ABSTRACT
We investigated the epibiotic community along Cymodocea nodosa leaves in a seagrass meadow in the Northern Adriatic Sea. In accordance with previous studies, we found that autotrophic organisms dominated throughout the study period (May–October 2014) and showed a clear temporal occurrence pattern with highest occurrence frequencies during summertime. Low relative occurrence of autotrophs was observed in spring, coinciding with higher values for sessile heterotrophs. In both autotrophs and heterotrophs, we observed a general trend towards increased epibiota cover on old leaves and towards the apical (i.e. older) parts of leaves, even though this pattern does not hold true for all species or sampling times. Most heterotrophs were rarely observed, but serpulid polychaetes, the foraminiferan Tretomphaloides concinnus, the hydrozoan Clytia linearis as well as gastropod clutches occurred frequently on all parts of the leaves and showed occurrence patterns putatively reflecting spatial competition with autotrophs and predator avoidance. Hitherto, few data have been available on the epifaunal diversity and community structure on C. nodosa. Thus, this study provides important baseline data for future studies investigating the impact of natural and anthropogenic stressors on epibiota communities associated with this ecologically important Mediterranean seagrass species.
Introduction
Seagrass meadows are among the most productive shallow coastal ecosystems (Duarte and Chiscano Citation1999; Hemminga and Duarte Citation2000) and as ecological engineers (Wright and Jones Citation2006) they provide numerous ecological services to the marine environment (Costanza et al. Citation1997; Duarte Citation2000; Barbera et al. Citation2005; Nakaoka Citation2005; Tuya et al. Citation2014). They increase seascape heterogeneity and consequently the net diversity of species, as the three-dimensional structures of seagrass meadows provide habitat for a great diversity of plants and vagile and sessile animals (Trautman and Borowitzka Citation1999; Hemminga and Duarte Citation2000; Piazzi et al. Citation2016; Hayes et al. Citation2020; Hoffmann et al. Citation2020). In addition, seagrass meadows offer an important source of nutrients, store large quantities of organic carbon (Orth et al. Citation2006) and play an important role as primary producers (Mazzella et al. Citation1993; Duffy Citation2006).
Epibiota initially colonize young leaves and increase their cover and biomass on old ones (Reyes et al. Citation1998; Reyes and Sansón Citation2001). Growth dynamics, associated with certain life strategies, as well as competition among epibiota, determine their success along the leaf surface (Borowitzka et al. Citation1990). However, if the abundance of epibiota is too high, this has a strong negative effect on the photosynthetic capacity, lifespan and growth rate of seagrass leaves (Sand-Jensen Citation1977; Bulthuis and Woelkerling Citation1983; Heijs Citation1985), which forces the release of older leaves (Ott Citation1980) and leads to a clear leaf-age gradient (Heijs Citation1985). Additionally, abiotic factors (e.g. water depth, light levels, seasonality) shape seagrass growth dynamics and meadow structure. With increasing water depth, light availability and, consequently, the photosynthetic capacity of the plants is reduced. On the other hand, growth and long-term survival of the leaves and epibiota is enhanced by reduced mechanical disturbances (Dring and Dring Citation1991; Reyes and Afonso-Carrillo Citation1995; Krause-Jensen et al. Citation2000; Duarte et al. Citation2007; Bračun et al. Citation2020). These complex interactions between biotic and abiotic factors shape a whole community, characterized by quick turnovers, short lifespans and strong adaptability, that is very susceptible to changes in the environment. Therefore, seagrass meadows and their associated flora and fauna represent a promising monitoring tool for detecting coastal environmental changes (Orlando-Bonaca et al. Citation2015; Ben Brahim et al. Citation2020).
In temperate and subtropical climates, such as the Mediterranean Sea, seagrass meadows represent crucial coastal ecosystems and provide important habitats (Meinesz et al. Citation1991; Orth et al. Citation2006; Duarte et al. Citation2008; Tuya et al. Citation2014). Five seagrass species naturally occur in the Mediterranean Sea: Posidonia oceanica (Linnaeus) Delile, 1813, Zostera marina Linnaeus 1753, Zostera noltei Hornemann, 1832, Cymodocea nodosa (Ucria) Ascherson, 1870 and Ruppia maritima Linnaeus, 1753. While P. oceanica is certainly the best studied seagrass species considering its epibiota (e.g. Mazzella et al. Citation1989, Citation1992; Marbá et al. Citation2002; Balata et al. Citation2007; Lepoint et al. Citation2014), less attention has been devoted to the other species (Bračun et al. Citation2020). However, especially C. nodosa and its associated community play an important ecological role, for instance by preparing the soil for the re-establishment of larger seagrass species (Toccaceli Citation1990) and by contributing to the coastal ecosystem functioning (Orlando-Bonaca et al. Citation2015, Citation2016).
Overall, studies of epibiota on C. nodosa are scarce and biased towards autotrophic components (Reyes and Afonso-Carrillo Citation1995; Reyes et al. Citation1998; Reyes and Sansón Citation1996, Citation1997, Citation2001). Hence, little is known about sessile invertebrates inhabiting the leaves (Guidetti et al. Citation2001; Ben Brahim et al. Citation2020; Bračun et al. Citation2020). However, growth patterns of seagrass and epibiota are strongly connected and show interdependencies (Sand-Jensen Citation1977; Bulthuis and Woelkerling Citation1983; Heijs Citation1985; Borowitzka et al. Citation1990). Therefore, studies focusing on a temporal and spatial resolution of epibiota are crucial in order to understand the processes that underlie these dynamics, especially for assessing potential environmental impacts (e.g. Orlando-Bonaca et al. Citation2015).
In a previous study we investigated the temporal growth patterns of C. nodosa in a seagrass meadow in the Northern Adriatic Sea and quantified the diversity of the epibiota on the leaves (Bračun et al. Citation2020). In this preceding study we revealed that the general structure and growth dynamics of the investigated patch are similar to other Mediterranean C. nodosa meadows. Additionally, we showed that overall autotrophs dominated over heterotrophs throughout the study period and at both investigated depths (1.5 and 5 m). Highest abundances of epibiota were observed during summer months and taxa differed among water depths. Additionally, the abundance of epibiota followed a clear leaf-age gradient for autotrophs but not for heterotrophs.
Building upon this, the present study aims to characterize the temporal dynamics of occurrence patterns of epibiota along single C. nodosa leaves. More specifically, we assessed differences in occurrence patterns of epibiota on basal, central and apical leaf segments throughout a period of six months, across two depths and two different leaf-ages, to provide a baseline characterization of this epibiota community on C. nodosa in the Northern Adriatic.
Materials and methods
The investigated Cymodocea nodosa meadow (A) is located in the south-west of the Istrian peninsula (Valsaline/Pula/Croatia – 44°50′59.6″N, 13°50′10.0″E), in the Northern Adriatic Sea. Sampling (by means of snorkelling and scuba diving) was conducted monthly from May–October 2014 at a depth of 1.5 m (shallow) and 5 m (deep). Each monthly sample consisted of four replicates per depth of a standardized area of 0.25 m² where seagrass was collected using a custom-built 0.5 m × 0.5 m frame attached to a mosquito net (Bračun et al. Citation2020). In order to investigate the epibiota on the leaves, 13 shoots from each replicate were harvested in a star-like standardized way within the frame (i.e. 3 + 2 + 3 + 2 + 3 samples per row = 13 shoots × sample-1 × 4 replicates × month-1 × depth-1 = 52 shoots × month-1 × depth-1) (Bračun et al. Citation2020). Leaves in a shoot were classified into three categories (old, young, others; B), and for the present study we only focused on the oldest and youngest leaf of the shoot (C). Determination of leaf-ages followed Reyes and Sansón (Citation2001), with old leaves typically occupying the outer position of a shoot and young leaves originating in between them. Occurrence as well as the position of the epibiota were recorded for each replicate, sample and depth for young (Y) and old (O) leaves. For characterizing differences in epibiota occurrence along seagrass leaves, leaves were subdivided into three segments of the same length (C; apical, central, basal). If possible, epibiota were determined to species level. Otherwise, higher taxonomic ranks were used to classify the specimens (Bračun et al. Citation2020).
Figure 1. Study site, mean monthly (seasonal) variation of shoot structure (leaf length, number of leaves) and investigated leaf segments. (A) Picture of the investigated meadow (Valsaline; Pula; Croatia; 44°50′59.6″N, 13°50′10.0″E) and ‘space competition’ on leaves shown by a sessile Polychaeta, which is overgrown by Corallinaceae. (B) Monthly variation of shoots for the shallow and deep site. Numbers (1–4) indicate other leaves, beside the old (O) and young (Y). (C) One representative shoot (July at 5 m): basal (blue), central (orange), apical (grey).
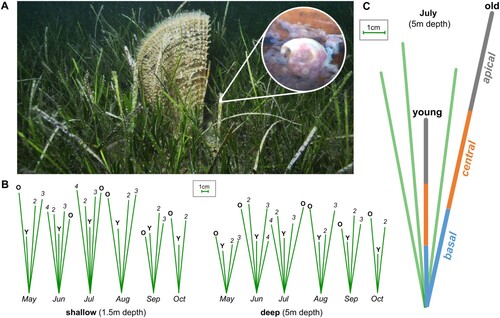
The occurrence frequency was calculated as the count of occurrences per taxonomic unit for each depth, leaf-age and leaf-segment divided by the total number of investigated leaves per month (i.e. Ntotal = 52). To calculate pairwise comparisons of the occurrence frequency between months and the leaf position, a pairwise Wilcoxon rank sum test was applied and P-values were Bonferroni corrected. Kruskal–Wallis tests were used to compare the occurrence frequencies of autotrophs and heterotrophs for different leaf segments, as well as for epibiota inhabiting old and young leaves in different depths. Statistical analyses as well as graphical visualizations were done in R v3.3.2 (R. Core Team Citation2013).
Results
General and temporal growth patterns of epibiota along leaves
In both autotrophs (Kruskal–Wallis, χ2 = 11.007, df = 2, p = 0.004) and heterotrophs (Kruskal–Wallis, χ2 = 7.130, df = 2, P = 0.028), cover along leaves significantly increased from the basal to the apical segment. Pairwise comparisons between different segments revealed significant differences only in the apical vs. basal and the central vs. basal comparison for the autotrophs (Wilcoxon rank sum test with continuity correction; apical vs. central: P = 1; apical vs. basal: P = 0.006; central vs. basal: P = 0.022; Bonferroni corrected) and between the central and basal segment for the heterotrophs (Wilcoxon rank sum test with continuity correction; apical vs. central: P = 1; apical vs. basal: P = 0.111; central vs. basal: P = 0.035; Bonferroni corrected). Additionally, with the exception of old leaves in the shallow transect (Kruskal–Wallis; χ2 = 3.884, df = 2, P = 0.143), a clear differentiation in epibiota occurrence patterns became evident across both depths and leaf-ages (A, A).
Figure 2. (A) Relative occurrence (log-scaled) of autotrophic and heterotrophic epibiota for each segment collected in the shallow transect (1.5 m depth). Relative occurrence in per cent for epiphytes on young (B) and old (C) leaves from apical (grey), to central (orange) and basal (blue) in shallow water. (D) Representative organisms found in the transect. Abbreviations of single taxa are ordered from bottom to top according to relative occurrence values in per cent. Abbreviations: bryozoan (bry), Ceramium (cer), Clytia linearis (cly), Corallinaceae (cor), Kirchenpaueria pinnata (kir), Pachycordyle pusilla (pach), Polychaeta diverse (pol), Rhodophyta diverse (rh), Tretomphaloides concinnus (tre). A detailed view on the relative occurrence of all other epibiota can be found in the Appendix (Figures S1, S3).
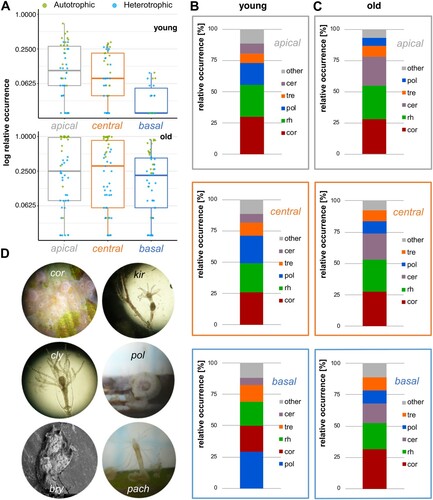
Figure 3. Seasonal occurrence frequency of (A) autotrophic and (B) heterotrophic epiphytes within the apical (grey), central (orange) and basal (blue) segment. Representative taxa for each group are shown in the figure: Ceramium spp. (A) and Clytia linearis (B).
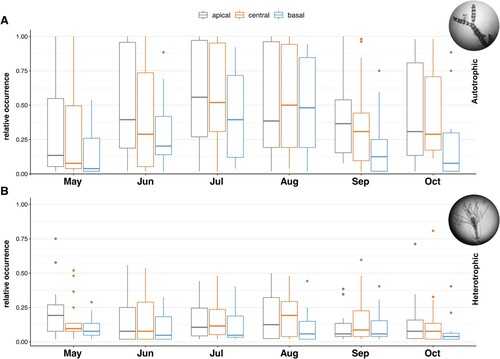
Throughout the study period (May–October 2014), the occurrence of epibiota increased from the basal to the apical segment, but with no significant difference between the central and apical segment (pairwise comparisons Wilcoxon rank sum test with continuity correction; apical vs. central: P = 1; apical vs. basal: P = 0.002; central vs. basal: P = 0.001; Bonferroni corrected). Epibiota reached highest occurrences in July and August on the apical and central segment (A, B). This pattern also holds true when looking at autotrophic and heterotrophic epibiota separately.
Growth patterns and diversity of epibiota in both investigated depths
In shallow water (B, C), young as well as old leaves were dominated by autotrophic Corallinaceae, Ceramium spp., as well as diverse other Rhodophyta and heterotrophs, Polychaeta and the foraminifera Tretomphaloides concinnus (Brady, 1884). Occasionally occurring organisms included gastropod clutches, the hydrozoans Clytia linearis (Thorneley, 1900) and Kirchenpaueria pinnata (Linnaeus, 1758), Bacillariophyceae, various bryozoans, athecate hydrozoans and bivalves (D). Hardly any Oscillatoria spp. (Cyanobacteria) were recorded on young leaves, whereas they were rather common on old leaves. On the young leaves of the shallow transect (B; Figure S1), the relative occurrence of polychaetes and T. concinnus decreased from the basal to the apical segments, whereas Rhodophyta, Corallinaceae and Oscillatoria spp. (on old leaves) increased. Old leaves were colonized by autotrophs such as Corallinaceae, Rhodophyta as well as Ceramium spp. and dominant heterotrophic taxa included the hydrozoans Pachycordyle pusilla (Motz-Kossowska, 1905) as well as C. linearis, polychaetes and the foraminiferan T. concinnus (D). Compared with young leaves, an increase of Ceramium spp. was found on old leaves (C, Figure S2).
Similar results were obtained for deeper water (B,C). On young and old leaves, various autotrophic Rhodophyta (incl. Ceramium spp., Corallinaceae) and heterotrophs such as Polychaeta, the foraminifera T. concinnus as well as gastropod clutches were most prevalent. Occasionally occurring organisms included other foraminifera, mucus cases of vagile polychaetes, several leptothecate and anthothecate hydrozoans (e.g. C. linearis, P. pusilla), various bryozoans (e.g. Schizobrachiella sanguinea) (Norman, 1868) and the anemone Bunodeopsis strumosa Andrès, 1881 (D). On the young leaves (B, Figure S1), the relative occurrence of gastropod clutches decreased towards the apical part whereas Polychaeta and Rhodophyta increased. T. concinnus was distributed evenly along all three segments. Compared with the young leaves, old leaves were dominated by autotrophic cover. On the old leaves (C, Figure S2), Ceramium spp. was quite common in the central and apical segments, but rare in the basal segment. Other autotrophs were generally more common towards the apical end of the leaves. In contrast, gastropod clutches (despite being present in the other segments as well), occurred quite frequently in the basal segment. T. concinnus and Polychaeta were found in all three segments but were generally less common in the basal parts of the leaves. Compared with the young leaves, polychaetes were generally less common on older leaves. In general, for some taxa (e.g. P. pusilla, K. pinnata, Bacillariophyceae) only single or very few individuals were recorded (Figure S1, S2).
Figure 5. (A) Relative occurrence (log-scaled) of autotrophic and heterotrophic epibiota for each segment collected in the deep transect (5 m depth). Relative occurrence in per cent for epiphytes on young (B) and old (C) leaves from apical (grey), to central (orange) and basal (blue) in shallow water. (D) Representative organisms found in the transect. Abbreviations: Athecate Hydrozoan (ATH), Bunodeopsis strumosa (buno), Ceramium (cer), Corallinaceae (cor), Foraminifera (fora), gastropod clutches (ovi), Polychaeta diverse (pol), Schizobrachiella sanguinea (schiz), Tretomphaloides concinnus (tre). A detailed view on the relative occurrence of all other epibiota can be found in the Appendix (Figures S2, S4).
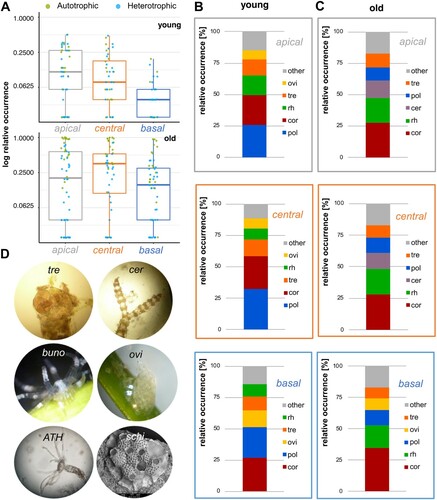
Discussion
Influence of depth and leaf age on epibiota occurrence pattern
Seagrass leaves offer a stable substrate for numerous organisms like algae and sessile invertebrates (Reyes and Sansón Citation2001). These epibiota represent an important component of the community structure of seagrass meadows and are major actors in food webs as well as the overall seagrass ecosystem functioning (Lepoint et al. Citation2014; Hoffmann et al. Citation2020). This is particularly true for C. nodosa that harbours a diverse flora and fauna, despite its relatively small leaf surface area (Mazzella et al. Citation1998; Guidetti et al. Citation2002).
In this study, we quantified the diversity and spatio-temporal occurrence patterns of epibiota along single C. nodosa leaves. Seagrass leaves grow from the base. Thus, the tips represent the oldest parts which are first exposed to and colonized by epibiota. Consequently, initial colonizers are found on young leaves and if they persist and survive, they are pushed ‘conveyer-like’ along the leaf to increasingly light-exposed, apical and thus older parts (Heijs Citation1985). Across the whole study period, epibiotic cover increased during the summertime and from the basal to the apical segment, independent of depth and leaf-age. If conditions are beneficial for autotrophs, they rapidly grow in size, while heterotrophs need time for establishment and are competing with autotrophs for leaf surface area (spatial competition) (Borowitzka et al. Citation1990).
The general trend of temporal changes in occurrence patterns of both autotrophs and heterotrophs in the three leaf segments followed the overall patterns reported by Bračun et al. (Citation2020) for the entire leaves and shoots. Autotrophic organisms dominated throughout the study period and showed a clear temporal occurrence pattern with highest values during summertime, when the light conditions were best for growth and lowest values in spring and autumn (Bračun et al. Citation2020). Low occurrence of autotrophs was observed in May which coincides with higher values for heterotrophs. During springtime, a large amount of nutrients, caused by upwelling effects of nutrient-rich water to upper coastal habitats, is available (Gilmartin et al. Citation1990). Filter-feeding invertebrates grow in size, since a lot of food and leaf area unoccupied by autotrophic epibiota is accessible.
Considering the colonization dynamics along the leaf, autotrophic and heterotrophic organisms follow different trajectories. In general, autotrophs show a much clearer separation between apical (incl. central) and basal leaf segments. In the apical parts of leaves more light for autotrophic organisms is available. This is also true for old leaves, that are, compared with young leaves, less affected by self-shading, due to their relative (i.e. outer) position in the shoot and overall longer leaf lengths and larger surfaces (Stoner Citation1980; Reyes et al. Citation1998). In a previous study on the same C. nodosa patch, growth parameters (e.g. leaf area index, shoot density and leaf length) revealed that old leaves offer one third more area for settlement than young leaves (Bračun et al. Citation2020). Additionally, the leaf area index (LAI) of the shallow transect is much higher (Bračun et al. Citation2020), which could explain the difference in autotroph occurrence patterns between depths. In deeper water light availability for photosynthesis is restricted (Dring and Dring Citation1991; Krause-Jensen et al. Citation2003), which leads to a reduced leaf area and lower growth rates (Bračun et al. Citation2020) of C. nodosa and its epibiota. Interestingly, the higher LAI values at shallow depths do not lead to an increased abundance of heterotrophs, which might be linked to stronger competition due to favourable conditions for autotrophic organisms.
Diversity and distribution of epibiota along leaves of Cymodocea nodosa
In the present study, a total of 18 taxa were identified, six of which were determined to species level (Table SV; Bračun et al. Citation2020). We classified algal epibiota into three main groups: Corallinaceae, represented by crustose algae, Ceramium spp., as erect algae and Rhodophyta (e.g. Reyes and Sansón Citation2001; Ben Brahim et al. Citation2020).
Corallinaceae were the dominant taxon throughout the study period, both depths and leaf-ages, as well as in all three leaf segments. This is in line with previous studies that recorded Rhodophyta as the by far most common phylum (up to 99.2% cover) on seagrass leaves (Reyes and Sansón Citation2001). Among the Rhodophyta, Corallinaceae are known as primary colonizers of seagrass leaves (Borowitzka et al. Citation1990) and considered as very resistant against mechanical disturbances and unfavourable light conditions. Calcareous deposits in the cell walls of Corallinaceae could also prevent or reduce predation pressure by small invertebrates such as isopods compared with other, softer bodied red algae such as Ceramium spp. (Anderson et al. Citation1998).
However, Ceramium spp. occurred in all leaf segments across the whole study period and both depths but reached highest relative occurrences in the shallow transect on old leaves and lowest occurrence frequencies in the deep transect on young leaves, particularly in the basal segments. Hence, if established, a slight increase of the relative occurrence of Ceramium spp. from the basal to the apical segment was detected, suggesting that it is well able to compete with calcareous rhodophytes for space on the leaves. Indeed, young Ceramium spp. develop erect thalli that tower over more planar growing forms such as Corallinaceae, which could lead to a photosynthetic advantage.
Additional autotrophic taxa included Bacillariophyceae (diatoms) and Oscillatoria spp. (Cyanobacteria). Bacillariophyceae live epiphytically, fixed with mucus, on seagrass leaves and occurred only on old leaves. Their absence on young leaves might be explained by self-shading effects due to the central position of the young leaf. Oscillatoria spp. appeared throughout the season, but preferentially in deeper water and on old leaves, and like the autotrophs they were most common towards the apical part of the leaves.
Thus far, most studies were confined to the investigation of algal cover on C. nodosa leaves (e.g. Reyes et al. Citation1998; Reyes and Sansón Citation2001; Mabrouk et al. Citation2014). Very little is known about the epifauna on C. nodosa leaves, but it is very likely a subset of that found on larger and longer-living seagrass species, such as P. oceanica (e.g. Reyes and Sansón Citation2001). A recent study on C. nodosa from Tunisian waters found 12 invertebrate species, including seven bryozoans, two hydrozoans and three annelids (Ben Brahim et al. Citation2020). In our recently published study (Bračun et al. Citation2020), which is also the foundation for this study, we detected three bryozoans (S. sanguinea, Collarina cf. balzaci, Puellina cf. gattyae), three hydrozoans (C. linearis, K. pinnata, P. pusilla), several annelids of the order Sabellida (Janua cf. pagenstecheri, Spirorbis cf. borealis, S. cf. corallinae) and mucus cases of vagile polychaetes. Apart from these, we found molluscs (bivalves and gastropod clutches), Anthozoa (B. strumosa) and foraminifera (T. concinnus, Massilina cf. secans, Peneroplis cf. plan).
A closer look at the distribution of epifauna along leaves revealed Polychaeta, the foraminiferan T. concinnus and gastropod clutches as the most common heterotrophic organisms. In both depths, especially the young leaves were inhabited by sessile tube-building polychaetes of the family Serpulidae. They are easily overgrown by calcareous algae (own observation) and if their aperture is affected, the feeding activity will be reduced or impeded. Therefore, it is advantageous for polychaetes to settle on little-covered fresh leaf parts (young, basal). However, during the lifetime of the leaf they are pushed towards the apical parts and run into risk of being overgrown by dominant algal components that find beneficial conditions in the upper segments (e.g. light availability). Furthermore, quivers or mucus cases of vagile polychaetes, built up from sand, shell detritus, sludge or cement (Brito et al. Citation2005; Westheide and Rieger Citation2013), were rarely found and likely do not influence the overall spatio-temporal growth dynamics of other epibiota.
The foraminiferan T. concinnus was common on all segments of the investigated seagrass leaves. Whereas their smooth umbilical (bottom) side is perfectly adapted for settling on seagrass surface, they are also easily overgrown by the dominant algal community.
Gastropod clutches were found in all segments, in both depths and throughout the season. Snails are common components in seagrass meadows and are known for their grazing activities on seagrass leaves, which also influences the growth of epibiota (Rueda and Salas Citation2007; Bračun et al. Citation2020). No significant temporal difference across the whole study period was observed, but overall, young parts seem to be preferred by the snails. Two reasons could explain this pattern. Young leaves are generally less overgrown than old leaves, which means that more space is available on which to lay eggs. Secondly, young parts (i.e. basal, central in a shoot) are less exposed to putative predators that could feed on the adult snails or the clutches.
Several hydrozoans, including C. linearis, K. pinnata and P. pusilla, were found. Highest occurrences were recorded for C. linearis. This species showed no preference for a particular leaf segment. Usually, reproduction rates of C. linearis are highest during summer and autumn. Their planktonic medusae can drift for several weeks (Lindner and Migotto Citation2002) or, considering the large distribution range, even longer (Lindner et al. Citation2011). In our study, highest relative occurrences were obtained in the months May and October. In previous studies, C. linearis and K. pinnata were documented from different substrates and found to be particularly common on the brown algae Cystoseira spp., but not on C. nodosa (Morri and Bianchi Citation1999). The athecate hydroid, P. pusilla, occurred on old leaves, in all segments, later in the season and especially in deeper water. Other anthoathecate hydroids colonized old leaves, but predominantly the basal part. A theca, which is missing in athecate hydroids, enables the polyp retraction and protects the vulnerable parts of the animals. Hence, anthoathecate hydroids are highly sensitive against mechanical disturbances and predation in more exposed parts of the leaves, which could explain their absence at the apical and central segment.
Scarcely found epibiota included bryozoans (e.g. S. sanguinea), bivalves or anthozoans (e.g. B. strumosa). S. sanguinea is a warm temperature species, often found in the Mediterranean Sea (Hayward and McKinney Citation2002; Mariani et al. Citation2005) and also inhabits P. oceanica (L.) Delile (Balata et al. Citation2007).. Even though larvae of this bryozoan species are efficient swimmers and show a photophilic behaviour (Mariani Citation2002), the single record of this species might have been rather accidental and could be explained by the presence of P. oceanica patches in deeper water close to the investigated C. nodosa site. Bivalves (i.e. putatively juvenile Mytilus edulis Linnaeus, 1758) were found only in June in both depths, on the central (shallow) and apical (deep) segment. Larvae of M. edulis attach preferentially on filamentous algae and until they reach a size of 5 cm a change of substratum is possible (Bayne Citation1964). B. strumosa was recorded only in October, on the basal segment of old leaves in 5 m depth. This is somewhat surprising, as this hemisessile anthozoan is typically found on leaves of Z. noltii, C. nodosa and Ruppia sp. in the summer months (Riemann-Zürneck Citation1998).
Conclusions
This study provides important insights into the spatio-temporal distribution of epibiota organisms along the leaves of C. nodosa. We conclude that the temporal patterns of changes in epibiotic community composition on differently aged leaves are mirrored in the patterns observed along single leaves. Thus, we found an increasing number of taxa and elevated relative occurrences on the older parts of a leaf (old leaves > young leaves, apical > basal segments), a pattern concordant with findings from previous studies that focused on the epiphytic algal communities on C. nodosa and other seagrass species (e.g. Humm Citation1964; Jacobs et al. Citation1983; Heijs Citation1985; Reyes et al. Citation1998). Notably, this general pattern not only holds for the autotrophic, but also for heterotrophic organisms (except for polychaetes or the hydrozoan C. linearis). Only few studies are available that focused not only on the algal epibiota but also on the epifauna associated with seagrass leaves. Thus, our study provides some important baseline data for increasing our understanding of the spatio-temporal structure of epibiota communities along seagrass, and in particular C. nodosa, leaves. Investigating potential changes in community composition and structure in the face of climate change or multiple other anthropogenic stressors with complex impacts on the structure and functioning of seagrass communities could be the key to preserve these important ecosystems and the whole associated flora and fauna (e.g. Grech et al. Citation2011; Siciliano et al. Citation2019; Vieira et al. Citation2020).
Supplemental Material
Download MS Word (817.5 KB)Acknowledgements
For field support and the implementation of the present study we thank the staff of the Morska Škola Pula (Croatia). Furthermore, we thank several colleagues for their input concerning the taxonomic determinations: Neela Enke, Martin Langer, Frans Jorissen, Caterina Morigi, Björn Berning, Robert A. Patzner, Bastian Brenzinger, Kristian Fauchald and Cinzia Gravili. Finally, we thank the Haus des Meeres (Vienna) for supporting S.B. with the Rupert-Riedl prize.
Disclosure statement
No potential conflict of interest was reported by the authors.
Additional information
Funding
References
- Anderson BC, Smit AJ, Bolton JJ. 1998. Differential grazing effects by isopods on Gracilaria gracilis and epiphytic Ceramium diaphanum in suspended raft culture. Aquaculture. 169(1-2):99–109. doi:https://doi.org/10.1016/S0044-8486(98)00322-6
- Balata D, Nesti U, Piazzi L, Cinelli F. 2007. Patterns of spatial variability of seagrass epibiota in the north-west Mediterranean Sea. Marine Biology. 151(6):2025–2035. doi:https://doi.org/10.1007/s00227-006-0559-y
- Barbera C, Tuya F, Boyra A, Sanchez-Jerez P, Blanch I, Haroun RJ. 2005. Spatial variation in the structural parameters of Cymodocea nodosa seagrass meadows in the Canary Islands: a multiscaled approach. Botanica Marina 48(2):122–126. doi:https://doi.org/10.1515/BOT.2005.021
- Bayne BL. 1964. Primary and secondary settlement in Mytilus edulis L. (Mollusca). The Journal of Animal Ecology 33:513–523. doi:https://doi.org/10.2307/2569
- Ben Brahim M, Mabrouk L, Hamza A, Jribi I. 2020. Comparison of spatial scale variability of shoot density and epiphytic leaf assemblages of Halophila stipulacea and Cymodocea nodosa on the Eastern coast of Tunisia. Plant Biosystems-An International Journal Dealing with all Aspects of Plant Biology. 154(3):413–426. doi:https://doi.org/10.1080/11263504.2019.1674399
- Borowitzka MA, Lethbridge RC, Charlton L. 1990. Species richness, spatial distribution and colonisation pattern of algal and invertebrate epibiota on the seagrass Amphibolis griffithii. Marine Ecology Progress Series. Oldendorf. 64(3):281–291. doi:https://doi.org/10.3354/meps064281
- Bračun S, Wagner M, Sefc KM, Koblmüller S. 2020. Temporal growth patterns of Cymodocea nodosa and diversity of its epibiota in the Northern Adriatic Sea. Annales: Series Historia Naturalis. 30(1):69–84. doi:https://doi.org/10.19233/ASHN.2020.10.
- Brito MC, Martin D, Núñez J. 2005. Polychaetes associated to a Cymodocea nodosa meadow in the Canary Islands: assemblage structure, temporal variability and vertical distribution compared to other Mediterranean seagrass meadows. Marine Biology. 146(3):467–481. doi:https://doi.org/10.1007/s00227-004-1460-1
- Bulthuis DA, Woelkerling WJ. 1983. Biomass accumulation and shading effects of epibiota on leaves of the seagrass, Heterozostera tasmanica, in Victoria, Australia. Aquatic Botany. 16(2):137–148. doi:https://doi.org/10.1016/0304-3770(83)90089-X
- Costanza R, d’Arge R, de Groot R, Farber S, Grasso M, Hannon B, Limburg K, Naeem S, O’Neill RV, Paruelo J, et al. 1997. The value of the world’s ecosystem services and natural capital. Nature. 387:253–260. doi:https://doi.org/10.1038/387253a0
- Dring MJ, Dring MH. 1991. The biology of Marine plants. Cambridge, UK: Cambridge University Press.
- Duarte CM. 2000. Marine biodiversity and ecosystem services: an elusive link. Journal of Experimental Marine Biology and Ecology. 250(1):117–131. doi:https://doi.org/10.1016/S0022-0981(00)00194-5
- Duarte CM, Chiscano CL. 1999. Seagrass biomass and production: a reassessment. Aquatic Botany. 65(1):159–174. doi:https://doi.org/10.1016/S0304-3770(99)00038-8
- Duarte CM, Dennison WC, Orth RJ, Carruthers TJ. 2008. The charisma of coastal ecosystems: addressing the imbalance. Estuar Coast. 31:233–238. doi:https://doi.org/10.1007/s12237-008-9038-7
- Duarte CM, Fourqurean JW, Krause-Jensen D, Olesen B. 2007. Dynamics of seagrass stability and change. In: Seagrasses: biology, ecology and conservation. Dordrecht: Springer; p. 271–294. doi:https://doi.org/10.1007/978-1-4020-2983-7_11.
- Duffy JE. 2006. Biodiversity and the functioning of seagrass ecosystems. Marine Ecology Progress Series. 311:233–250. doi:https://doi.org/10.3354/meps311233
- Gilmartin M, Degobbis D, Revelante N, Smodlaka N. 1990. The mechanism controlling plant nutrient concentrations in the northern Adriatic Sea. Internationale Revue der gesamten Hydrobiologie und Hydrographie. 75(4):425–445. doi:https://doi.org/10.1002/iroh.19900750402
- Grech A, Coles R, Marsh H. 2011. A broad-scale assessment of the risk to coastal seagrasses from cumulative threats. Marine Policy. 35(5):560–567. doi:https://doi.org/10.1016/j.marpol.2011.03.003
- Guidetti P, Buia MC, Lorenti M, Scipione MB, Zupo V, Mazzella L. 2001. Temporal trends in the Adriatic seagrass communities of Posidonia oceanica (L.) Delile, Cymodocea nodosa (Ucria) Ascherson, Zostera marina L.: plant phenology, biomass partitioning, elemental composition and faunal features. In: FM Faranda, L Guglielmo and G Spezie, editors. Mediterranean ecosystems. Milano: Springer; p. 289–295. doi:https://doi.org/10.1007/978-88-470-2105-1_37.
- Guidetti P, Lorenti M, Buia MC, Mazzella L. 2002. Temporal dynamics and biomass partitioning in three Adriatic seagrass species: Posidonia oceanica, Cymodocea nodosa, Zostera marina. Marine Ecology. 23(1):51–67. doi:https://doi.org/10.1046/j.1439-0485.2002.02722.x
- Hayes MA, McClure EC, York PH, Jinks KI, Rasheed MA, Sheaves M, Connolly RM. 2020. The differential importance of deep and shallow seagrass to nekton assemblages of the Great Barrier Reef. Diversity. 12(8):292. doi:https://doi.org/10.3390/d12080292
- Hayward PJ, McKinney FK. 2002. Northern Adriatic bryozoa from the vicinity of rovinj, Croatia. Bulletin of the American Museum of Natural History. 270:1–139. doi:https://doi.org/10.1206/0003-0090(2002)270<0001:NABFTV>2.0.CO;2
- Heijs FM. 1985. The temporal distribution and community structure of the epiphytic algae on Thalassia hemprichii (Ehrenb.) Aschers. from Papua New Guinea. Aquatic Botany. 21(4):295–324. doi:https://doi.org/10.1016/0304-3770(85)90074-9
- Hemminga MA, and Duarte CM. 2000. Seagrass ecology. Cambridge, UK: Cambridge University Press. doi:https://doi.org/10.1017/CBO9780511525551
- Hoffmann L, Edwards W, York PH, Rasheed MA. 2020. Richness of primary producers and consumer abundance mediate epiphyte loads in a tropical seagrass system. Diversity. 12(8):384. doi:https://doi.org/10.3390/d12100384
- Humm HA. 1964. Epiphytes of the seagrass, Thalassia hemprichii, in Florida. Bulletin of Marine Science of the Gulf and Caribbean. 14:306–341.
- Jacobs RPWM, Hermelink PM, Van Geel G. 1983. Epiphytic algae on eelgrass at Roscoff, France. Aquatic Botany. 15:157–173. doi:https://doi.org/10.1016/0304-3770(83)90026-8
- Krause-Jensen D, Middelboe AL, Sand-Jensen K, Christensen PB. 2000. Eelgrass, Zostera marina, growth along depth gradients: upper boundaries of the variation as a powerful predictive tool. Oikos. 91:233–244. doi:https://doi.org/10.1034/j.1600-0706.2001.910204.x
- Krause-Jensen D, Pedersen MF, Jensen C. 2003. Regulation of eelgrass (Zostera marina) cover along depth gradients in Danish coastal waters. Estuaries. 26(4):866–877. doi:https://doi.org/10.1007/BF02803345
- Lepoint G, Balancier B, Gobert S. 2014. Seasonal and depth-related biodiversity of leaf epiphytic Cheilostome Bryozoa in a Mediterranean Posidonia oceanica meadow. Cahiers de Biologie Marine 55:57–67.
- Lindner A, Migotto AE. 2002. The life cycle of Clytia linearis and Clytia noliformis: metagenic campanulariids (Cnidaria: Hydrozoa) with contrasting polyp and medusa stages. Journal of the Marine Biological Association of the UK. 82(04):541–553. doi:https://doi.org/10.1017/S0025315402005866
- Lindner A, Govindarajan AF, Migotto AE. 2011. Cryptic species, life cycles, and the phylogeny of Clytia (Cnidaria: Hydrozoa: Campanulariidae). Zootaxa. 2980(1):23–36. doi:https://doi.org/10.11646/zootaxa.2980.1.2
- Mabrouk L, Ben Brahim M, Hamza A, Mahfoudhi M, Bradai MN. 2014. A comparison of abundance and diversity of epiphytic microalgal assemblages on the leaves of the seagrasses Posidonia oceanica (L.) and Cymodocea nodosa (Ucria) Asch in Eastern Tunisia. Journal of Marine Biology 2014:1–10. doi:https://doi.org/10.1155/2014/275305.
- Marbá N, Duarte CM, Holmer M, Martínez R, Basterretxea G, Orfila A, Jordi A, Tintoré J. 2002.; effectiveness of protection of seagrass (Posidonia oceanica) populations in Cabrera National Park (Spain). Environmental Conservation. 29(04):509–518. doi:https://doi.org/10.1017/S037689290200036X
- Mariani S. 2002. Larval supply and recruitment of invertebrates in the western Mediterranean: patterns in contrasting benthic communities [Ph.D. Thesis]. Universitat de Barcelona
- Mariani S, Alcoverro T, Uriz MJ, Turon X. 2005. Early life histories in the bryozoan Schizobrachiella sanguinea: a case study. Marine Biology. 147(3):735–745. doi:https://doi.org/10.1007/s00227-005-1616-7
- Mazzella L, Scipione MB, Buia MC. 1989. Spatio-temporal distribution of algal and animal communities in a Posidonia oceanica Meado. Marine Ecology. 10(2):107–129. doi:https://doi.org/10.1111/j.1439-0485.1989.tb00069.x
- Mazzella L, Buia MC, Gambi MC, Lorenti M, Russo GF, Scipione MB Zupo V. 1992. Plant-animal trophic relationships in the Posidonia oceanica ecosystem of the Mediterranean Sea: A review. Plant-Animal Interactions in the Marine Benthos. In: John D.M. et al. Eds., Systematics Association, Special. Oxford, UK: Clarendon Press, N6, 165–187.
- Mazzella L, Scipione MB, Gambi MC, Buia MC, Lorenti M, Zupo V, Cancemi G. 1993. The Mediterranean seagrass Posidonia oceanica and Cymodocea nodosa. A comparative overview. First International conference on the Mediterranean coastal environment, MEDCOAST. S. 103–116.
- Mazzella L, Guidetti P, Lorenti M, Buia MC, Zupo V, Scipione MB, Rismondo A, Curiel D. 1998. Biomass partitioning in Adriatic seagrass ecosystems (Posidonia oceanica, Cymodocea nodosa, Zostera marina). Rapports et procès-verbaux des réunions Commission internationale pour l'exploration scientifique de la Mer Méditerranée. 35:562–563.
- Meinesz A, Lefevre JR, Astier JM. 1991. Impact of coastal development on the infralittoral zone along the southeastern Mediterranean shore of continental France. Marine Pollution Bulletin. 23:343–347. doi:https://doi.org/10.1016/0025-326X(91)90698-R
- Morri C, Bianchi CN. 1999. Hydroids (Cnidaria: Hydrozoa) from the Aegean Sea, mostly epiphytic on algae. Cahiers de Biologie Marine. 40(3):283.
- Nakaoka M. 2005. Plant–animal interactions in seagrass beds: ongoing and future challenges for understanding population and community dynamics. Population Ecology. 47(3):167–177. doi:https://doi.org/10.1007/s10144-005-0226-z
- Orlando-Bonaca M, Francé J, Mavrič B, Grego M, Lipej L, Flander-Putrle V, Šiško M, Falace A. 2015. A new index (MediSkew) for the assessment of the Cymodocea nodosa (Ucria) Ascherson meadow's status. Marine Environmental Research. 110:132–141. doi:https://doi.org/10.1016/j.marenvres.2015.08.009
- Orlando-Bonaca M, Lipej L, Francé J. 2016. The most suitable time and depth to sample Cymodocea nodosa (Ucria) Ascherson meadows in the shallow coastal area. experiences from the northern Adriatic Sea. Acta Adriatica: International Journal of Marine Sciences. 57(2):251–261. https://hrcak.srce.hr/171641
- Orth RJ, Carruthers TJ, Dennison WC, Duarte CM, Fourqurean JW, Heck KL, Hughes AR, Kendrick GA, Kenworthy WJ, Olyarnik S, et al. 2006. A global crisis for seagrass ecosystems. Bioscience. 56(12):987–996. doi:https://doi.org/10.1641/0006-3568(2006)56[987:AGCFSE]2.0.CO;2
- Ott JA. 1980. Growth and production in Posidonia Oceanica (L.) Delile. Marine Ecology. 1(1):47–64. doi:https://doi.org/10.1111/j.1439-0485.1980.tb00221.x
- Piazzi L, Balata D, Ceccherelli G. 2016. Epiphyte assemblages of the Mediterranean seagrass Posidonia oceanica: an overview. Marine Ecology 37(1):3–41. doi:https://doi.org/10.1111/maec.12331
- R. Core Team. 2013. R: A Language and Environment for Statistical Computing: R Foundation for Statistical Computing. Retrieved from http://www.R- project.org (11.02.2020).
- Reyes J, Afonso-Carrillo J. 1995. Morphology and distribution of nongeniculate coralline algae (Corallinaceae, Rhodophyta) on the leaves of the seagrass Cymodocea nodosa (Cymodoceaceae). Phycologia. 34:179–190. doi:https://doi.org/10.2216/i0031-8884-34-3-179.1
- Reyes J, Sansón M. 1996. Las algas epífitas en Cymodocea nodosa en el Médano, isla de Tenerife (Magnoliophyta, Cymodoceae). Vieraea. 25:45–56.
- Reyes J, Sansón M. 1997. Temporal distribution and reproductive phenology of the epibiota on Cymodocea nodosa leaves in the Canary islands. Botanica Marina. 40:193–202. doi:https://doi.org/10.1515/botm.1997.40.1-6.193
- Reyes J, Sansón M, Afonso-Carrillo J. 1998. Distribution of the epibiota along the leaves of Cymodocea nodosa in the Canary islands. Botanica Marina. 41:543–552. doi:https://doi.org/10.1515/botm.1998.41.1-6.543
- Reyes J, Sansón M. 2001. Biomass and production of the epibiota on the leaves of Cymodocea nodosa in the Canary islands. Botanica Marina. 44(4):307–313. doi:https://doi.org/10.1515/BOT.2001.039
- Riemann-Zürneck K. 1998. How sessile are sea anemones? A review of free-living forms in the Actiniaria Cnidaria: Anthozoa. Marine Ecology. 19(4):247–261. doi:https://doi.org/10.1111/j.1439-0485.1998.tb00466.x
- Rueda JL, Salas C. 2007. Trophic dependence of the emerald neritid Smaragdia viridis (Linnaeus, 1758) on two seagrasses from European coasts. Journal of Molluscan Studies. 73(2):211–214. doi:https://doi.org/10.1093/mollus/eym011
- Sand-Jensen KAJ. 1977. Effect of epibiota on eelgrass photosynthesis. Aquatic Botany. 3:55–63. doi:https://doi.org/10.1016/0304-3770(77)90004-3
- Siciliano A, Schiel DR, Thomsen MS. 2019. Effects of local anthropogenic stressors on a habitat cascade in an estuarine seagrass system. Marine and Freshwater Research. 70(8):1129–1142. doi:https://doi.org/10.1071/MF18414
- Stoner AW. 1980. The role of seagrass biomass in the organization of benthic macrofaunal assemblages. Bulletin of Marine Science. 30(3):537–551.
- Toccaceli M. 1990. Il recif-barriere di Posidonia oceanica della Baia di Carini (Sicilia Nord-Occidentale). Oebalia. 16:781–784.
- Trautman DA, Borowitzka MA. 1999. Distribution of epiphytic organisms on Posidonia autralis and P. sinuosa, two seagrasses with different leaf morphology. Marine Ecology Progress Series. 179:215–229. doi:https://doi.org/10.3354/meps179215
- Tuya F, Ribeiro-Leite L, Arto-Cuesta N, Coca J, Haroun R, Espino F. 2014. Decadal changes in the structure of Cymodocea nodosa seagrass meadows: natural vs. human influences. Estuarine, Coastal and Shelf Science. 137:41–49. doi:https://doi.org/10.1016/j.ecss.2013.11.026
- Vieira R, Martin A, Engelen AH, Thomsen MS, Arenas F. 2020. Interactive effects of co-occurring anthropogenic stressors on the seagrass Zostera noltei. Ecological Indicators. 109:105780. doi:https://doi.org/10.1016/j.ecolind.2019.105780
- Westheide W, Rieger R. 2013. Spezielle Zoologie. Teil 1: Einzeller und Wirbellose Tiere. Berlin, Heidelberg: Springer Spektrum, 3. Auflage.
- Wright JP, Jones CG. 2006. The concept of organisms as ecosystem engineers ten years on: progress, limitations, and challenges. BioScience. 56:203–209. doi:https://doi.org/10.1641/0006-3568(2006)056[0203:TCOOAE]2.0.CO;2