ABSTRACT
The invasive brown alga Sargassum muticum was first recorded in Norway in 1988 and is presently common in the sublittoral fringe on the sheltered coast in South Norway. Here, results from a study on mesofauna associated with S. muticum in an archipelago on the southwest coast of Norway are presented, with two stations placed in sounds exposed to tidal currents and two in sheltered bays. Sargassum muticum occurred with a patchy distribution and an estimated density of less than 7 individuals m−2 at three of the stations (two in sheltered bays and one in a sound) and 42 individuals m−2 at the fourth station (placed in a sound). A total of 102 distinct faunal taxa were identified, with an average of 31 taxa per sample. Crustacea and Gastropoda constituted around half of the identified taxa. Fauna richness showed no relation to site type but had a curvilinear correlation with habitat size (dry weight of thallus). Total and sessile fauna richness showed a significant negative correlation with branching density. Vagile faunal density showed a curvilinear correlation with weight of epiphytes and was significantly related to site type with higher densities in the bays. The community composition of vagile fauna reflected this and was significantly related to site type, but not to epiphyte weight or branching density. When including sessile taxa in a community analysis the stations became less separated, and the community was significantly influenced by branch density. Thus, both site characteristics and morphology of S. muticum influenced the associated fauna community.
Introduction
Seaweeds represent important habitats for marine micro-, meso- and macrofauna by providing substratum, shelter or food resources for the associated fauna (e.g. Vandendriessche et al. Citation2007; Christie et al. Citation2009). While some animals are closely associated with specific seaweeds for substratum or food, others are generalists and can be found on several seaweed species (e.g. Sotka et al. Citation1999; Viejo Citation1999; Armitage and Sjøtun Citation2016).
An important question is whether introduced seaweeds can harbour a similar fauna richness and density as native seaweeds do. In particular, introduced seaweeds may lack specially adapted taxa that are associated with native seaweeds. When comparing the associated fauna on the introduced seaweed Codium fragile subsp. fragile (Suringar) Hariot and the native brown alga with a similar habit, Fucus serratus Linnaeus, Armitage and Sjøtun (Citation2016) found that the native fucoid had more of some specialized mesograzers. Such differences may have consequences for local food webs. In a field experiment from a site in Ireland, Salvaterra et al. (Citation2013) found that substituting or adding the introduced Sargassum muticum (Yendo) Fensholt in native communities had a strong impact on food web structure, probably caused by strong alterations of fauna composition, including more generalists on S. muticum. On the other hand, several studies have shown that differences in faunal composition and abundance between introduced and native seaweeds are mainly caused by differences in structural complexity (e.g. Veiga et al. Citation2014, Citation2016; Lutz et al. Citation2019), suggesting that, for example, type of branching of introduced seaweeds is of most significance for the faunal composition. In a study from Helgoland, Germany, Bushbaum et al. (Citation2006) found that S. muticum supported a very similar faunal community to that of the native Halidrys siliquosa (Linnaeus) Lyngbye, which has a morphology and branching structure relatively similar to that of S. muticum.
The habitat architecture of seaweeds seems to be one of the most important factors determining the overall faunal richness and composition (e.g. Veiga et al. Citation2014; Suárez-Jiménez et al. Citation2017). By mimicking different seaweed structures using different types of artificial substratum, Christie et al. (Citation2007) showed that a smooth surface was particularly unattractive for colonizing fauna, while a branched structure was most attractive. Type of branching or degree of branching may further influence fauna composition. For example, Chemello and Milazzo (Citation2002) compared the molluscan fauna on several brown algae and found that the branching complexity of different species could explain the differences in the composition of associated molluscs. Eilertsen et al. (Citation2011) studied the amphipod fauna on three red algal species with contrasting morphology and found that the algal thallus organization and branching pattern had a strong impact on the composition of the associated amphipods. Veiga et al. (Citation2014) compared the fauna composition and abundance associated with three different macroalgal species and found that the macroalgal architecture played a significant role in shaping epifaunal assemblages. However, the most complex macroalgae do not always have the highest epifaunal abundances, and for example Desmond et al. (Citation2018) found some of the highest values of epifaunal abundance were associated with macroalgae of a relatively simple morphology.
The brown alga S. muticum is one of the most well-known and well-studied introduced seaweeds in Europe. It is native to the Western Pacific but has been introduced to western North America and Europe, most likely through import of Pacific oysters from Japan (Engelen et al. Citation2015). It has been present in Norway since 1988 (Rueness Citation1989), whereafter it spread rapidly along the coast of South Norway and reached the southwest coast of Norway in 1991 (Steen Citation1992). Its northernmost record is at present at 62°55′N, 7°26′E (https://www.biodiversity.no/).
Sargassum muticum shows a strong seasonal variation in morphology, which is most pronounced in the higher latitudes of its introduced areas (Engelen et al. Citation2015). During the dormancy period, which is normally during winter in Europe, it is reduced to the holdfast and basal parts (Engelen et al. Citation2015). In late winter and spring it forms long upright main axes, followed by formation of spirally set side branches. The onset of the main axes has been shown to be under photoperiodic control (Hwang and Dring Citation2002), and the branching organization and apical growth dominance over lateral branch formation is most likely regulated by phytohormone production (Chamberlain et al. Citation1979). In its northern range of distribution in Europe the leaves and gas vesicles are often shed during the last part of the summer (authors’ pers. obs.).
Sargassum muticum can grow to a relatively large size and forms a complex thallus with a densely branched habit and small gas bladders and leaves. This should make it an excellent habitat for many associated invertebrates in its introduced ranges. For example, Viejo (Citation1999) found between 30 and 40 fauna taxa associated with S. muticum in northern Spain, and Bushbaum et al. (Citation2006) found a total of around 60 taxa (fauna and macroalgae) associated with S. muticum in the North Sea. The strong seasonality and small overwintering basal parts will on the other hand restrict the availability of the habitat to a relatively short period of the year for many taxa and make it less suitable for algal epiphytes or sessile perennial taxa. To what degree it represents food for associated native fauna is not clear. Introduced species may escape their specialized predators or parasites, and this may also explain the success of some invading species (the Enemy Release Hypothesis; see Heger and Jeschke Citation2014). In the areas where it has been introduced some attempts have been made to investigate degree of grazing on S. muticum, but the results are pointing in different directions. While some studies show that S. muticum is less favoured by macro- and mezograzers than native seaweeds in an introduced area (e.g. Britton-Simmons Citation2004; Monteiro et al. Citation2009; Cacabelos et al. Citation2010; Engelen et al. Citation2011), others have found that specific native mesograzers graze more on S. muticum than on native seaweeds, e.g. Strong et al. (Citation2009) (Dexamine spinosa Montagu, 1813) and Britton-Simmons et al. (Citation2011) (Lacuna vincta Montagu, 1803).
In southwest Norway S. muticum occurs with a patchy distribution in the sublittoral fringe and upper subtidal. It often grows to large plants in places with strong tidal currents such as narrow sounds, while it may show reduced size in more sheltered areas (). Strong spatial variation in abundance, phenology and size was also demonstrated for S. muticum in northern Spain, where Olabarria et al. (Citation2009) recorded size and development at six localities. This suggests that S. muticum may in general show a strong phenotypic variation in response to local environmental conditions. This may in turn affect its associated fauna composition since phenotypic changes in size and branching are likely to influence amount and composition of the associated fauna. In addition, site characteristics may also influence composition of associated fauna. For example, Bushbaum et al. (Citation2006) found different fauna compositions on S. muticum from two different types of biotopes.
Figure 1. Study area west of Bergen with localization of stations A–D, and with photos of Sargassum muticum from the different stations included. (Photos: M. Eilertsen; maps: Kartagena, Statens kartverk and Vemaps.com).
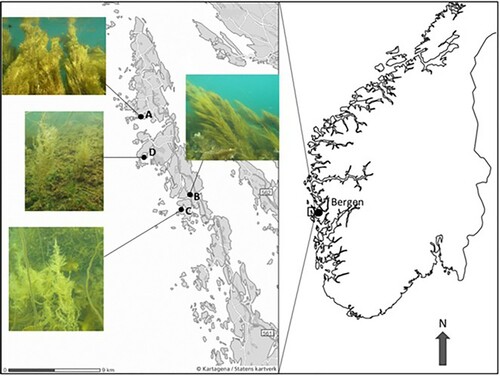
We here present the results from a study on fauna associated with S. muticum from four localities, situated in either sheltered bays or sounds exposed to tidal currents, within 10 km along the coastal archipelago of southwestern Norway. Morphological features of each individual S. muticum sample were recorded (size, weight, number of lateral branches and lateral branch length). The aim of the study was to explore how faunal composition was related to site characteristics and morphological features of the host plant.
Materials and methods
Field collection and laboratory processing
Fieldwork was done during spring low water on 10–13 August 2010 at four stations situated in the outer archipelago of southwestern Norway west of Bergen (). Stations A (60°35.272′N, 04°47.697′E) and B (60°31.033′N, 04°53.454′E) were located in sounds with strong tidal currents, while Stations C (60°30.34′N, 04°52.802′E) and D (60°33.074′N, 04°48.690′E) were in sheltered bays with less influence of tidal currents. All the fieldwork was done by snorkelling and skin diving.
The abundance of S. muticum at the stations was assessed by counting the number of individuals in sample quadrats covering 50 cm × 50 cm. At stations B, C and D a 20 m long rope, marked for each metre, was placed along the shore at the water level. Five random numbers between 1–20 were selected. At each number a 50 cm wide transect, made of sample quadrats placed in a row perpendicular to each other from the water level and as deep as S. muticum were growing (2–2.5 m), was examined. Sargassum muticum individuals were counted in each sample quadrat. At station A the density of S. muticum was much higher, and the S. muticum vegetation persisted to greater depths that at the other stations. Here, five sample quadrats were placed randomly at 30–50 cm depth along the shore, and the S. muticum plants counted in each sample square.
At each station, five S. muticum were sampled haphazardly for fauna identification at 0.5–1.5 m depth, with at least 1–2 m between in order not to disturb the associated vagile fauna of the other samples. Each sample consisted of one complete thallus. Each sampled individual was carefully loosened at the base and, while still submerged, transferred slowly to a cloth bag held open nearby. The bag was thereafter closed and removed from the water. This way of sampling aims to cause negligible disturbance to the associated meso- and small macrofauna and has been used in other studies (e.g. Fredriksen et al. Citation2005; Armitage and Sjøtun Citation2016).
Each sample was thereafter put into a large tray with clean fresh water for some minutes to stun the vagile animals, taking care to rinse the cloth bags carefully for all visible animals. For each sample the fresh water was filtered, and the fauna collected by using a 0.5 mm plankton net. The large individual S. muticum plants were thereafter frozen, and the smaller ones were fixated in 4% formalin solution. The fauna fraction was stored in 4% formalin solution with borax until taxa were sorted and identified.
The frozen or formalin fixated individuals of S. muticum were later processed in the laboratory. Each individual was placed in a basin filled with fresh water and gently shaken, in order to make any remaining vagile fauna fall off. The S. muticum individual was removed for further processing, and the water was filtered again through a 0.5-mm plankton net to collect the fauna.
The main axis length of S. muticum was measured. If there were more than one main axis present, they were counted, and their lengths measured. All first-order laterals >5 cm long were removed and counted per main axis, and the lengths were measured. The density of first-order branches of the main axis or axes of each individual was calculated as mean number of branches per cm main axis. The thallus was thereafter examined for sessile fauna, and samples for identification were collected. All visible algal epiphytes were removed. Finally, each individual and its epiphytes were dried at 70°C until constant weight.
Fauna were identified to lowest taxon possible by using keys and identification literature. The identification work was done using different sources (e.g. Sars Citation1895; Sars Citation1899; Sneli Citation1975; Enckell Citation1980; Hayward and Ryland Citation1995). Approximately three-quarters of the taxa was identified to species level. Acari, copepods, ostracods and nematodes were excluded from the dataset as they were not counted systematically in all samples (due to size and difficulty with identifications).
Data analyses
The entire dataset of vagile fauna was used in analysis of fauna density (individuals per g dry thallus weight). For analysis of taxa richness, 10 indistinct taxa were excluded, being either (a) juveniles where adults of the same species/genus/family were also present, or (b) unidentified members of a higher taxon where identified members of the same genus/family were also present in the data. For multivariate analyses, 18 rare taxa (here defined as only one individual found) were also excluded.
Relationships between taxa richness and explanatory variables were analysed using generalized linear mixed effects models with Poisson distribution (GLMM, R-package lme4; Bates et al. Citation2015). Relationships between vagile fauna density and explanatory variables were analysed using a GLMM with negative binomial distribution, due to overdispersion (R-package glmmTMB; Brooks et al. Citation2017). Model selection and significance of variables was assessed by likelihood ratio test. Interactions were not included because of the low number of data points, but polynomial fits were considered for certain variables based on visual inspection of the data and model diagnostic plots, which were made using R-package DHARMa (Hartig Citation2020).
Explanatory variables included in the analysis of taxa richness were habitat size (dry weight of the thallus and epiphytic algae, g), branching density (density of first-order laterals per cm of main axis), and site type (sound or sheltered bay), with station as a random effect. Thallus and epiphyte weight were combined into one variable as they were positively correlated (0.65), to avoid issues with multicollinearity (checked with variance inflation factor analysis; R-package car, Fox and Weisberg Citation2019). For the vagile fauna density analysis, where abundances were standardized to individuals per g dry thallus weight, the included variables were epiphyte abundance (dry weight, g), branching density, site type and station (random effect).
Community composition of the associated fauna was analysed using multivariate statistics (R package vegan; Oksanen et al. Citation2020). NMDS was performed with Bray–Curtis dissimilarities of the vagile fauna densities (individuals per g dry thallus weight), with densities square root transformed to limit the influence of very abundant groups (e.g. juvenile Rissoa/Pusillina). A second NMDS including the sessile fauna was also done, where densities were transformed to presence–absence and Jaccard dissimilarities were used. Relationships between community composition and explanatory variables were assessed using permutational multivariate analysis of variance (adonis in vegan; stratified by station). Associations between specific taxa and site type (sheltered bay or sound) were estimated using correlation indices (phi coefficient of association and point biserial correlation in R package indicspecies; De Caceres and Legendre Citation2009).
Results
Density and morphological characteristics of Sargassum muticum
The estimated densities of S. muticum at the stations varied from 42.4 ± 27.3 plants m−2 at station A to 1.1 ± 1.0 m−2 at station B. The remaining stations had intermediate densities; at station C the estimated density was 6.7 ± 2.0 m−2, and at station D it was 4.8 ± 1.3 m−2 (figures represent averages ± conf. int. per station).
On average, the largest sampled individuals were from Station A (between 95 and 153 cm maximum length and 3.2 and 50.5 g dry weight), while the smallest were from Station D (between 58 and 90 cm maximum length and 0.9–9.9 g dry weight). Most of the sampled individuals from Station B and C were intermediate with lengths between 65 and 128 cm, and weights between 1.5 and 20.7 g (). Most of the sampled individuals had 1 or 2 main axes, but at station A there was one thallus with 3 main axes, and at station C there was one with 4, and another with 7 main axes. Branching density varied from 0.5 to 1.3 first-order laterals per cm of main axis. Average values per station varied from 0.7 ± 0.1 at station A to 1.0 ± 0.2 at station D (mean ± SD). Branching density was uncorrelated with the dry weight of the S. muticum sample (Pearson correlation = 0.1) and was not related to site type (sound or bay) (see ).
Figure 2. Violin plots showing thallus characteristics of Sargassum muticum (n = 5), including (left) thallus dry weight (g), (centre) no. of side branches per cm and (right) epiphyte dry weight (g), at stations A–D. The shapes show data spread, while the black point shows the mean for each station. Type of site shown by shading (sound = light grey, sheltered bay = dark grey).
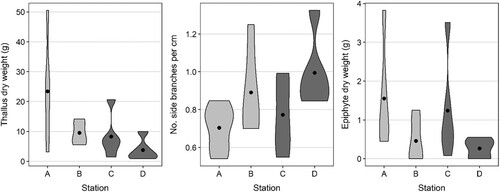
Stations B and D had on average the lowest loads of epiphytic algae on the S. muticum thalli (). This was however variable within stations; for example, at station C, three of the thalli had under 0.7 g dry weight of epiphytes while another had 3.5 g. Thin, filamentous algae dominated the epiphytes, with Cladophora sp., Rhizoclonium sp. and Ceramium sp. being the most common genera.
Associated fauna taxa richness
A total of 102 distinct faunal taxa were found associated with the 20 sampled S. muticum thalli: 63 vagile and 39 sessile taxa, with 88 identified to species level. The full species dataset is available in dataverse.no at https://doi.org/10.18710/QZ1AWV. The number of taxa per sample varied from 12–44 taxa (average 31.3 ± 9.0 SD taxa per sample). The most common groups were Crustacea and Gastropoda, which together constituted around half the identified taxa, while sessile taxa from the groups Bryozoa and Cnidaria constituted more than 25% of the total number of taxa identified (). The most frequently occurring sessile taxa were the bryozoans Juxtacribrilina annulata (Fabricius, 1780), Celleporella hyalia (Linnaeus, 1767), Celleporina decipiens (Hayward, 1976) and Membranipora membranacea (Linnaeus, 1767), and the bivalves Hiatella artica (Linnaeus, 1767) and juvenile Mytilus edulis (Linnaeus 1758).
Figure 3. Overview of number of faunal taxa associated with Sargassum muticum (102 taxa; this excludes juveniles or unidentified species which had congeners present, 10 taxa). Labels indicate number of taxa within each group; the three sections without labels are Echinodermata, Insecta and Porifera with 1 taxon each.
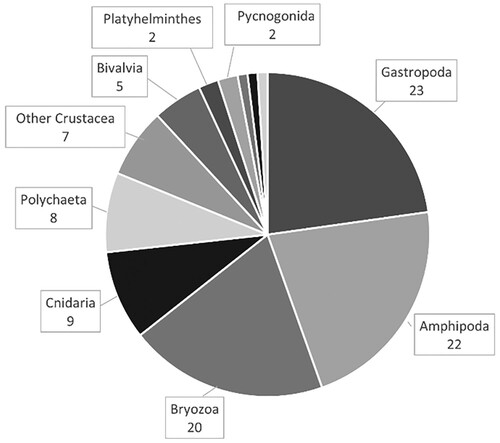
The number of fauna taxa present per sample showed a significant positive correlation to the habitat size (thallus and epiphytic algal weight; χ22 = 11.0, P = 0.004), but the increase in number of taxa was only evident up to around 25–35 g thallus dry weight, with no increase of species richness with increasing size above this (). A similar relationship was visible when only vagile (χ22 = 6.41, P = 0.04) or sessile taxa (χ22 = 7.72, P = 0.02) were analysed ().
Figure 4. The number of associated taxa per Sargassum muticum sample related to dry weight (g) of the thallus and epiphytes (‘habitat’). Taxa richness is shown for all taxa (a), vagile taxa (b) and sessile taxa (c). The line is the prediction of the model (at average branch density for total and sessile fauna where this variable was significant). Type of site is shaded (sound = light grey, sheltered bay = dark grey).
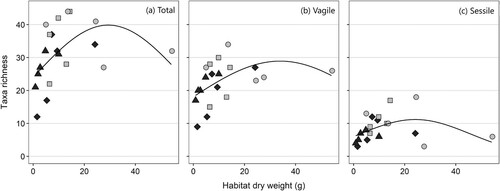
Taxa richness was negatively correlated with the density of first-order lateral branches of main axes (χ21 = 8.50, P = 0.004) (). However, when analysing vagile and sessile taxa separately, the correlation between branching density and number of taxa was only found to be significant for the sessile component of fauna (χ21 = 9.94, P = 0.002; ). There was no significant relationship between taxa richness and site type (sheltered bays or sounds; χ21 = 1.77, P = 0.18).
Figure 5. The number of associated taxa per Sargassum muticum sample related to branching density (number of primary laterals per cm main axis). Taxa richness is shown for all taxa (a), vagile taxa (b) and sessile taxa (c). The line is the prediction of the model (at average habitat dry weight); there was no significant relationship for the vagile fauna. Type of site is shaded (sound = light grey, sheltered bay = dark grey).
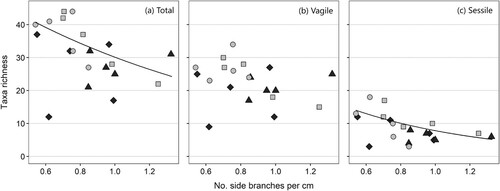
Associated fauna density
Of the vagile taxa, the groups Gastropoda and Amphipoda dominated in numbers. In terms of density (individuals per g dry weight), the most abundant gastropods were Rissoa parva (da Costa, 1778), Lacuna vincta and Rissoella opalina (Jeffreys, 1848), while the most abundant amphipods were Caprella acanthifera (Leach, 1814), Microprotopus maculatus (Norman, 1867), Dexamine thea (Boeck, 1861), D. spinosa, Ampithoe gammaroides (Spence Bate, 1857), Crassicorophium bonelli (Milne-Edwards, 1830) and Phtisica marina (Slabber, 1769) (See full species dataset in dataverse.no at https://doi.org/10.18710/QZ1AWV). There were however large differences between stations and samples. High vagile fauna density at station C was driven by high densities of gastropods (nearly 95 adult individuals per g dry weight; ≤ 25 at stations A, B and D), and at station D by high densities of amphipods (260 individuals per g dry weight; < 45 at stations A, B and C) ().
Figure 6. Abundances of gastropods and amphipods per gram thallus in each sample of Sargassum muticum, plotted by station. ‘Adult’ groups have juveniles excluded. Type of site is shaded (sound = light grey, sheltered bay = dark grey).
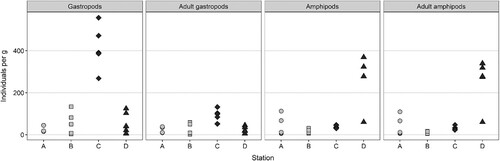
Density of vagile fauna was highly related to site type (χ21 = 15.4, P < 0.001), with densities much higher at the sheltered sites (Stations C and D) than at the stations in the sounds (Station A and B; ). A positive but curved relationship of density of vagile fauna to abundance of epiphytic macroalgae was also suggested (χ22 = 11.2, P = 0.004), with an increase in fauna density up to around 2 g dry weight epiphytic macroalgae per thallus (). However, this relationship should be interpreted cautiously given that few samples had high epiphyte weights, potentially allowing the 1–2 samples with high weights to disproportionally affect the analysis. We have also not accounted for the potential effect of fauna density increasing with thallus weight, and some of the relationship to epiphyte weight could be due to this (due to the correlation between these two factors). An experimental design with manipulated amounts of epiphytic algae would be needed to confirm this result. No significant relationship to branching density was found (χ21 = 1.98, P = 0.16).
Figure 7. Abundances of vagile fauna (individuals per gram thallus dry weight) in each sample of Sargassum muticum, plotted against dry weight of epiphytic algae. The lines are the predictions of the model. Type of site is indicated by line type and colour (sound = light grey, dotted line; sheltered bay = dark grey, solid line).
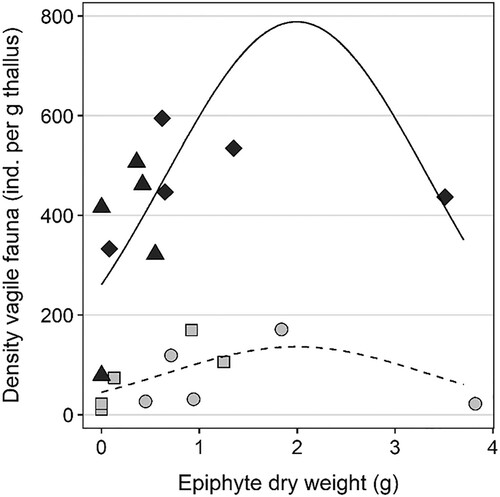
Associated fauna community composition
The vagile fauna community composition was similar at the stations in sounds, A and B, while the composition at the sheltered stations, C and D, was more dissimilar, both from each other and from stations A and B (). Community composition was significantly related to site type (R2 = 0.32, P = 0.03) but not epiphyte weight or density of side branches. When sessile fauna was also included, with vagile fauna converted to presence–absence, the stations became less separated in the NMDS plot but were still gathered by site type (sound or bay) (). Here community composition was related to the density of side branches (R2 = 0.08, P = 0.01), but not to site type or epiphyte weight.
Figure 8. NMDS plot of the composition of the vagile fauna community associated with Sargassum muticum (excluding unsure or rare species, 49 taxa), stress = 0.09. Based on a Bray–Curtis dissimilarity matrix on square-root transformed species density data (individuals per g thallus dry weight).
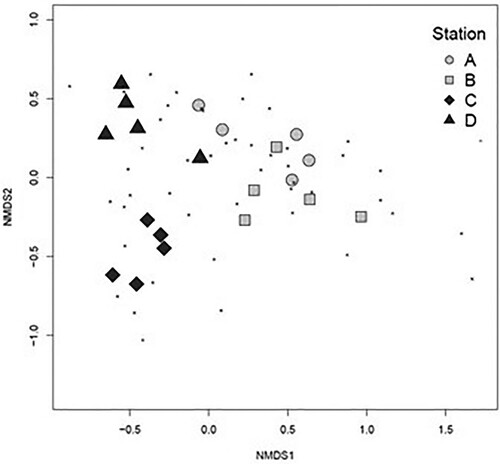
Figure 9. NMDS plot of the composition of the sessile and vagile fauna community associated with Sargassum muticum (excluding unsure or rare taxa, 84 taxa), stress = 0.14, Jaccard distance matrix on presence-absence data. The vector shows the relationship of branch density (number of primary laterals per cm main axis) with community composition. Samples are shaded by site type (sound = light grey; sheltered bay = dark grey).
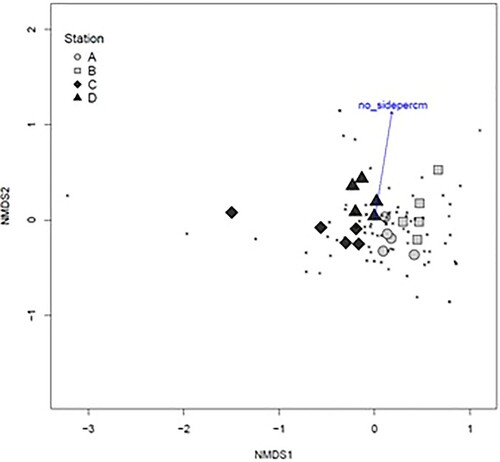
Several taxa were associated with the high-current, sound site type; these included the bryozoans Celleporina decipiens, Membranipora membranacea, Celeporella hyalina, and Electra pilosa (Linnaeus, 1767), juvenile Syllidae (Polychaeta), juvenile Asterias rubens Linnaeus, 1758 (Echinodermata), and the gastropods Skenea serpuloides (Montagu, 1808) and Doto coronata (Gmelin, 1791) (). Taxa associated with the sheltered, bay site type were more dominated by amphipods and gastropods; this included Pusillina sarsii (Lovén 1846), Rissoa parva and Littorina obtusata (Linnaeus 1758) from the Gastropoda, Ampithoe gammaroides, Dexamine spinosa, Microprotopus maculatus, Phtisica marina and Crassicorophium bonellii from the Amphipoda, and one polychaete, Websterinereis glauca (Claparède, 1870) ().
Table I. Results of multilevel pattern analysis: (A) Vagile species associations to site type, based on abundances (point biserial correlation coefficient; pb), (B) All species associations to site type, based on presence-absence (phi coefficient of association; phi). Both using association function r.g, permutations = 999. References to main food: (1) Guerra-García et al. (Citation2014), (2) Dixon and Moore (Citation1997), (3) Steneck and Watling (Citation1982), (4) Lambert (Citation1991), (5) Riisgård and Manríquez (Citation1997), – no information.
Discussion
Taxa richness
We found an equal or higher number of faunal taxa associated with S. muticum in this study compared with earlier studies from Europe (e.g. Viejo Citation1999; Wernberg et al. Citation2004; Bushbaum et al. Citation2006; Cacabelos et al. Citation2010), which may reflect a fauna pool with high diversity in the investigated area. The fact that complete thalli of S. muticum were sampled in the present study may also be of significance in the comparison with other findings. For example, Bushbaum et al. (Citation2006) reported a lower number of faunal taxa associated with S. muticum than the present study. This could be caused by subsampling of branches in Bushbaum et al. (Citation2006), which would exclude faunal taxa associated with the basal parts of the thalli. In addition, the results of the present study showed that richness of associated fauna increased with habitat size up to a limit, which means that only subsampling part of S. muticum individuals could result in an underestimation of the number of faunal taxa.
A curved relationship between habitat size and vagile fauna taxa richness was found, suggesting that increasing thallus size and/or occurrences of epiphytes can result in an increase in fauna richness, but only to a certain limit. This could be a sample size effect, since it is common that species accumulate with sample size up to a limit. This sample limit normally depends on the scale of the study and the species pool present (Chase and Knight Citation2013). However, some of the effect could also be due to the additional effect of the epiphytic algal component to the habitat. Epiphytes will add other habitat elements to the overall habitat represented by the host alga, for example by having a different type of thallus structure or a different branching pattern (Christie et al. Citation2007). This may provide habitats for an additional fauna component. A somewhat similar relationship between epiphytes on the fucoid Fucus serratus and the chlorophyte Codium fragile subsp. fragile and faunal richness inhabiting the two macroalgae was found by Armitage and Sjøtun (Citation2016), and a significant positive correlation between epiphytic biomass and number of species was found by Gestoso et al. (Citation2012). Jacobucci et al. (Citation2009) found that the amphipod diversity and evenness were positively influenced by epiphytes on Sargassum filipendula C.Agardh, and attributed this to an increase in attachment sites, shelter and food supply by epiphytes. Further, a curved relationship between epiphyte biomass and density of vagile fauna was found in the present study, underlining the importance of the epiphytic component for the total community. In a similar way Armitage and Sjøtun (Citation2016) also found a correlation between density of several fauna groups and epiphyte weight. In further studies, manipulation of epiphytic abundance could test the significance of epiphytes for the fauna richness and density associated with large macroalgae.
In addition to the variation in size of the collected S. muticum, differences in branching density of the first-order side branches were evident, unrelated to site type. This could be due to local light conditions influencing the morphology of the sampled individuals (see Arenas et al. Citation2002). Alternatively, it could be due to grazers causing a more open structure through removal of side branches. However, a significant correlation between the vagile fauna density and branch density was not observed in the present study, contradicting that grazing is likely to influence branching density. On the other hand, we found a negative correlation between the sessile fauna richness and branching density, suggesting that a more open branching was favourable for many sessile taxa. This may possibly be connected to food availability for sessile taxa, since dense branching may reduce water flow through the thalli, which would consequently result in less food for sessile filter feeders and particle feeders. Bryozoa, a dominant component of the sessile fauna in the present study, is very dependent on availability of planktonic algae for growth (O'Dea and Okamura Citation1999).
Most studies have used different macroalgal species when testing the potential effect of seaweed morphology and branching structure on the associated fauna composition (e.g. Veiga et al. Citation2014; Suárez-Jiménez et al. Citation2017), or artificial substratum (e.g. Christie et al. Citation2007). However, other macroalgal features than the overall habitat architecture, e.g. surface texture or content of secondary metabolites, may also influence the composition of the associated fauna (e.g. Crisp and Williams Citation1960; Williams Citation1964; Wickström and Pavia Citation2004). This makes it difficult to eliminate the species component in such comparisons. In the present study the effect of intraspecific morphological variation on fauna composition was studied in one species, and different branching density of S. muticum was shown to have a significant impact on the sessile faunal component. A similar result was found by Veiga et al. (Citation2018) when comparing the fauna on S. muticum to a more densely branched species of the genus, S. flavifolium Kützing, and where they found higher abundance of two filter-feeding bivalves on the more open-branched S. muticum. This could suggest that a dense branching architecture in general could represent a particularly unfavourable habitat for some sessile filter or particle feeding invertebrates.
Fauna composition
Both site characteristics and morphology of the host species seem to be important in shaping the composition of the associated fauna community, which is in accordance with earlier findings (e.g. Bushbaum et al. Citation2006; Cacabelos et al. Citation2010; Engelen et al. Citation2013; Desmond et al. Citation2018). The community analysis of the vagile fauna component showed a divergence between stations, where the two sites from the sounds formed a unit which was partly separated from the stations in the bays. Furthermore, the vagile fauna composition of the two stations in the bays were clearly separated from each other. This supports the results from the analyses of fauna densities and species associations, where the bays had much higher densities of vagile fauna than the sounds, and were somewhat dissimilar, with one station being dominated by gastropods and the other by amphipods. The difference in dominant groups at the bay sites is difficult to explain from this study alone, as we sampled only two stations for each habitat type. Similarities between the faunal community in the sounds nevertheless suggest that there was a somewhat distinct associated faunal community in the sounds. Surveys of additional stations could confirm if these findings can be generalized.
When including the sessile component of the fauna and analysing data as presence–absence, the samples from sounds and bays still formed separate clusters, but with less separation between the two community types. In this analysis, the most important factor influencing the overall community structure was branching density. That this factor only became significant in the analysis with sessile fauna included indicates that it was most relevant for this component of the fauna, and ties with our hypothesis that more open branching is favourable for sessile taxa. This can be connected to a better flow of water through thalli with more open branching, allowing a better supply of food particles for filtrating or particle feeding taxa. This water-flow hypothesis could also explain why several bryozoan species were particularly associated with S. muticum in sounds, where tidal currents are stronger.
The most abundant gastropods (Rissoa parva, Lacuna vincta and Rissoella opaline) and some of the abundant amphipods (Dexamine spinosa and Ampithoe gammaroides) associated with S. muticum in the present study are partly or fully herbivorous (Steneck and Watling Citation1982; Guerra-García et al. Citation2014). It is likely that these graze on S. muticum and/or its epiphytes. Britton-Simmons et al. (Citation2011) found that the gastropod Lacuna vincta was more abundant on S. muticum than on native seaweeds at all examined sites in the USA, and it also preferred to graze on S. muticum. Strong et al. (Citation2009) observed that the amphipod Dexamine spinosa was more abundant on S. muticum than on native seaweeds in Northern Ireland. They concluded that grazing together with ectocarpoid fouling had a severe negative impact on S. muticum at the studied site. In the present study, the densities of gastropods and amphipods were higher in the bays than in the sounds, possibly causing higher grazing on S. muticum at these sites. The potential impact of grazers on S. muticum in its northern area of distribution in Europe is not known. Results from a cage experiment excluding mesograzers indicate that grazing could be an important factor reducing the recruitment of S. muticum in this area (Sjøtun et al. Citation2007).
Members of Crustacea and Gastropoda represented more than half of the total number of faunal taxa recorded on and associated with S. muticum. This is in line with general findings of dominating mesofaunal groups associated with seaweeds in Norway (Fredriksen et al. Citation2005; Christie et al. Citation2009; Armitage and Sjøtun Citation2016). Small crustaceans and gastropods are important as food for larger animals such as labrids or other small fish in the shallow coastal zone (Norderhaug et al. Citation2005). The gastropods Lacuna vincta and Rissoa parva were among the most commonly occurring gastropods associated with S. muticum in the present study, and according to Norderhaug et al. (Citation2005) these were among the most common prey of fish inhabiting a shallow kelp forest habitat and were primarily eaten by small labrids. This suggests that the vagile mesofauna associated with S. muticum may sustain important coastal food webs. On the other hand, the results of the present study show that gastropods and crustaceans were not equally abundant at all sites but occurred in lower densities in the sounds than in the bays. Also, the occurrences of S. muticum tended to be patchy and in general not deeper than around 2 m, while S. latissima, which S. muticum co-occurred with at the sites, formed kelp beds to greater depths. Thus, even though the numbers of faunal taxa associated with S. muticum is relatively similar to what has been recorded for S. latissima (Christie et al. Citation2009), S. latissima may occur in much larger abundances and is for that reason most likely more important for the coastal ecosystems than S. muticum. In addition, the thallus morphology of S. muticum in the North Atlantic is highly seasonal, with the thallus fronds growing through the spring and starting to deteriorate already in August, with the remainder of the thallus overwintering in a very reduced state. In addition, due to the floating ability of the fronds much of the biomass will float away. This will reduce the value of S. muticum as a stable habitat for the associated fauna, and it will probably represent a particularly unfavourable habitat for sessile and perennial taxa, like many bryozoans and hydroids.
During the last decades S. latissima has shown strong variations in abundance and in particular a strong decline along the southern coast of Norway (Moy and Christie Citation2012). Sargassum muticum tolerates temperatures up to 30°C (Engelen et al. Citation2015) and may for that reason survive heatwaves well, which raises the question whether S. muticum may increase in abundance locally as the climate changes and the sea temperature rises. If this was the case it could partly take over the ecological role S. latissima has in the coastal ecosystem, by providing shelter and food for associated mesofauna during its growth season in spring and summer. However, results from a field experiment performed during two successive summers in southwestern Norway and which included among others S. latissima and S. muticum, seem to partly contradict this. A heatwave occurred during one of the summers, and while S. latissima was clearly negatively affected by this, S. muticum was deteriorating at the end of both summers, irrespective of temperature conditions (Armitage et al. Citation2017). On the other hand, S. muticum showed significantly higher survival in some of the experimental conditions during the hot summer. This suggests that S. muticum may increase in abundance in a future scenario of more frequent heatwaves, but it will probably not be able to fully replace kelp as the foundation species along the coast of the North Atlantic.
Acknowledgements
We thank the crew of R/V ‘Hans Brattström’ for their practical assistance during the fieldwork.
Disclosure statement
No potential conflict of interest was reported by the authors.
Additional information
Funding
Literature
- Arenas F, Viejo RM, Fernández C. 2002. Density-dependent regulation in an invasive seaweed: responses at plant and modular levels. Journal of Ecology. 90:820–829. doi:https://doi.org/10.1046/j.1365-2745.2002.00720.x
- Armitage CS, Husa V, Petelenz-Kurdziel EA, Sjøtun K. 2017. Growth and competition in a warmer ocean: A field experiment with a non-native and two native habitat building seaweeds. Marine Ecology Progress Series. 573:85–99. doi:https://doi.org/10.3354/meps12161
- Armitage CS, Sjøtun K. 2016. Epiphytic macroalgae mediate the impact of a non-native alga on associated fauna. Hydrobiologia. doi: https://doi.org/10.1007/s10750-016-2707-9.
- Bates D, Maechler M, Bolker B, Walker S. 2015. Fitting linear mixed-Effects Models using lme4. Journal of Statistical Software. 67:1–48. doi:https://doi.org/10.18637/jss.v067.i01.
- Britton-Simmons KH, Pister B, Sánchez I, Okamoto D. 2011. Response of a native, herbivorous snail to the introduced seaweed Sargassum muticum. Hydrobiologia. 661:187–196. doi:https://doi.org/10.1007/s10750-010-0523-1
- Britton-Simmons KH. 2004. Direct and indirect effects of the introduced alga Sargassum muticum on benthic, subtidal communities of Washington state, USA. Marine Ecology Progress Series. 277:61–78. doi:https://doi.org/10.3354/meps277061
- Brooks ME, Kristensen K, van Benthem KJ, Magnusson A, Berg CW, Nielsen A, Skaug HJ, Maechler M, Bolk BM. 2017. glmmTMB balances speed and flexibility Among packages for zero-inflated generalized linear Mixed modeling. The R Journal. 9:378–400. doi:https://doi.org/10.32614/RJ-2017-066
- Bushbaum C, Chapman AS, Saier B. 2006. How an introduced seaweed can affect epibiota diversity in different coastal systems. Marine Biology. 148:743–754. doi:https://doi.org/10.1007/s00227-005-0128-9
- Cacabelos E, Olabarria C, Incera M, Troncoso JS. 2010. Effects of habitat structure and tidal height on epifaunal assemblages associated with macroalgae. Estuar Coast Shelf S. 89:43–52. doi:https://doi.org/10.1016/j.ecss.2010.05.012
- Chamberlain AHL, Gorham J, Kane DF, Lewey SA. 1979. Laboratory growth studies on Sargassum muticum (Yendo) fensholt. II. apical dominance. Botanica Marina. 22:11–19.
- Chase JM, Knight TM. 2013. Scale-dependent effect sizes of ecological drivers on biodiversity: why standardised sampling is not enough. Ecology Letters. 16:17–26. doi:https://doi.org/10.1111/ele.12112
- Chemello R, Milazzo M. 2002. Effect of algal architecture on associated fauna: some evidence from phytal molluscs. Marine Biology. 140:981–990. doi:https://doi.org/10.1007/s00227-002-0777-x
- Christie H, Jørgensen NM, Norderhaug KM. 2007. Bushy or smooth, high or low; importance of habitat architecture and vertical position for distribution of fauna on kelp. Journal of Sea Research. 58:198–208. doi:https://doi.org/10.1016/j.seares.2007.03.006
- Christie H, Norderhaug KM, Fredriksen S. 2009. Macrophytes as habitat for fauna. Marine Ecology Progress Series. 396:221–233. doi:https://doi.org/10.3354/meps08351
- Crisp DJG, Williams GB. 1960. Effect of extracts from fucoids in promoting settlement of epiphytic polyzoa. Nature. 188:1206–1207. doi:https://doi.org/10.1038/1881206b0
- De Caceres M, Legendre P. 2009. Associations between species and groups of sites: indices and statistical inference. Ecology. http://sites.google.com/site/miqueldecaceres/.
- Desmond MJ, Suárez-Jiménez R, Nelson WA, Hepburn CD. 2018. Epifaunal community structure within southern New Zealand kelp forests. Marine Ecology Progress Series. 596:71–81. doi:https://doi.org/10.3354/meps12587
- Dixon IMT, Moore PG. 1997. A comparative study on the tubes and feeding behaviour of eight species of corophoid Amphipoda and their bearings on phylogenetic relationships between the corophioidea. Philos Trans R Soc B. 352:93–112. doi:https://doi.org/10.1098/rstb.1997.0006
- Eilertsen M, Norderhaug KM, Sjøtun K. 2011. Does the amphipod fauna associated to epiphytes on kelp (Laminaria hyperborea) change with depth? Mar Biol Res. 7:224–234. doi:https://doi.org/10.1080/17451000.2010.492592
- Enckell PH. 1980. Kräftdjur. Feltfauna. Signum i Lund.
- Engelen AH, Henriques N, Monteiro C, Santos R. 2011. Mesograzers prefer mostly native seaweeds over the invasive brown seaweed Sargassum muticum. Hydrobiologia. 669:157–165. doi:https://doi.org/10.1007/s10750-011-0680-x
- Engelen AH, Primo AL, Cruz T, Santos R. 2013. Faunal differences between the invasive brown macroalga Sargassum muticum and competing native macroalgae. Biological Invasions. 15:171–183. doi:https://doi.org/10.1007/s10530-012-0276-z
- Engelen AH, Serebryakova A, Ang P, Britton-Simmons K, Mineur F, Pedersen MF, Arenas F, Fernández C, Steen H, Svenson R, et al. 2015. Circumglobal invasion by the brown seaweed Sargassum muticum. Oceanogr Mar Biol. 53:81–126.
- Fox J, Weisberg S. 2019. An {R} Companion to applied regression, third edition. Thousand Oaks CA: Sage. https://socialsciences.mcmaster.ca/jfox/Books/Companion/.
- Fredriksen S, Christie H, Sæthre BA. 2005. Species richness in macroalgae and macrofauna assemblages on Fucus serratus L. (Phaeophyceae) and Zostera marina L. (Angiospermae) in Skagerrak, Norway. Mar Biol Res. 1:2–19. doi:https://doi.org/10.1080/17451000510018953
- Gestoso I, Olabarria C, Troncoso JS. 2012. Effects of macroalgal identity on epifaunal assemblages: native species versus the invasive species Sargassum muticum. Helgoland Mar Res. 66:159–166. doi:https://doi.org/10.1007/s10152-011-0257-0
- Guerra-García JM, de Figueroa JM T, Navarro-Barranco C, Ros M, Sánchez-Moyano JE, Moreira J. 2014. Dietary analysis of the marine Amphipoda (Crustacea: Peracarida) from the iberian peninsula. Journal of Sea Research. 85:508–517. doi:https://doi.org/10.1016/j.seares.2013.08.006
- Hartig F. 2020. DHARMa: Residual Diagnostics for Hierarchical (Multi-Level / Mixed) Regression Models. R package version 0.3.3.0. https://CRAN.R-project.org/package=DHARMa.
- Hayward PJ, Ryland JS. 1995. Handbook of the marine fauna of north-west Europe. Oxford: Oxford University Press. 800.
- Heger T, Jeschke JM. 2014. The enemy release hypothesis as a hierarchy of hypotheses. Oikos. 123:741–750. doi:https://doi.org/10.1111/j.1600-0706.2013.01263.x
- Hwang EK, Dring MJ. 2002. Quantitative photoperiodic control of erect thallus production in Sargassum muticum. Botanica Marina. 45:471–475. doi:https://doi.org/10.1515/BOT.2002.049
- Jacobucci GB, Tanaka MO, Leite FPP. 2009. Temporal variation of amphipod assemblages associated with Sargassum filipendula (phaeophyta) and its epiphytes in a subtropical shore. Aquatic Ecology. 43:1031–1040. doi:https://doi.org/10.1007/s10452-009-9230-2
- Lambert WJ. 1991. Coexistence of hydroid eating nudibranchs: do feeding biology and habitat use atter? Biological Bulletin. 181:248–260. doi:https://doi.org/10.2307/1542096
- Lutz M, Minchinton TE, Davis AR. 2019. Differences in architecture between native and non-indigenous macroalgae influence associations with epifauna. Journal of Experimental Marine Biology and Ecology. 514–515:76–86. doi:https://doi.org/10.1016/j.jembe.2019.03.006
- Monteiro C, Engelen AH, Santos R. 2009. Macro- and mesoherbivores prefer native seaweeds over the invasive brown seaweed Sargassum muticum: a potential regulating role on invasions. Marine Biology. 156:2505–2515. doi:https://doi.org/10.1007/s00227-009-1275-1
- Moy FE, Christie H. 2012. Large-scale shift from sugar kelp (saccharina latissima) to ephemeral algae along the south and west coast of Norway. Mar Biol Res. 8:309–321. doi:https://doi.org/10.1080/17451000.2011.637561.
- Norderhaug KM, Christie H, Fosså JH, Fredriksen S. 2005. Fish–macrofauna interactions in a kelp (Laminaria hyperborea) forest. J Mar Biol Assoc UK. 85:1279–1286. doi:https://doi.org/10.1017/S0025315405012439
- O'Dea A, Okamura B. 1999. Influence of seasonal variation in temperature, salinity and food availability on module size and colony growth of the estuarine bryozoan conopeum seurati. Marine Biology. 135:581–588. doi:https://doi.org/10.1007/s002270050659
- Oksanen J, Blanchet FG, Friendly M, Kindt R, Legendre P, McGlinn D, Minchin PR, O'Hara RB, Simpson GL, Solymos P, et al. 2020. vegan: community Ecology package. R Package Version 2. 5–7. https://CRAN.R-project.org/package=vegan.
- Olabarria C, Rossi F, Rodil IF, Quintas P, Troncoso JS. 2009. Use of hierarchical designs to detect scales of heterogeneity in the invasive species Sargassum muticum. Scientia Marina. 73:507–514. doi:https://doi.org/10.3989/scimar.2009.73n3507
- Riisgård HU, Manríquez P. 1997. Filter-feeding in fifteen marine ectoprocts (Bryozoa): particle capture and water pumping. Marine Ecology Progress Series. 154:223–239. doi:https://doi.org/10.3354/meps154223
- Rueness J. 1989. Sargassum muticum and other introduced Japanese macroalgae: biological pollution of European coasts. Marine Pollution Bulletin. 20:173–176. doi:https://doi.org/10.1016/0025-326X(89)90488-8
- Salvaterra T, Green DS, Crowe TP, O’Gorman EJ. 2013. Impacts of the invasive alga Sargassum muticum on ecosystem functioning and food web structure. Biological Invasions. 15:2563–2576. doi:https://doi.org/10.1007/s10530-013-0473-4
- Sars GO. 1895. An account of the Crustacea of Norway. 1. Amphipoda. Cammermeyer. Christiania, København. 711 pp. + 248 plates.
- Sars GO. 1899. An account of the Crustacea of Norway. 2. Isopoda. Bergen Museum. Bergen. 270 pp. + 104 plates.
- Sjøtun K, Eggereide SF, Høisæter T. 2007. Grazer-controlled recruitment of the introduced Sargassum muticum (Phaeophyceae, Fucales) in Northern Europe. Marine Ecology Progress Series. 342:127–138. doi:https://doi.org/10.3354/meps342127
- Sneli JA. 1975. Mollusca Prosobranchia. Universitetsforlaget. 55.
- Sotka EE, Hay ME, Thomas JD. 1999. Host-plant specialization by a non-herbivorous amphipod: advantages for the amphipod and costs for the seaweed. Oecologia. 118:471–482. doi:https://doi.org/10.1007/s004420050750
- Steen H. 1992. Sargassum muticum i Norge: Årssyklus og utbredelse i relasjon til toleranse overfor regulerende miljøfaktorer. MS thesis, University of Oslo.
- Steneck RS, Watling L. 1982. Feeding capabilities and limitation of herbivorous molluscs: A functional group approach. Marine Biology. 68:99–319. doi:https://doi.org/10.1007/BF00409596
- Strong JA, Maggs CA, Johnson MP. 2009. The extent of grazing release from epiphytism for Sargassum muticum (Phaeophyceae) within the invaded range. J Mar Biol Assoc UK. 89:303–314. doi:https://doi.org/10.1017/S0025315408003226
- Suárez-Jiménez R, Hepburn CD, Hyndes GA, McLeod RJ, Taylor RB, Hurd CL. 2017. The invasive kelp Undaria pinnatifida hosts an epifaunal assemblage similar to native seaweeds with comparable morphologies. Marine Ecology Progress Series. 582:45–55. doi:https://doi.org/10.3354/meps12321
- Vandendriessche S, Messiaen M, O’Flynn S, Vincx M, Degraer S. 2007. Hiding and feeding in floating seaweed: floating seaweed clumps as possible refuges or feeding grounds for fishes. Estuar Coast Shelf S. 71:691–703. doi:https://doi.org/10.1016/j.ecss.2006.09.017
- Veiga P, Rubal M, Sousa-Pinto I. 2014. Structural complexity of macroalgae influences epifaunal assemblages associated with native and invasive species. Marine Environmental Research. 101:115–123. doi:https://doi.org/10.1016/j.marenvres.2014.09.007
- Veiga P, Sousa-Pinto I, Rubal M. 2016. Meiofaunal assemblages associated with native and non-indigenous macroalgae. Continental Shelf Research. 123:1–8. doi:https://doi.org/10.1016/j.csr.2016.04.007
- Veiga P, Torres AC, Besteiro C, Rubal M. 2018. Mollusc assemblages associated with invasive and native Sargassum species. Continental Shelf Research. 161:12–19. doi:https://doi.org/10.1016/j.csr.2018.04.011
- Viejo RM. 1999. Mobile epifauna inhabiting the invasive Sargassum muticum and two local seaweeds in northern Spain. Aquatic Botany. 64:131–149. doi:https://doi.org/10.1016/S0304-3770(99)00011-X
- Wernberg T, Thomsen MS, Staehr PA, Pedersen MF. 2004. Epibiota communities of the introduced and indigenous macroalgal relatives Sargassum muticum and Halidrys siliquosa in Limfjorden (Denmark). Helgoland Marine Research. 58:154–161. doi:https://doi.org/10.1007/s10152-004-0180-8
- Wickström SA, Pavia H. 2004. Chemical settlement inhibition versus post-settlement mortality as an explanation for differential fouling of two congeneric seaweeds. Oecologia. 138:223–230. DOI https://doi.org/10.1007/s00442-003-1427-9.
- Williams GB. 1964. The effect of extracts of Fucus serratus in promoting the settlement of larvae of Spirorbis borealis (polychaeta). J Mar Biol Assoc UK. 44:397–414. doi:https://doi.org/10.1017/S0025315400024917