin. Springer International Publishing; p. 960–996.
- Merkouriadi I, Leppäranta M. 2014. Long-term analysis of hydrography and sea-ice data in Tvärminne, Gulf of Finland, Baltic Sea. Climatic Change. 124:849–859. DOI:10.1007/s10584-014-1130–3.
- Möllmann C, Kornilovs G, Sidrevics L. 2000. Long-term dynamics of main mesozooplankton species in the central Baltic Sea. Journal of Plankton Research. 22:2015–2038. DOI:10.1093/plankt/22.11.2015.
- Nascimento FJA, Karlson AML, Naslund J, Gorokhova E. 2009. Settling cyanobacterial blooms do not improve growth conditions for soft bottom meiofauna. Journal of Experimental Marine Biology Ecology. 368:138–146. DOI:10.1016/j.jembe.2008.09.014.
- Niemi Å, Åström A-M. 1987. Ecology of phytoplankton in the Tvärminne area, SW coast of Finland. IV. Environmental conditions, chlorophyll a and phytoplankton in winter and spring 1984 at Tvärminne Storfjärd. Annales Botanici Fennici. 24:333–352.
- Raateoja M, Seppälä J, Kuosa H, Myrberg K. 2005. Recent changes in trophic state of the Baltic Sea along SW coast of Finland. Ambio. 34:188–191.
- Raateoja M, Hällfors H, Kaitala S. 2018. Vernal phytoplankton bloom in the Baltic Sea: intensity and relation to nutrient regime. Journal of Sea Research. 138:24–33. DOI:10.1016/j.seares.2018.05.003.
- Richardson A. 2008. In hot water: zooplankton and climate change. ICES Journal of Marine Science. 65:279–295. DOI:10.1093/icesjms/fsn028.
- Rodil I, Lucena-Moya P, Tamelander T, Norkko J, Norkko A. 2020. Seasonal variability in benthic-pelagic coupling: Quantifying organic matter inputs to the seafloor and benthic macrofauna using a multi-marker approach. Frontiers in Marine Science. 7:404. DOI:10.3389/fmars.2020.00404.
- Sommer U, Gliwicz ZM, Lampert W, Duncan A. 1986. The PEG-model of seasonal succession of planktonic events in fresh waters. Archivfür Hydrobiologie. 106:433–471.
- Sommer U, Lengfellner K. 2008. Climate change and the timing, magnitude and composition of the phytoplankton spring bloom. Global Climate Change. 14:1199–1208. DOI:10.1111/j.1365-2486.2008.01571.x.
- Sommer U, Lewandowska A. 2011. Climate change and the phytoplankton spring bloom: warming and overwintering zooplankton have similar effects on phytoplankton. Global Change Biology. 17:154–162. DOI:10.1111/j.1365-2486.2010.02182.x.
- Sommer U, Aberle N, Lengfellner K, Lewandowska A. 2012. The Baltic Sea spring phytoplankton bloom in a changing climate: An experimental approach. Marine Biology. 159:2479–2490. DOI:10.1007/s00227-012-1897-6.
- Spilling K. 2007. Dense sub-ice bloom of dinoflagellates in the Baltic sea, potentially limited by high pH. Journal of Plankton Research. 29:895–901. DOI:10.1093/plankt/fbm067.
- Spilling K, Lindström M. 2008. Phytoplankton life cycle transformation lead to species-specific effects on sediment processes in the Baltic Sea. Continental Shelf Research. 28:2488–2495. DOI:10.1016/j.csr.2008.07.004.
- Spilling K, Olli K, Lehtoranta J, Kremp A, Tedesco L, Tamelander T, Klais R, Peltonen H, Tamminen T. 2018. Shifting diatom – dinoflagellate dominance during spring bloom in the Baltic Sea and its potential effects on biogeochemical cycling. Frontiers in Marine Science. 5:327. DOI:10.3389/fmars.2018.00327.
- Sunda WG, Cai WJ. 2012. Eutrophication induced CO2-acidification of subsurface coastal waters: interactive effects of temperature, salinity, and atmospheric PCO2. Environmental Science and Technology. 46:10651–10659. DOI:10.1021/es300626f.
- Tamelander T, Heiskanen A-S. 2004. Effects of spring bloom phytoplankton dynamics and hydrography on the composition of settling material in the coastal northern Baltic Sea. Journal of Marine Systems. 52(1–4):217–234. DOI:10.1016/j.jmarsys.2004.02.001.
- Tamelander T, Aubert AB, Wexels Riser C. 2012. Export stoichiometry and contribution of copepod faecal pellets to vertical flux of particulate organic carbon, nitrogen and phosphorous. Marine Ecology Progress Series. 459:17–28. DOI:10.3354/meps09733.
- Tamelander T, Spilling K, Winder M. 2017. Organic matter export to the seafloor in the Baltic sea: drivers of change and future projections. Ambio. 46:842–851. DOI:10.1007/s13280-017-0930-x.
- Vanharanta M, Elovaara S, Franklin D, Spilling K, Tamelander T. 2020. Viability of pico- and nanophytoplankton in the Baltic Sea during spring. Aquatic Ecology. 54:119–135. DOI:10.1007/s10452-019-09730-3.
- Velthuis M, Domis L, Frenken T, Stephan S, Kazanjian G, Aben R, Hilt S, Kosten S, van Donk E, Van de Vaal D. 2017. Warming advances top-down control and reduces producer biomass in a freshwater plankton community. Ecosphere (Washington, DC). 8:e01651. DOI:10.1002/ecs2.1651.
- Viitasalo M, Vuorinen I, Saesmaa S. 1995. Mesozooplankton dynamics in the northern Baltic Sea: implications of variations in hydrography and climate. Journal of Plankton Research. 17:1857–1995. DOI:10.1093/plankt/17.10.1857.
- Viitasalo M, Rosenberg M, Heiskanen A-S, Koski M. 1999. Sedimentation of copepod fecal material in the coastal northern Baltic Sea: where did all the pellets go? Limnology and Oceanography. 44:1388–1399.
- Wasmund N, Nausch G, Gerth M, Busch S, Burmeister C, Hansen R, Sadkowiak E. 2019. Extension of the growing season of phytoplankton in the western Baltic Sea in response to climate change. Marine Ecology Progress Series. 622:1–6. DOI:10.3354/meps12994.
- Wassmann P. 1998. Retention vs. export food chains: processes controlling sinking loss from marine pelagic systems. Hydrobiologia. 363:29–57. DOI:10.1023/A:1003113403096.
- Wexels Riser C, Wassmann P, Olli K, Pasternak A, Arashkevich E. 2002. Seasonal variation in production, retention and export of zooplankton faecal pellets in the marginal ice zone and central Barents Sea. Journal of Marine Systems. 38:175–188. DOI:10.1016/S0924-7963(02)00176-8.
- Winder M, Sommer U. 2012. Phytoplankton response to a changing climate. Hydrobiologia. 698:5–16. DOI:10.1007/s10750-012-1149-2.
- Winder M, Schindler D. 2004. Climate change uncouples trophic interactions in an aquatic ecosystem. Ecology. 85:2100–2106. DOI:10.1890/04-0151.