Abstract
The main purpose of this study was to evaluate the efficiency of the alternative quantitative sampling approach and if it was possible, by using this method, to elucidate temporal variations concerning the composition, vertical distribution, and abundance of the motile epifaunal community. The study was performed on a vertical rocky bottom in Gullmarsfjorden on the Swedish west coast. Quantitative sampling was performed at four depths (2, 6, 10 and 15 m) on three occasions (19 June, 23–24 July and 23 September 1996) by means of a water jet powered suction sampler (venturi principle). In total, 53 macrofaunal taxa were found, of which 29 taxa occurred on all sampling dates. Significant vertical zonation patterns were recorded in July and September, but not in June, with amphipods, polychaetes, and echinoderms (mainly Asterias rubens) as the most important contributors to these patterns. A temporal variation in the faunal assemblage also occurred. Species abundance was significantly different between the sampling dates at 2, 6 and 10 m, but not at 15 m. The abundance was higher in July and September than in June. Species with the highest observed differences in abundance were juvenile Asterias rubens, abundant in July, while juvenile shrimp, especially Thoralus cranchii, and some amphipods, especially Microdeutopus spp., increased in abundance in September. This sampling approach was highly effective, and the high abundance and diversity of the motile epifauna indicate that these species play an important role in hard-bottom communities.
Introduction
The coastal benthic zone is a highly productive marine environment. Primary production is considerable and nutrient enrichment originates from terrestrial, pelagic and benthic sources. Rocky shores and bottoms are an important part of this environment and form a very diverse and complex habitat. The hard substrates represent only a minor part (ca. 1%) of all sea bottoms (e.g. Strömberg & Ganning Citation1978), but in certain areas they are widespread and of great importance. Along the northern half of the Swedish west coast, hard bottoms constitute a major part of the shoreline.
The rocky intertidal zone has always caught the attention and interest of scientists, with extensive studies focused on zonation and species interactions (e.g. Colman Citation1933; Dayton Citation1971; Brattström Citation1980, Citation1999; Ricketts et al. Citation1985). Subtidal areas have been difficult to study until the advent of scuba, even though some pioneering work has been carried out (e.g. Gislén Citation1930). However, most studies have only included the sessile fauna (e.g. Svane & Gröndahl Citation1988) or the sessile fauna in interaction with larger motile predators such as asteroids (e.g. Gulliksen & Skjæveland Citation1973) and echinoids (e.g. Karlson Citation1978). The rocky bottom motile epifaunal community has been more or less neglected, probably due to quantitative sampling problems. On the other hand, many studies have been performed on the motile epifauna in seagrass meadows and shallow bays (e.g. Pihl Baden & Pihl Citation1984; Person & Olafsson Citation1986; Edgar & Robertson Citation1992). These areas are very productive and serve as nursery and feeding grounds for fish and motile invertebrates (e.g. Pihl & Rosenberg Citation1982). Rocky bottoms may have a similar function; the epifauna may provide a potentially important trophic link between fish and primary producers, such as the host seaweed with its associated periphyton. Muñoz & Ojeda (Citation1998) also sampled fish in rocky intertidal pools (Chile) and demonstrated how important both sessile and motile macrofauna are as food sources for fish assemblages.
Rocky bottom motile faunal investigations have partly been restricted to those on macroalgae (e.g. Wieser Citation1952; Hagerman Citation1966; Fenwick Citation1976; Edgar Citation1983a; Taylor & Cole Citation1994). A few studies (e.g. Berggren Citation1994) have described basic patterns in the distribution and abundance of the motile fauna, especially concerning vertical and temporal variation.
The objective of the present study was to investigate the abundance, composition and distribution of the motile epifauna in time and space on a rocky subtidal bottom. Emphasis was on the following questions: (1) Is the described venturi type of suction sampler a valuable tool for quantitative sampling on rocky bottoms? (2) Does any vertical zonation occur in the motile epifaunal community on rocky bottoms in Gullmarsfjorden? (3) If there is a zonation pattern, what are the causative factors and what species show a zonation pattern? (4) Is it possible to detect temporal differences in the motile epifaunal community on rocky bottoms by using the described technique?
Materials and methods
Study area
Gullmarsfjorden, with a sill depth of about 40 m and a deep basin of about 119 m, is influenced by three water masses, each with different origin and characteristics (Svansson Citation1984). The upper layer has a salinity of 18–30‰ and is strongly influenced by brackish water from the Baltic Current. This layer has a mean thickness of about 15 m, but varies considerably due to wind and barometric pressure. The intermediate layer consists mainly of water from the Skagerrak and the North Sea. This layer has a salinity of 32–34‰ and is found at a depth of 15–50 m. The deepest water mass, with a salinity of approximately 34.5‰, is of Atlantic origin (Svansson Citation1984; Svansson & Hognestad Citation1985).
The study was performed at Gåsklåvan (58°18.6′N 11°32.5′E), a semi-sheltered area in the central part of the fjord, with a more or less vertical rock wall extending to a depth of about 70 m ().
Figure 1. Location of the sampling site in Gullmarsfjorden on the Swedish west coast. The dotted line represents the 100 m isobath. KMRS=Kristineberg Marine Research Station.
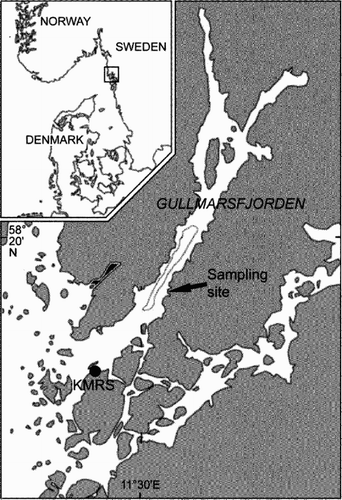
During the study period, the algal density was high in the upper zone of the rock wall, but decreased gradually with depth. Laminaria, and more finely structured species, e.g. Ceramium, occurred from the surface to approximately 3 m depth. Filamentous green and red algae dominated at about 6 m depth. Below 10 m depth the vegetation was very sparse.
Sampling procedures
The motile macrofauna was sampled during daytime on 19 June, 23–24 July and 23 September 1996. On each occasion, four sites of vertical transects were randomly chosen within a 30 m fixed horizontal section along the rock wall. Samples were collected at 2, 6, 10 and 15 m depth along each transect. In total, 48 samples were collected during the study period. Due to failure of the sampling gear, one of the transects from 19 June had to be excluded.
The fauna was sampled quantitatively with a “water jet” powered suction sampler. The suction was induced by means of the venturi principle and the sampler operated efficiently over the whole depth scale. The venturi suction force is primarily created by introducing a high flow of water at an angle (in this case by means of an attached PVC pipe) into a larger diameter pipe. This creates a suction force on one end of the large pipe, where a sampling hose is attached, while a collecting device (net or sieve) is attached at the other end. The sampling device was powered by a high-pressure water pump (Honda WH 15X) that delivered 400 l min−1, on board a small boat. A fine-meshed plankton net was attached to the water intake to prevent collection of organisms from the water column. The material was collected by sampling inside a PVC cylinder enclosing an area of 0.1 m2. The top of the cylinder had a ca. 10 cm wide hole centrally located. It was covered by a flexible rubber sealant with a 5 cm diameter hole. The sampling pipe was placed through this hole to sample the 0.1 m2 area. It was possible to observe the sampling area through two Plexiglass windows attached to the upper end of the cylinder. An underwater flashlight was also attached on the inside of the cylinder to illuminate the sampling area.
During sampling, the cylinder was placed against the rock wall and the sampling pipe was repeatedly swept over the entire surface. Each area was sampled for 3 min and all the material was collected into a 1 mm sieve. To prevent fragile animals from being damaged, the sieve was partially submerged in seawater.
All samples were preserved in 4% buffered formaldehyde. The animals were sorted under a dissecting microscope, identified to the lowest possible taxon, and the number of each taxon was recorded.
The efficiency of the suction sampler was tested on 23 September 1996. Regular sampling was performed at 6 m depth for 3 min. The sampling cylinder was kept at the same spot, which was then sampled for an additional 3 min with the material collected into another sieve.
The software Statecol (Ludwig & Reynolds Citation1988) was used for the descriptive statistics. Diversity was expressed by using the Shannon–Wiener index and evenness was calculated using Hill's modified ratio E5 (Alatalo Citation1981). Species richness was expressed as the average number of species per 0.1 m2 on each sampling occasion and at each depth (in June n=3, in July and September n=4).
Multivariate techniques were used to examine similarities and dissimilarities between samples with the software package PRIMER 4.0 (Clarke & Warwick Citation1994; Carr Citation1996). Abundance data were fourth root transformed in order to decrease the influence of dominating species (Field et al. Citation1982) and a similarity matrix involving all species was constructed using the Bray–Curtis index. This matrix was used for non-metric multidimensional scaling (MDS) analyses. Analysis of similarity (ANOSIM) was used to calculate significance levels, and similarity percentages (SIMPER) were used to determine which species were responsible for the observed differences.
Results
The sampling efficiency by this device was very high, indicated by the fact that almost all individuals were collected during the first 3 min of sampling (). After the first 3 min, 377 individuals (24 species) were recorded and seven individuals (two species) were recorded after the additional 3 min period. No new species were recorded after the additional 3 min and 98.2% of the individuals were collected during the first 3 min.
Table I. Efficiency of the suction sampler. The number of taxa after an ordinary sampling and after an additional 3 min sampling at the same spot.
In total, 53 taxa were recorded during the entire sampling period, of which 29 were present on all sampling dates. Thirty-six taxa were found in June, 42 in July and 44 in September ().
Table II. Taxa found on the three sampling occasions.
Molluscs dominated in June (), particularly the gastropod Bittium reticulatum (daCosta), at all depths, except at 2 m where crustaceans (mainly the amphipod Corophium insidiosum Crawford) were the most abundant group. Polychaetes and echinoderms were also found, but in lower numbers. In June, the abundance was comparatively low among all groups at 15 m.
Figure 2. Mean abundance of taxonomic groups, expressed as individuals per 0.1 m2. The error bars represent+standard error values.
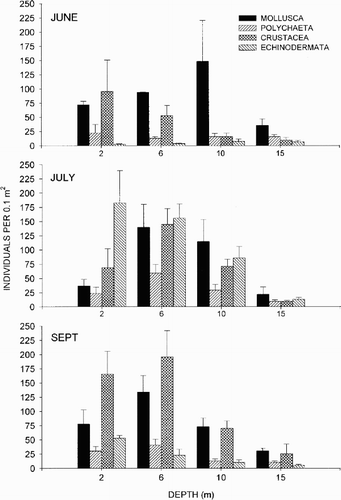
The abundance was generally higher in July than in June (especially at 2 and 6 m), with an increase in echinoderms (mainly juvenile Asterias rubens), crustaceans, and polychaetes. However, the overall abundance at 15 m was still low.
The total abundance was slightly lower in September than in July, but with no significant change at 15 m. The echinoderms decreased between July and September at all depths except at 15 m, while the crustaceans increased in abundance at 2 and 6 m.
The Shannon–Wiener diversity index () indicated that diversity increased during the sampling period at 2 and 6 m and reached its maximum in September. At 10 and 15 m the difference in diversity was less pronounced between sampling dates. Diversity increased with depth in June, but decreased with depth in September. In July, diversity was relatively constant with depth, except at 2 m where the value was very low.
Figure 3. a) Diversity (Shannon-Wiener index), b) evenness expressed by Hill's modified ratio E5, and c) species richness as average number of species per 0.1 m2. (In June n=3, in July and September n=4).
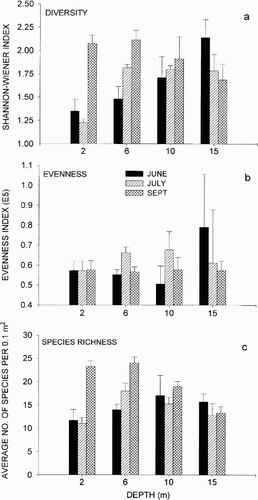
Evenness () was stable at 2 m, but varied increasingly with depth. The difference between the replicates was also very high at 15 m in June and July. Species richness increased during the sampling period at 2 and 6 m (), while the temporal variation was low at 10 and 15 m.
The MDS ordinations to elucidate possible zonation on the different sampling occasions all had stress values for the two-dimensional solutions low enough for a satisfactory representation ().
Figure 4. Multi dimensional scaling (MDS) of the zonation pattern in June, July and September. Each point represents one replicate and one depth.
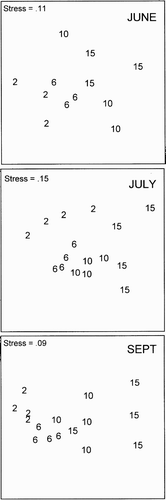
In June, pairwise tests in ANOSIM showed no significant differences between the four depths, but a tendency towards zonation could be detected between the two shallower and the two deeper levels. However, in July there was a significant difference (P<0.03) between all depth intervals, except between 10 and 15 m. The same pattern was recorded in September, all depth intervals were clearly separated (P<0.03), except 10 and 15 m. Thus, a vertical zonation did develop in the motile epifaunal community during the summer and it was clearly discernible in July and September, but there was no distinct separation between the two deeper levels.
In July, the high abundance at 6 m contributed greatly to the average dissimilarities between depths. Species that contributed the most (>6%) to the differences were the amphipods Microdeutopus propinquus Footnote* G. O. Sars, M. gryllotalpa daCosta, and C. insidiosum, the echinoderm A. rubens, and the polychaetes Kefersteinia cirrata (Keferstein) and Phyllodoce maculata Fauvel ().
Table III. SIMPER results: the three species that contributed the most (as a percentage) to the total dissimilarity between depths where significant (<0.05) differences occurred. Species depth preference (highest abundance) is also presented.
In September, the differences between depths were mostly impacted by abundances at the shallow depths. Species that had the highest impact (>6%) on the observed differences were M. anomalus* (Rathke) and M. gryllotalpa ().
The MDS plots concerning temporal variation at all depths () all had low stress values for the two-dimensional solutions, indicating a satisfactory representation. All replicates were grouped together at all depths, except at 15 m, and according to the ANOSIM, all the groups at 2, 6 and 10 m were significantly (P<0.03) separated from each other, indicating that the community underwent a significant temporal change. At the deepest level, the change over time was less obvious, but there was a significant (P<0.03) difference between the first (June) and the last (September) sampling occasion.
Figure 5. Multi dimensional scaling (MDS) ordination of temporal variation. Each point represents one replicate and one sampling occasion at the different depths.
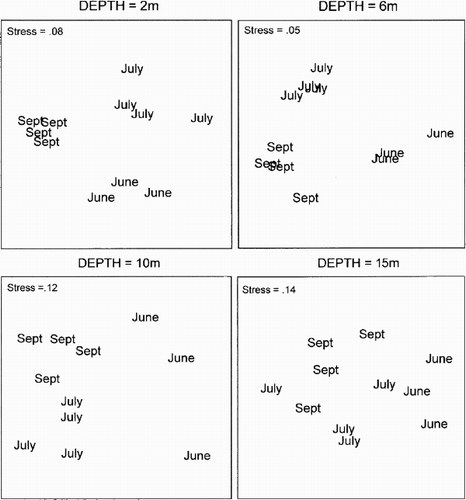
In general, the abundance was higher in July and September than in June, which was caused by an increase in the number of both individuals and species. The starfish A. rubens contributed significantly to the temporal difference and so did the shrimp Thoralus cranchii (Leach). The polychaete P. maculata and the amphipod M. propinquus occurred mainly in July and September. Microdeutopus gryllotalpa and M. anomalus were found on each sampling date, but dominated in September ().
Discussion
The suction sampler presented here, collecting 98.2% of all individuals within 3 min, proved to be highly efficient and reliable. The suction force was also significant, which probably made it possible to sample individuals hiding in crevices and among dense algae, in contrast to sampling by means of, e.g. dip nets and dredges. However, the study was only performed during daylight hours and over a limited period of time. Nocturnal migration among epifauna has also been reported, and night sampling would probably suggest different zonation patterns. Howard (Citation1987) showed that many seagrass-inhabiting crustaceans perform diel migration between the sediment surface and the seagrass canopy. Other studies (e.g. Robertson & Howard Citation1978) also indicate that amphipods, isopods, and polychaetes associated with seagrass beds spend some time in the water column at night.
The results from our study showed that vertical zonation was hard to detect in June, even though a tendency towards zonation between the two shallower and the two deeper strata could be detected, whereas there was a clear zonation pattern in July and September.
Zonation may be caused by a number of factors, one of the most important is probably substrate. Many animals are associated with specific algae. A zonation pattern among algae would most likely reflect a zonation pattern in the motile fauna (e.g. Berggren Citation1993, Citation1994). Laboratory experiments have also indicated that the distribution of phytal amphipods results from both predation and algal selection (Edgar Citation1983b). The morphology of the algae may affect the habitat selection of animals; plants with a fine structure may provide more food in the form of periphyton because of the greater surface area per wet weight and better refuge from predation (Taylor & Cole Citation1994). Many amphipods use plants as an attachment point to avoid being dislodged by water movement. Structural complex plants increase the opportunities for attachment (Johnson & Scheibling Citation1987). Algae with wide thalli (e.g. Laminaria and Ulva) occurred more numerously at 2 m depth than at other depths within the study area. These algae are neither easy to cling to, nor do they provide good shelter against predators. These factors may have contributed to the high abundances that were recorded at 6 m, where more finely structured algae dominated.
According to Edgar (Citation1983a), changes in the phytal fauna with depth are probably also related to differences in water movement. For example, suspension-feeding amphipods (e.g. C. insidiosum) are more abundant where water currents are stronger (Fenwick Citation1976), and concentrations of suspended material are higher (Sheader Citation1978), i.e. in the upper zone. Krapp-Schickel (Citation1993) has described how water movement is a very important factor regulating the zonation patterns of amphipods. For example, species inhabiting the splash zone are excellent swimmers, while the conditions on deep water favours tube-building species. The more pronounced settling of particles in the lower zone allows for increased numbers of detritivores, e.g. B. reticulatum. According to Graham (Citation1988), this gastropod feeds primarily on detritus. However, in this study, B. reticulatum was found to be most abundant at 2 and 6 m depths, most likely more exposed to wave action and therefore with low detritus accumulation.
The recruitment of animals to certain depths may contribute to the zonation patterns as well. Development in almost all amphipods is direct and the juveniles are usually released directly into the same environment as the parent generation (e.g. Steele & Steele Citation1991). This may contribute to continuous zonation if the parents are limited to certain areas. The recruitment of animals with planktonic larvae to different depths may be due to larval response to, e.g. photo- and geotaxis. Juvenile A. rubens occurred mainly at shallow sites. They feed carnivorously immediately after completion of metamorphosis (Barker & Nichols Citation1983). The small starfish feeds primarily on young blue mussels, Mytilus edulis L. (Mortensen Citation1924), which are abundant among algae and hydroids in shallow areas (Jensen & Spärck Citation1934).
The more diffuse zonation patterns at 10 and 15 m depth may be due to a more uniform environment, which does not stimulate zonation. The substrate at the two deep levels differed from the two shallow ones. Below 10 m the bottom became more homogeneous with respect to the composition and density of both sessile fauna and algae. Very few algae were recorded at 10 and 15 m.
The thermo- and haloclines are generally found between 10 and 15 m, and variations in salinity and temperature are less pronounced below this level, which may lead to a more stable environment (Svansson Citation1984; Svansson & Hognestad Citation1985). Wave action was not a factor on deeper water, contributing to more stable conditions.
The reason why the zonation pattern was more conspicuous later in the study period may be explained by the fact that the community probably had time for development. Many species that recruited early during the study period had a limited vertical distribution. When their abundance increased, the zonation of the total fauna became more prominent.
The epifaunal community changed with time at all depths, but at 15 m the variation was limited. A significant difference was only recorded at this depth between June and September. Both Shannon–Wiener diversity and species richness increased clearly with time at the two shallow depths (2 and 6 m), while evenness values remained rather constant. This indicates that the ratio between the number of species and individuals did not change considerably. One major reason for these changes when comparing June and July was the increased number of juvenile starfish, A. rubens. Juveniles also occurred in September but in lower numbers. They were most abundant at 2 and 6 m, but also occurred sparsely at deeper levels. Barker & Nichols (Citation1983) found that juvenile A. rubens settled in late June or early July and that small numbers of starfish continued to settle in August and September. The decrease in number of starfish in September was probably due to juvenile mortality and, to some extent, migration out of the sampling area.
Crustaceans showed a clear temporal change. Juvenile shrimp, T. cranchii and Athanas nitescens (Leach), were fairly numerous in September. These were also more common at shallow depths. According to Berggren (Citation1994) both T. cranchii and A. nitescens are mostly found in shallow water (<8 m). The abundance and number of species of the amphipods also increased during the study period with a peak in September, except C. insidiosum, which was rather stable. Other studies (e.g. Hagerman Citation1966) have reported a rather stable occurrence of this species throughout the year. Mature females with eggs were observed on all sampling occasions, and according to Sheader (Citation1978), C. insidiosum breed throughout the year except in November and December.
Opisthobranchs such as Onchidoris muricata (Müller) and Elysia viridis (Montagu) also contributed to the temporal variation as they occurred only in September.
No significant temporal community change was recorded at 15 m. Diversity, evenness and species richness showed rather consistent values at this depth during the sampling period. As discussed earlier, the community at the deepest level was most likely exposed to a more stable environment. Temporal variation was mainly caused by the recruitment of individuals of already existing species and of the occurrence of new species.
Editorial responsibility: Christoffer Schander
Acknowledgments
This paper is Smithsonian Marine Station at Fort Pierce contribution number 607. The authors would like to thank Jarl-Ove Strömberg for his valuable support, advice, and critical review of the manuscript. We are grateful to Peter Adolfsson and Rocío Fernández for invaluable help during the field work, and to Sherry Reed for a critical review of the revised manuscript. We thank Stefan Agrenius for valuable help with the species identification. Thanks are also due to Michelle Kush for linguistic corrections.
Notes
Published in collaboration with the University of Bergen and the Institute of Marine Research, Norway, and the Marine Biological Laboratory, University of Copenhagen, Denmark
Taxonomical remark: there exists some uncertainty whether the amphipods Microdeutopus anomalus and M. propinquus are separate species or if they should be regarded as one species. Sars (Citation1890) treated them, with some hesitation, as two species, as did Oldevig (Citation1933) and Dahl (Citation1948). Myers (Citation1969) and Hansson (Citation1994) dealt with them as synonyms, while Enequist (Citation1949) did not express a definite opinion. Some consistent differences in character were observed from the material of this study and they were therefore treated as two species here. Adult females with eggs (from both species) have occurred at the same time and have shown a distinct difference in size and in the number of joints on the accessory flagellum. The collected males also differed in size, in the appearance of the anterior gnathopod and in the number of joints on the accessory flagellum.
References
References
- Alatalo , RV . 1981 . Problems in the measurement of evenness in ecology . Oikos , 37 : 199 – 204 .
- Barker , MF and Nichols , D . 1983 . Reproduction, recruitment and juvenile ecology of the starfish, Asterias rubens and Marthasterias glacialis . Journal of the Marine Biological Association of the United Kingdom , 63 (4) : 745 – 65 .
- Berggren , M . 1993 . Aquarium experiments on habitat choice of littoral shrimps of the Faroe Islands . Crustaceana , 65 : 129 – 43 .
- Berggren M 1994 The shrimps of the algal zone in the Gullmar Fjord area, west coast of Sweden Habitat choice of benthic shrimps PhD thesis University of Göteborg
- Brattström , H . 1980 . Rocky-shore zonation in the Santa Marta area, Colombia . Sarsia , 65 : 163 – 226 .
- Brattström , H . 1999 . Marine biological investigations in the Bahamas. 23. Description of the littoral zonation at nine Bahamian rocky-shore localities . Sarsia , 84 : 319 – 65 .
- Carr MR 1996 PRIMER User Manual (Plymouth Routines in Multivariate Ecological Research) Plymouth Marine Laboratory
- Clarke KR Warwick RM 1994 Change in Marine Communities: an Approach to Statistical Analysis and Interpretation Natural Environment Research Council
- Colman , J . 1933 . The nature of the intertidal zonation of plants and animals . Journal of the Marine Biological Association of the United Kingdom , 18 : 435 – 76 .
- Dahl E 1948 On the Smaller Arthropoda of Marine Algae, Especially in the Polyhaline Waters off the Swedish West Coast Undersökningar över Öresund 35 Lund C. W. K. Gleerup
- Dayton , PK . 1971 . Competition, disturbance, and community organization: the provision and subsequent utilization of space in a rocky intertidal community . Ecological Monographs , 41 (4) : 351 – 89 .
- Edgar , GJ . 1983a . The ecology of south-east Tasmanian phytal animal communities. I. Spatial organization on a local scale . Journal of Experimental Marine Biology and Ecology , 70 : 129 – 57 .
- Edgar , GJ . 1983b . The ecology of south-east Tasmanian phytal animal communities. IV. Factors affecting the distribution of ampithoid amphipods among algae . Journal of Experimental Marine Biology and Ecology , 70 : 205 – 25 .
- Edgar , GJ and Robertson , AI . 1992 . The influence of seagrass structure on the distribution and abundance of mobile epifauna: pattern and process in a Western Australian Amphibolis bed . Journal of Experimental Marine Biology and Ecology , 160 : 13 – 31 .
- Enequist , P . 1949 . Studies on the soft-bottom amphipods of the Skagerak . Zoologiska Bidrag från Uppsala , 28 : 299 – 492 .
- Fenwick , GD . 1976 . The effect of wave exposure on the amphipod fauna of the alga Caulerpa brownii . Journal of Experimental Marine Biology and Ecology , 25 : 1 – 18 .
- Field , JG , Clarke , KR and Warwick , RM . 1982 . A practical strategy for analysing multispecies distribution pattern . Marine Ecology Progress Series , 8 : 37 – 52 .
- Gislén , T . 1930 . Epibioses of the Gullmar Fjord II. Kristinebergs Zoologiska Station 1877–1927. . Skriftserie, Kungliga svenska vetenskapsakademien , 4 : 1 – 380 .
- Graham A 1988 Molluscs: Prosobranch and Pyramidellid Gastropods Synopses of the British Fauna, No. 2 Leiden E.J. Brill/Dr. W. Backhuys
- Gulliksen , B and Skjæveland , SH . 1973 . The sea-star, Asterias rubens L., as predator on the ascidian, Ciona intestinalis (L.), in Borgenfjorden, North-Tröndelag, Norway . Sarsia , 52 : 15 – 20 .
- Hagerman , L . 1966 . The macro- and microfauna associated with Fucus serratus L., with some ecological remarks . Ophelia , 3 : 1 – 43 .
- Hansson HG 1994 Sydskandinaviska marina flercelliga evertebrater Länsstyrelsen i Göteborgs och Bohus län, Miljöenheten [in Swedish]
- Howard , RK . 1987 . Diel variation in the abundance of epifauna associated with seagrasses of the Indian River, Florida, USA . Marine Biology , 96 : 137 – 42 .
- Jensen ADS Spärck R 1934 Bløddyr II. Saltvandsmuslinger. Danmarks Fauna 40 København G. E. C. Gads
- Johnson , SC and Scheibling , RE . 1987 . Structure and dynamics of epifaunal assemblages on intertidal macroalgae Ascophyllum nodosum and Fucus vesiculosus in Nova Scotia, Canada . Marine Ecology Progress Series , 37 : 209 – 27 .
- Karlson , R . 1978 . Predation and space utilization patterns in a marine epifaunal community . Journal of Experimental Marine Biology and Ecology , 31 : 225 – 39 .
- Krapp-Schickel , G . 1993 . Do algal-dwelling amphipods react to the “critical zones” of a coastal slope? . Journal of Natural History , 27 : 883 – 900 .
- Ludwig JA Reynolds JF 1988 Statistical Ecology A Primer on Methods and Computing New York John Wiley & Sons
- Mortensen TH 1924 Pighude (Echinodermer). Danmarks Fauna 27 København G.E.C. Gads
- Muñoz , AA and Ojeda , FP . 1998 . Guild structure of carnivorous intertidal fishes of the Chilean coast: implications of ontogenetic dietary shifts . Oecologia , 114 : 563 – 73 .
- Myers , AA . 1969 . A revision of the amphipod genus Microdeutopus Costa (Gammaridea: Aoridae) . Bulletin of the British Museum (Natural History) , 17 (4) : 93 – 148 .
- Oldevig H 1933 Sveriges amphipoder Göteborg Wettergren & Kerber [in Swedish]
- Persson , LE and Olafsson , EB . 1986 . Distribution and abundance of mobile epifauna and macrozoobenthos in south Swedish shallow marine areas . Ophelia , suppl. 4 : 201 – 10 .
- Pihl , L and Rosenberg , R . 1982 . Production, abundance, and biomass of mobile epibenthic marine fauna in shallow waters, western Sweden . Journal of Experimental Marine Biology and Ecology , 57 : 273 – 301 .
- Pihl Baden , S and Pihl , L . 1984 . Abundance, biomass and production of mobile epibenthic fauna in Zostera marina (L.) meadows, western Sweden . Ophelia , 23 (1) : 65 – 90 .
- Ricketts EF Calvin J Hedgpeth JW Philips DW 1985 Between Pacific Tides Stanford CA Stanford University Press
- Robertson , AI and Howard , RK . 1978 . Diel trophic interactions between vertically-migrating zooplankton and their fish predators in an eelgrass community . Marine Biology , 48 : 207 – 13 .
- Sars GO 1890 An Account of the Crustacea of Norway, Vol. 1, Amphipoda Copenhagen A.L.B. Cammermeyers
- Sheader , M . 1978 . Distribution and reproductive biology of Corophium insidiosum (Amphipoda) on the north-east coast of England . Journal of the Marine Biological Association of the United Kingdom , 58 : 585 – 96 .
- Steele DH Steele VJ 1991 Morphological and environmental restraints on egg production in amphipods In: Wenner A, Kuris A, editors Crustacean Issues 7, Crustacean Egg Production Rotterdam A.A. Balkema
- Strömberg JO Ganning B 1978 Marin ekologi In: Marin ekologi, sedimentologi och marina miljöföroreningar Delrapport till Havet-Naturförhållanden och utnyttjande (FRP, no 7) [in Swedish]
- Svane , I and Gröndahl , F . 1988 . Epibioses of Gullmarsfjorden: an underwater stereophotographical transect analysis in comparison with the investigations of Gislén in 1926–29 . Ophelia , 28 (2) : 95 – 110 .
- Svansson A 1984 Hydrography of the Gullmar Fjord Meddelande från Havsfiskelaboratoriet, Lysekil 297
- Svansson , A and Hognestad , P . 1985 . Hydrography of the Kattegat and the Skagerrak area 1984. Meddelande från Havsfiskelaboratoriet . Lysekil , 314 : 7 – 12 .
- Taylor , RB and Cole , RG . 1994 . Mobile epifauna on subtidal brown seaweeds in northeastern New Zealand . Marine Ecology Progress Series , 115 : 271 – 82 .
- Wieser , W . 1952 . Investigations on the microfauna inhabiting seaweeds on rocky coasts . Journal of the Marine Biological Association of the United Kingdom , 31 : 145 – 74 .