Abstract
The influence of benthic viruses for bacterial mortality and carbon recycling was investigated in continental margin sediments off central Chile. Virus net production correlated significantly with the total benthic mineralization rate (r2=0.94, n = 5) across a coastal–shelf transect. Within anoxic bag incubations, viral production also correlated significantly with bacterial activity (r2=0.86, n = 7). The coupling between virus production and independent measurements of bacterial mineralization strongly suggested that benthic viral production was regulated by bacterial metabolic activity. From the rates of viral and bacterial production [2–6×106 virus-like particles (VLP) cm−3 h−1 and 2–7×105 cells cm−3 h−1, respectively], the potential impact of viruses on bacterial mortality and dissolved organic carbon production was estimated. Viral-induced mortality corresponded to 44–138% of bacterial net production, indicating that viruses had substantial impact on bacterial mortality. The estimated release of viral lysates of 0.3–3.5 nmol C cm−3 h−1, however, contributed insignificantly to bacterial carbon respiration (<8%). Compiling data for various sediments using the same incubation approach confirmed that (1) viral activity is coupled to benthic mineralization rates, (2) virus-induced mortality constitutes a significant loss factor for benthic bacteria, whereas (3) viral-mediated recycling of organic carbon plays a minor role in benthic environments.
Published in collaboration with the University of Bergen and the Institute of Marine Research, Norway, and the Marine Biological Laboratory, University of Copenhagen, Denmark
Introduction
It is now well established that viruses contribute significantly to microbial mortality in pelagic environments, with large implications for microbial population dynamics and pelagic carbon cycling [recently reviewed in Weinbauer (Citation2004) and Suttle (Citation2005)]. Because viral abundances in sediments are generally 10–100-fold higher than in the overlying water column, it has been speculated that viruses also have significant impact on the microbial ecology and carbon turnover in sediments. Research in benthic viruses has, therefore, received increasing focus during the last few years (e.g. Danovaro & Serresi Citation2000; Bird et al. Citation2001; Hewson et al. Citation2001; Middelboe et al. Citation2003a; Danovaro et al. Citation2005). The density of benthic viruses seems to correlate with the trophic level of the ecosystem, ranging from 108–109 virus-like particles (VLP) cm−3 in coastal, eutrophic sediments, to about 107 VLP cm−3 in deeper or more oligotrophic areas (e.g. Hewson et al. Citation2001). The high benthic VLP abundances may partly reflect a continuous supply via sedimenting particles (Hewson et al. Citation2001). However, it still needs to be investigated to what extent these VLP contribute to the dynamic, active pool of VLP that has recently been demonstrated in sediments (e.g. Fischer et al. Citation2003; Glud & Middelboe Citation2004) or merely to an inactive fraction of decaying VLP.
Benthic carbon mineralization proceeds via a complex network of microbial-driven interrelated biogeochemical processes. To the extent that benthic viruses are important for bacterial mortality, they could regulate and modify the activity and structure of the microbial communities, and thereby influence the fate of organic matter reaching the seafloor. However, little is known about the role of viruses for benthic microbial activity, and even basic knowledge of the virus production rates or factors regulating their activity is lacking.
The few measurements on benthic VLP production that have been obtained cover a wide range of production rates from 106 to 108 VLP cm−3 h−1, corresponding to turnover times of the total viral community from a few hours to several days (Hewson & Fuhrman Citation2003; Fischer et al. Citation2003; Mei & Danovaro Citation2004; Glud & Middelboe Citation2004). Most of these studies have been carried out at single locations, and the values have been based on different methodological approaches, hampering any direct comparisons. At present there exist no data on the spatial or regional variations in viral production rate applying comparable methods. Such studies are important in order to establish general concepts for the distribution and production of viruses and their potential coupling to the inherent microbial diagenetic activity.
In the present study we measured the horizontal and vertical variations in viral production and related the rates to the microbial heterotrophic respiration of sediments in the upwelling zone off central Chile. This area is extremely productive (Daneri et al. Citation2000) and the high sedimentation of phytoplankton-derived organic matter results in benthic diagenetic activities that are among the highest reported from open waters (Thamdrup & Canfield Citation1996; Glud et al. Citation1999). The data are used to infer the importance of viruses for bacterial mortality and secondary bacteria production along a depth transect from the shallow coastal area to deeper and less active shelf sediments.
Material and methods
Study site and sampling
Sampling was conducted on 5–7 January 2004 from RV Kai Kai at four stations (C4, C7, C14 and C18) along a transect from Concepción Bay to the continental margin off the bay, and at a single station in Coliumo Bay (, ). The transect expressed a gradient in water depth from 14 m in Coliumo Bay to 93 m at C18. The region is an upwelling area characterized by high primary production (monthly averages of 3–6 g C m−2 day−1 during the productive period from September to November; Daneri et al. Citation2000), and periodical low oxygen concentration or anoxia in the bottom water. The area is well known for mass occurrence of the mat forming filamentous sulphur bacteria Thioploca sp. during periods with upwelling (Fossing et al. Citation1995). Consequently, the sediment is relatively rich in organic matter with organic carbon contents in the surface sediment ranging from ∼6% at shallow bay stations to 4–5% at the shelf, and decreasing with depth to minimum values of ∼3% below 5 cm at all stations (Thamdrup & Canfield Citation1996).
Table I. Position and basic characteristics of the investigated stations.
At each station, sediment cores were collected with a multiple corer (Barnett et al. Citation1984) and cores were kept in bottom water at in situ conditions while on board. In all cases, the salinity and temperature in the bottom water were 35.2–35.4‰ and 10.8–12.0°C, respectively. Upon return to the laboratory within 3–4 h after sampling, the sediment cores were placed in a cold room at in situ temperature (11°C), where all the following work was carried out. The cores were analysed for total exchange rates of oxygen and dissolved inorganic carbon (DIC), and the depth distribution of porosity, organic carbon content, bacteria and VLP abundance. Furthermore, a set of cores was used for performing anoxic, homogenized sediment incubations to follow changes in the DIC concentration, and bacteria and VLP abundance over time.
Sediment characteristics
Profiles of sediment porosity and organic carbon were measured at a vertical resolution of 2 cm at all stations. Porosity was determined from density and weight loss after drying at 105°C for 24 h.
The total benthic exchange rate of oxygen and DIC was measured in duplicate whole core incubations as a measure of the integrated benthic mineralization rate (Glud et al. Citation1999). After 24 h pre-incubations at an in situ oxygen concentration (maintained by flushing with a nitrogen/air mixture), the submerged cores were capped with a gas-tight lid and the oxygen decrease was measured continuously with an oxygen mini-electrode inserted through a small perforation in the lid (Rasmussen & Jørgensen Citation1992). During incubations, a small rotating Teflon-coated magnet maintained a well-mixed overlying water phase. At regular intervals, samples for DIC quantification were obtained and preserved in 7 ml gas-tight glass containers (Exetainers) spiked with 200 µl of saturated HgCl2 until later analysis. The total DIC concentration was measured by infrared gas analysis (ADC 225 MK3) after acidification. The DIC and oxygen exchange rates were calculated from the linear concentration change over time accounting for the volume of enclosed water. Prior to whole core incubation, the oxygen microdistribution was measured with a Clark-type microelectrode with an internal reference and guard cathode (Revsbech Citation1989). This was, however, only performed at the true oxic station of Coliumo Bay ().
Extraction and enumeration of VLP and bacteria
The vertical distribution of bacteria and VLP was determined at a depth resolution of ∼2cm after extraction, according to Glud & Middelboe (Citation2004). For extraction, 4 g of sediment was weighed and transferred to a 50 ml Falcon tube. The samples were amended with 4 ml of 0.02 µm filtered bottom water, 1 ml of sodium pyrophosphate (10 mM final concentration), and 1 ml of glutaraldehyde (3% final concentration), and mixed thoroughly. After 15 min, the tubes were placed in a sonication bath for 2×1 min followed by centrifugation at 100g for 6 min. The supernatant was transferred to new tubes and the centrifuged sediment was washed twice with 0.02 µm filtered bottom water. The total extract was pooled and stored at 5°C for no more than 24 h until preparation of the slides for epifluorescence microscopy. It is important to reduce the time between preservation of the sample and slide preparation as viruses may decay rapidly in aldehyde-preserved samples (Glud & Middelboe Citation2004; Wen et al. Citation2004). Although our previous tests showed that the loss in viral counts was relatively low in sediment extracts during the first 24 h of storage (Glud & Middelboe Citation2004), a variable storage time may still account for some of the variability in viral abundance between samples. It should be noted that only the extractable fraction of viruses and bacteria was quantified by the applied method (i.e. the abundance of viruses and bacteria in the extract after removal of larger sediment particles by centrifugation). For the viruses, recent tests showed that centrifugation of the sediment did not affect the abundance of viruses relative to uncentrifuged samples (Fischer et al. Citation2005). The bacteria, on the other hand, are probably more difficult to dislodge and extract from the sediment than viruses, and it has previously been demonstrated (Middelboe et al. Citation2003a; Siem-Jørgensen Citation2005) that a significant portion of benthic bacteria are not released from the sediment by the applied treatments. Moreover, the abundance of benthic bacteria obtained by direct counts of sonicated and highly diluted uncentrifuged sediment samples have generally been up to 10-fold higher than observed in the present study (e.g. Velji & Albright Citation1985). Consequently, the present quantification of total extractable bacteria probably underestimated the total number of bacteria in the sediment.
The slides were prepared using SYBR-green I and II (Glud & Middelboe Citation2004), following the protocol of Noble & Fuhrman (Citation1998). A 200 µl extract was filtered onto 0.02 µm filters (Anodisc) and stained for 15 min, and mounted on a glass slide. The slides were kept at −20°C until counting and 300–600 bacteria and VLP were counted in 10–15 fields on each slide. The abundance of bacteria and VLP was not measured in replicate in the individual subsamples. However, replicate samplings in selected samples (i.e. at time zero in the anaerobic incubations) showed that the average abundance was determined with an error of 2–7%. This was a confirmation of results from previous studies, where we obtained a similar precision in the determination of VLP and bacterial abundance in a given sample using the applied procedure (Middelboe et al. Citation2003a; Glud & Middelboe Citation2004).
Virus production experiments
At each station, the depth interval of 0.5–2 cm from three cores was pooled in a gas-tight plastic Würgler bag and homogenized (Hansen et al. Citation2000). At C18, additional incubations were established for the sediment horizons of 20–25 and 80–90 cm depth. The bags were flushed with nitrogen gas and incubated at in situ temperature. Subsamples for DIC, bacterial and VLP counts were collected at regular intervals for ∼24 h. At each sampling occasion, the bags were carefully flushed with nitrogen to avoid any oxygen contamination. A sample of 4 g of sediment was transferred to a 50 ml Falcon tube for the extraction of bacteria and viruses, as described above, and 10 ml gas-tight containers (Exetainers) were filled with sediment. The Exetainers had no gas phase, and were centrifuged at 3000g for 5 min to allow for sampling of 1 ml of pore water that was transferred to a 1 ml gas-tight glass container spiked with 20 µl of saturated HgCl2. The samples were kept at 5°C until the DIC analysis (see above).
Rates of viral and bacterial production and bacterial respiration were estimated as the slope of the regression line based on the initial linear increase in abundances and DIC concentration, respectively. Because the virus production experiments were performed in single bags, the standard deviations of the rate measurements were derived from the linear regression measurements in the individual incubations, and calculated as the standard deviation of the slope of the regression lines, based on a variable number of data points (four to five). The statistical significance of the differences between obtained rates of microbial activity and production rates in the different treatments was estimated by comparing the slopes of the regression lines using Student's t-test.
Based on virus production estimates, the rate of bacterial lysis was estimated assuming a burst size of 14 (i.e. virus production divided by 14; Glud & Middelboe Citation2004). The impact of viral lysis on bacterial production was then calculated as the cell lysis rate divided by bacterial net production (i.e. not including viral-lysed cells). This allowed an estimation of the theoretical net bacterial production in the absence of viruses.
Sulphate reduction rates (SRR)
The bacterial SRR was quantified in duplicate cores from each station with a vertical resolution of 2 cm down to 20 cm. At C18, the SRR was measured down to 80 cm depth. Five microlitres (250 kBq) of 35S–SO4 2 − (Amersham) was injected into the cores through perforations in the core liners, and the cores were incubated for 24 h. The SRR was estimated from the reduction of 35S–SO4 2 − to total reducible inorganic sulphur (TRIS = H2 35S, Fe35S, Fe35S2, and 35S0) determined by the one-step acidic Cr-II method (Fossing & Jørgensen Citation1989). The Zn35S and 35SO4 2 − radioactivity were measured in a liquid scintillation counter. The SRRs were calculated from the fraction of reduced sulphur produced during incubation and the sulphate concentration according to Jørgensen & Fenchel (Citation1974) using an isotopic fractionation factor of 1.06. In order to compare total DIC production rates and the SRR, the latter was multiplied by two according to the stoichiometry: H++CH2O +½SO4 2 − →CO2+½H2S + H2O (Canfield et al. Citation1993). Sulphate concentrations were measured in diluted and filtered samples by non-suppressed anion-exchange chromatography.
Results
Sediment characteristics
Sediment porosity ranged from 0.78 to 0.98 vol:vol in the surface layers and decreased in all cores with sediment depth to 0.58–0.82 vol:vol at 18 cm (). At station C4, porosity was generally higher than at the other stations, and particularly the upper 4 cm contained very soft, floating material with porosities of 0.95–0.98. Visible mats of Thioploca sp. were present at all locations, except from C18, and covered 70–80% of the sediment surface at all the remaining stations.
The oxygen concentration in the bottom water was low in the shallow Coliumo Bay (68 µM) and decreased to below the detection limit (2 µM) with increasing water depth (). Consequently, oxygen uptake was only measurable in Coliumo Bay, where total and diffusive oxygen uptake corresponded to 40.7±30.7 mmol C m−2 day−1 and 11.7±1.2 mmol C m−2 day−1, respectively, and the oxygen penetration depth was only 0.46 mm ().
Table II. Bacterial activities at the different stations.
The transect from Concepción Bay to the deeper stations (C4→C18) on the shelf expressed a gradient in benthic microbial activity (). Total DIC exchange rates were highest at C4 (168 mmol C m−2 day−1) and decreased along the transect to 26.2 mmol C m−2 day−1 at C18. Apparently the coarser sediment of the shallowest station in Coliumo Bay had a benthic mineralization rate that was similar to the activity at C18 (). As for the DIC production, the depth-integrated SRR decreased from a maximum of 66.2 mmol C m−2 day−1 at C4 to 11.2 mmol C m−2 day−1 at C18. The SRR thus explained 39–82% of total benthic respiration, indicating that other electron acceptors such as Fe oxides also sustained the heterotrophic DIC production at the respective sites.
Vertical profiles of the SRR showed highest activity in the upper 2 cm, with an additional peak at 6–8 cm depth at the two inner stations (). Surface activities ranged within a factor of 10 from 0.078 µmol SO4 −2 cm−3 day−1 at C18 to 0.76 µmol SO4 −2 cm−3 day−1 at C14 (). Minimum rates were obtained in the deep samples at C18, with relatively constant levels of 0.001–0.002 µmol SO4 −2 cm−3 day−1 below 30 cm (data not shown).
Vertical distribution of bacteria and VLP
For all stations, VLP abundance decreased with sediment depth in the upper 10 cm (A). However, there were no clear trends in the abundances moving along the transect where the surface densities ranged between 2.0 and 3.0×108 VLP cm−3, and the values did not correlate to the measured microbial activity. At all stations, a secondary peak in abundance was encountered at sediment depths below 10 cm and minimum values of 7×107 VLP cm−3 was either observed at 18 cm depth for the least active sites (CB, C14, C18) or at intermediary sediment depths of ∼6 − 8 cm (C4, C7) (). Total extractable bacteria in all instances expressed a decrease with sediment depth from 1.9–4.8×107 cells cm−3 at the surface to 0.4–1.4×107 cells cm−3 below 15 cm (B). The SRR was significantly correlated to the bacterial abundance (r2=0.74, n = 40, P < 0.001).
Virus production experiments
There was a significant linear increase in viral abundance over time in all the incubations (). Net VLP production of the surface sediment peaked at C4 with rates of 6.0×106±7×105 VLP cm−3 h−1 and reached a minimum value of 2.7×106±8×105 VLP cm−3 h−1 at C18 (significantly lower than at C4, P < 0.05) (). In Coliumo Bay, net VLP production (3.5×106±6×105 VLP cm−3 h−1) was significantly lower than at C4. At C18, VLP production decreased with sediment depth from 2.7×106±8×105 VLP cm−3 h−1 at the surface sediment to 2.3×106±3×105 VLP cm−3 h−1 at 20–25 cm depth and 1.8×106±3×105 VLP cm−3 h−1 at 80–90 cm (). However, the rates were not significantly different.
Figure 4. Measured changes in virus-like particle abundance (VA) over time (t) in anaerobic Würgler bag incubations of homogenized sediment. The data represent incubations of the surface layer (0.5–2 cm) for all the investigated stations as well as two deep layers (20–25 and 80–90 cm) from C18. The regression lines are described by the following functions: Coliumo Bay: VA = 3.5×106 t + 1.7×108, r2=0.99; C4: VA = 6.0×106 t + 1.4×108, r2=0.96; C7: VA = 3.9×106 t + 1.3×108, r2=0.94; C14: VA = 4.9×106 t + 1.4×108, r2=0.94; C18 (0.5–2 cm): VA = 2.7×106 t + 1.2×108, r2=0.79; C18 (20–25 cm): VA = 2.3×106 t + 1.0×108, r2=0.96; C18 (80–90 cm): VA = 1.8×106 t + 0.9×108, r2=0.91. In all cases, there was a significant (P < 0.05) linear increase in virus-like particle (VLP) abundance with time, and VLP production was estimated from the slope of the regression lines.
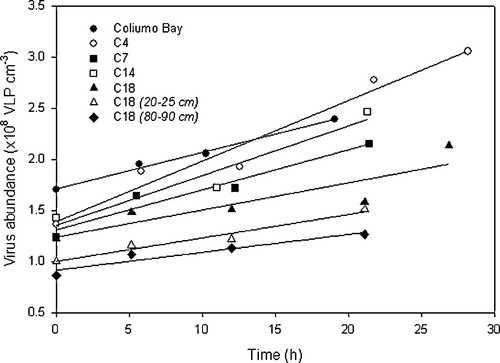
Figure 5. Bacterial and virus-like particle production along the transect. Production rates were measured in anaerobic incubations of homogenized sediment from the 5–2 cm sediment layer and calculated from the linear increase in viral and bacterial abundance with time (). The error bars represent the standard deviations of the slopes of the linear regression lines.
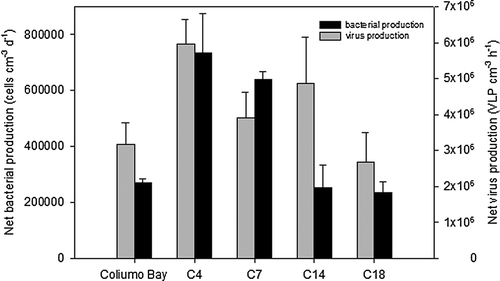
Bacterial net production showed a horizontal pattern that resembled viral activity distribution with significantly higher rates in the inner Concepción Bay stations C4 and C7, 6.4–7.3×105 cells cm−3 h−1, than in Coliumo Bay and at the shelf stations, 2.3–2.5×105 cells cm−3 h−1 (P < 0.05) (). Bacterial production correlated significantly with DIC accumulation of the bag incubations (r2=0.81, P < 0.01, ), suggesting that bacterial activity was directly reflected in cell production. The slope of the regression line indicated that 1.7×1010 cells were produced per mmol DIC, indicating a bacterial carbon conversion efficiency of approximately 3–14% in the system (applying a range in bacterial cell carbon content of 20–100 fg C; Simon et al. Citation1990). The VLP net production obtained during the bag incubations correlated significantly with total DIC exchange rates (r2=0.94, P < 0.001, ) measured in the parallel whole core incubations. Moreover, the VLP production correlated with bacterial respiration (DIC production) in the anaerobic incubations (, black squares).
Figure 6. Net bacterial production (NBP) as a function of bacterial respiration [dissolved inorganic carbon (DIC) production] in the anaerobic sediment incubations. The line represent the linear regression (NBP = 1.7×107 DIC − 0.021, r2=0.81, P < 0.01).
![Figure 6. Net bacterial production (NBP) as a function of bacterial respiration [dissolved inorganic carbon (DIC) production] in the anaerobic sediment incubations. The line represent the linear regression (NBP = 1.7×107 DIC − 0.021, r2=0.81, P < 0.01).](/cms/asset/13bda734-8792-40e1-ad0f-cc66f4a4c6ed/smar_a_162046_o_f0006g.gif)
Figure 7. Virus-like particle production (VP) in the anaerobic incubations (0.5–2 cm sediment layer) as a function of the corresponding total benthic respiration (BR) [measured as the dissolved inorganic carbon (DIC) exchange rate during whole core incubations]. Values are given as±standard deviation, as estimated from the slope of the linear regression lines. The regression line is described by the function VP = 2.2×104 BR + 2.5×106, r2=0.94, P < 0.001.
![Figure 7. Virus-like particle production (VP) in the anaerobic incubations (0.5–2 cm sediment layer) as a function of the corresponding total benthic respiration (BR) [measured as the dissolved inorganic carbon (DIC) exchange rate during whole core incubations]. Values are given as±standard deviation, as estimated from the slope of the linear regression lines. The regression line is described by the function VP = 2.2×104 BR + 2.5×106, r2=0.94, P < 0.001.](/cms/asset/d505153b-2e8b-4272-8037-8042a8fd5c03/smar_a_162046_o_f0007g.gif)
Figure 8. A cross system analysis of the correlation between virus production (VP) and bacterial respiration (BR) during anaerobic bag incubations. Data are from Øresund, Denmark (Glud & Middelboe Citation2004) (VP = 3.7×105 BR − 5.3×106; r2=0.90, P < 0.01), Sagami Bay, Japan (Middelboe et al. Citation2006) (VP = 3.3×104 BR + 1.5×106; r2=0.98, P < 0.01) and the Chilean upwelling zone (present study) (VP = 9.3×104 BR + 5.3×106; r2=0.96, P < 0.01).
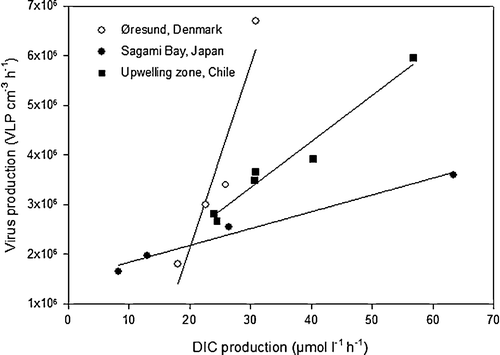
Discussion
Vertical and horizontal variations in VLP abundance
The range in total benthic mineralization of 26–168 mmol C m−2 day−1 () corresponded well with previous measurements in the same area, and supported the conclusion that benthic activity in the Chilean upwelling zone is high compared with coastal sites in general (Thamdrup & Canfield Citation1996; Glud et al. Citation1999). In the shallow bay area (), benthic mineralization is partly fuelled by urban and terrestrial input of organic matter, but the continued high rates of carbon oxidation at the shelf station (C18) reflect the extremely high primary productivity in the area due to upwelling of nutrient-rich bottom water (Daneri et al. Citation2000). Consequently, the bottom water becomes oxygen depleted and benthic mineralization is dominated by anaerobic processes throughout the sediment. In our investigation, the sulphate reduction accounted for 40–80% of total respiration, which is slightly lower than previously encountered, where the range was 89–117% (Thamdrup & Canfield Citation1996). This, could reflect interannual variations in the sediment biogeochemistry. The importance of sulphate reduction for the benthic mineralization activity was also indicated by the significant positive correlation between sulphate reduction and total extractable bacteria in the present study.
The abundance of bacteria and VLP was relatively constant along the investigated transect, regardless of the fact that the different stations represented a range of trophic conditions. Thus, the overall microbial activity and trophic condition per se seem not to be strong indicators for the total viral abundance at a given location. Rather, the spatial variations in viral and bacterial abundance are probably regulated on a smaller scale, by microscale dynamics of bacterial and viral activity, which are beyond the temporal and spatial resolution of activity measurements employed here. In the present case, the rather variable vertical distribution of viral and bacterial abundance may have been influenced by the activity of Thioploca sp. The microbial mats formed by these giant filamentous sulphur bacteria covered most of the investigated sediment, with individual filaments reaching 10–15 cm into the sediment (Huettel et al. Citation1996). The vertical migration of the Thioploca filaments in response to changes in oxygen, nitrate and sulphide profiles may, contribute to the spatial heterogeneity in viral and bacterial distribution. Thioploca-specific viruses may also have been present. However, an analysis of thin sections of Thioploca filaments by transmission electron microscopy did not reveal any visibly infected cells.
In general, the measured abundance of viruses at a given time and place is the result of the preceding rates of production and decay, and do therefore not necessarily show a close coupling with the concomitant microbial activity. Virus production, on the other hand, should be expected to display a more direct association with microbial activity. That was indeed the case in the present study, as will be discussed below.
Virus production and its coupling to bacterial activity
The obtained rates of virus production (3–8×106 VLP cm−3 h−1) in the upper anaerobic sediment (0.5–2 cm) were lower than previous measurements from surface sediments (). An overview of the published rates of benthic virus production to date () illustrates that the rates vary by a factor of >100 between different studies and indicates that surface sediments of shallow eutrophic waters have relatively high rates (up to 400×106 VLP cm−3 h−1) compared with deeper sediment layers (2–8×106 VLP cm−3 h−1) and deep-sea sediments (2×106 VLP cm−3 h−1).
Table III. Overview of available data on rates of benthic virus production.
However, the fact that the highest values were all obtained in slurry-based incubations (Hewson & Fuhrman Citation2003; Fischer et al. Citation2003; Mei & Danovaro Citation2004) could indicate that different approaches in the estimation of viral production may affect the obtained rates. Slurry incubations are known to stimulate bacterial activity above that of bag incubations and whole core incubations (e.g. Hansen et al. Citation2000), but it still remains to be shown to what extent they also enhance VLP production. However, the potential impact of the incubation procedure needs to be taken into account when virus production estimates from different studies are compared.
The observation of a net accumulation of VLP and bacteria in sediment incubations in general indicates that bacterial activity is stimulated by sediment handling and homogenization. Assuming that viral abundance is at quasi steady state on a short time scale, little or no net production should be expected in undisturbed sediment kept at in situ conditions. On the other hand, a recent seasonal study of benthic viral abundances demonstrated significant periodical accumulation and subsequent decay of total viral numbers on a monthly basis (Siem-Jørgensen Citation2005). The present observation of VLP and bacteria accumulation in the bag experiments could potentially reflect a short-term non-steady state in abundances of surface sediments. However, it is likely that the applied experimental conditions enhanced viral production in the bags compared with in situ rates, and that the obtained rates therefore reflected the potential rather than the actual virus activity.
Even though the VLP production rates obtained in the present study probably cannot be directly interpreted as actual in situ rates, the measurements allow a comparison of potential viral activity at different locations, thus providing valuable information about spatial variability in viral activity.
Interestingly, significant virus production (1.8×106 VLP cm−3 h−1; r2=0.86, P < 0.05) was detected even at 80–90 cm depth (). This demonstrated the potential for the production of new viruses even in these deep sediment layers, which were characterized by relatively low SRR of ∼0.002 µmol SO4 cm−3 day−1.
Virus production was strongly correlated with bacterial respiration in the anaerobic Würgler bag incubations (r2=0.96, P < 0.01). When we summarize the results from our studies of virus production in different environments [shallow eutrophic coastal area (Øresund); Glud & Middelboe Citation2004; deep-sea station (Sagami Bay); Middelboe et al. Citation2006; and the present study], we in all cases find a significant positive correlation between VLP production and the simultaneously obtained bacterial metabolic activity (measured as DIC production) (). The general observation that the VLP production is slightly delayed relative to any increase in bacterial respiration or production indicates that the microbial metabolic rate is the prime factor regulating the benthic VLP production. These data are directly comparable as the same experimental approach was applied in all three studies. Together, the data provide solid evidence that benthic viruses (1) are dynamic components of the benthic microbial community with turnover times of 0.5–6 days, and (2) respond rapidly to environmental changes, such as temperature and/or organic matter addition (Glud & Middelboe Citation2004; Middelboe et al. Citation2006).
The cross system analysis also indicated that there is no universal relationship between bacterial respiration and VLP net production, in the sense that the slope of the relationship differs among environments. The underlying reason for these differences is not obvious, but may well be related to differences in burst size, the dominant life cycle (lytic, lysogenic, chronic) of the benthic viruses, or specific differences in the virus and bacteria community at the respective sites.
The compiled data of clearly demonstrate that VLP production responds to changes in microbial activity during anoxic incubations of homogenized sediment. However, the data from the present study bring us further than that. Here we have also demonstrated a significant correlation between the potential virus production and the actual benthic mineralization rates measured on intact cores along the transect (P < 0.001, ). The coupling between VLP production and bacterial activity is thus not only a phenomenon that goes on in our incubation set-up, but probably illustrates a general response of marine sediments and provides an important contribution to understand the mechanisms regulating viral activity in marine sediments. Our data thus suggested that benthic viral activity in general is determined by the activity of the corresponding bacterial assemblage (i.e. here represented by the total benthic mineralization rate and net cell production) rather than by their total abundance.
The interpretation of our viral production data is to some extent limited by the fact that only single incubations were established for each station, which means that the statistics relied on regression analysis of the increases in viral and bacterial activity in individual bags. However, we have previously demonstrated that such incubations of homogenized sediment samples are reproducible, with only small variations in rate measurements between parallel incubations (<10% standard deviation) (Glud & Middelboe Citation2004). We are confident, therefore, that the observed coupling between viral production and bacterial mineralization along the transect represents a true spatial gradient in microbial activity.
Viral impact on bacterial mortality and benthic carbon cycling
Estimates of virus-mediated bacterial mortality and dissolved organic carbon (DOC) production are sensitive to a number of factors. Applied conversion factors (e.g. burst size and cell carbon content), methods used to quantify bacterial production (e.g. net accumulation of cells, incorporation of radioactive tracers), and the above-mentioned differences in incubation approach (e.g. whole sediment, bag incubations and slurries), are all factors that will affect the estimates. In the present study, the impact of viral lysis on bacterial mortality was estimated from virus production measurements and assuming a burst size of 14 (Glud & Middelboe Citation2004). Using that approach, bacterial mortality ranged between 2 and 4×105 cells lysed cm−3 h−1, corresponding to 44–138% of the measured net bacterial production in the incubations (). Because already lysed cells are not included in the estimate of net bacterial production, this means that the observed production theoretically would have been 44–138% higher in the absence of viruses. The results thus indicated that viral activity significantly reduced the accumulation of cells in the incubations, and therefore may have large implications for the fate of bacterial production in the sediment.
Table IV. Virus-induced bacterial mortality, bacterial respiration and virus-mediated dissolved organic carbon (DOC) production in anaerobic sediment incubations.
The values were in line with our previous estimates of virus-induced bacterial mortality (20–60% of bacterial net production) in other benthic environments using the same approach (Glud & Middelboe Citation2004; Middelboe et al. Citation2006). Also, other studies, applying different approaches to estimate virus-induced mortality, have found significant viral control of bacterial production (e.g. 1–42%; Fischer et al. Citation2004; 20–60%; Mei & Danovaro Citation2004) and abundance (4–14% h−1; Hewson & Fuhrman Citation2003). The observed ranges of virus-induced bacterial mortality from benthic investigations are, thus, in line with observations from pelagic systems (e.g. Fuhrman Citation1999; Weinbauer Citation2004), and suggest that the influence of viral activity on bacterial population dynamics may be equally important in benthic environments. Other studies, on the other hand, have found that viruses contributed insignificantly to benthic bacterial mortality (e.g. average 6%; Fischer et al. Citation2003), emphasizing the need for further investigations on this matter and an evaluation of the variety of applied methods. In general, the applied method for the quantification of benthic bacterial abundance results in an underestimation of bacterial abundance (e.g. Hewson & Fuhrman Citation2003), which means that the viral mortality of bacteria in sediments could be smaller than indicated here.
Virus-mediated DOC production amounted to 0.3–3.5 nmol C cm−3 h−1 (), and was estimated from bacterial cell lysis (i.e. based on virus production and a burst size of 14) and a range of theoretical cell carbon contents. Compared with total bacterial carbon demand (i.e. DIC production during incubation), the release of lysates turned out only to sustain between 1 and 8% of the bacterial respiration (). This was consistent with most previous estimates (e.g. Fischer et al. Citation2003; Mei & Danovaro Citation2004; Glud & Middelboe Citation2004; Middelboe et al. Citation2006), and suggests that viral-mediated recycling of organic carbon seems to contribute insignificantly (<10%) to bacterial carbon demand, and thus plays a minor role in benthic environments. This is in contrast to pelagic systems, where viral lysates potentially have been shown to contribute significantly as the substrate for bacterial production (Wilhelm & Suttle Citation1999; Middelboe et al. Citation2003b).
As demonstrated above, a number of interesting generalizations concerning the role of viruses for benthic microbial mortality and carbon cycling can be extracted from the accumulating data of benthic viral activity. It is at the same time obvious, however, that a basic knowledge on conversion factors (e.g. burst size and cell carbon content) and the influence of the applied methodology (e.g. extraction and incubation procedures) still needs to be elucidated before we can obtain a more comprehensive understanding of the impact of viruses on benthic processes on variable spatial and temporal scales.
Editorial responsibility: Antje Boetius
Acknowledgments
We wish to thank Professor Victor Ariel Gallardo, Dr Javier Sellanes and Sergio Contreras, University of Concepción for invaluable help during our stay in Dichato. Anni Glud is acknowledged for excellent technical assistance and Kai Finster for running the sulphate reduction analyses. The study was supported by the Danish Natural Sciences Research Council. Three anonymous reviewers and subject editor Antje Boetius provided valuable suggestions that improved the manuscript.
Notes
Published in collaboration with the University of Bergen and the Institute of Marine Research, Norway, and the Marine Biological Laboratory, University of Copenhagen, Denmark
References
- Barnett , PRO , Watson , J and Conelly , D . 1984 . A multiple corer for taking virtually undisturbed samples from shelf, bathyal, and abyssal sediments . Oceanologica Acta , 7 : 399 – 408 .
- Bird , DF , Juniper , SK , Ricciardi-Rigault , M , Martineu , P , Prairie , YT and Calvert , SE . 2001 . Subsurface viruses and bacteria in Holocene/Late Pleistocene sediments of Saanich Inlet, BC: ODP holes 1033B and 1034B, Leg 169S . Marine Geology , 174 : 227 – 39 .
- Canfield , DE , Jørgensen , BB , Fossing , H , Glud , RN , Gundersen , JK , Thamdrup , B , Hansen , JW , Nielsen , LP and Hall , POJ . 1993 . Pathways of organic carbon oxidation in three continental margin sediments . Marine Geology , 113 : 27 – 40 .
- Daneri , G , Dellarosa , V , Quiñones , R , Jacob , B , Montero , P and Ulloa , O . 2000 . Primary production and community respiration in the Humboldt Current System off Chile and associated oceanic areas . Marine Ecology Progress Series , 197 : 41 – 9 .
- Danovaro , R , Corinaldesi , C , Dell'Anno , A , Fabiano , M and Corselli , C . 2005 . Viruses, prokaryotes and DNA in the sediments of deep-hypersaline anoxic basin (DHAB) of the Mediterranean Sea . Environmental Microbiology , 7 : 586 – 92 .
- Danovaro , R and Serresi , M . 2000 . Viral density and virus–bacterium ratio in the deep-sea sediments of the eastern Mediterranean . Applied and Environmental Microbiology , 66 : 1857 – 61 .
- Fischer , UR , Kirschner , AKT and Velimirov , B . 2005 . Optimization of extraction and estimation of viruses in silty freshwater sediments . Aquatic Microbial Ecology , 40 : 207 – 16 .
- Fischer , UR , Weisz , W , Wieltschnig , C , Kirschner , KT and Velimirov , B . 2004 . Benthic and pelagic viral decay experiments: a model-based analysis and its applicability . Applied and Environmental Microbiology , 70 : 6706 – 13 .
- Fischer , UR , Wieltschnig , C , Kirschner , AKT and Velimirov , B . 2003 . Does virus-induced lysis contribute significantly to bacterial mortality in the oxygenated sediment layer of shallow Oxbow lakes? . Applied and Environmental Microbiology , 69 : 5281 – 9 .
- Fossing , H , Gallardo , VA , Jørgensen , BB , Huettel , M , Nielsen , LP , Schulz , H , Canfield , DE , Forster , S , Glud , RN , Gundersen , JK Küver , J . 1995 . Concentration and transport of nitrate by the mat-forming sulphur bacterium Thioploca . Nature , 374 : 713 – 5 .
- Fossing , H and Jørgensen , BB . 1989 . Measurement of bacterial sulfate reduction in sediments: evaluation of the single-step chromium reduction method . Biogeochemistry , 8 : 223 – 45 .
- Fuhrman , JA . 1999 . Marine viruses and their biogeochemical and ecological effects . Nature , 399 : 541 – 8 .
- Glud , RN , Gundersen , JK and Holby , O . 1999 . Benthic in situ respiration in the upwelling area off central Chile . Marine Ecology Progress Series , 186 : 9 – 18 .
- Glud , RN and Middelboe , M . 2004 . Virus and bacteria dynamics of a coastal sediment: implication for benthic carbon cycling . Limnology and Oceanography , 49 : 2073 – 81 .
- Hansen , JW , Thamdrup , B and Jørgensen , BB . 2000 . Anoxic incubation of sediment in gas-tight plastic bags: a method for biogeochemical process studies . Marine Ecology Progress Series , 208 : 273 – 82 .
- Hewson , I and Fuhrman , JA . 2003 . Viriobenthos production and virioplankton sorptive scavenging by suspended sediment particles in coastal and pelagic waters . Microbial Ecology , 46 : 337 – 47 .
- Hewson , I , O'Neil , JM , Fuhrman , JA and Dennison , WC . 2001 . Virus-like particle distribution and abundance in sediments and overlying waters along eutrophication gradients in two subtropical waters . Limnology and Oceanography , 46 : 1734 – 46 .
- Huettel , M , Forster , S , Klöser , S and Fossing , H . 1996 . Vertical migration in the sediment-dwelling sulphur bacteria Thioploca spp. in overcoming diffusion limitations . Applied and Environmental Microbiology , 62 : 1863 – 72 .
- Jørgensen , BB and Fenchel , T . 1974 . Sulfur cycle of a marine sediment model system . Marine Biology , 24 : 189 – 201 .
- Mei , ML and Danovaro , R . 2004 . Virus production and life strategies in aquatic sediments . Limnology and Oceanography , 49 : 459 – 70 .
- Middelboe , M , Glud , RN and Finster , K . 2003a . Distribution of viruses and bacteria in relation to diagenetic activity in an estuarine sediment . Limnology and Oceanography , 48 : 1447 – 56 .
- Middelboe , M , Glud , RN , Wenzhöfer , F , Oguri , K and Kitazato , H . 2006 . Spatial distribution and activity of viruses in the deep-sea sediments of Sagami Bay, Japan . Deep-Sea Research I , 53 : 1 – 13 .
- Middelboe , M , Riemann , L , Steward , GF , Hansen , V and Nybroe , O . 2003b . Virus-induced transfer of organic carbon between marine bacteria in a model community . Aquatic Microbial Ecology , 33 : 1 – 10 .
- Noble , RT and Fuhrman , JA . 1998 . Use of SYBR-Green I for rapid epifluorescence counts of marine viruses and bacteria . Aquatic Microbial Ecology , 14 : 113 – 8 .
- Rasmussen , H and Jørgensen , BB . 1992 . Microelectrode studies of seasonal oxygen uptake in a coastal sediment: role of molecular diffusion . Marine Ecology Progress Series , 81 : 289 – 303 .
- Revsbech , NP . 1989 . An oxygen microelectrode with a guard cathode . Limnology and Oceanography , 34 : 474 – 8 .
- Siem-Jørgensen M. 2005 . Virus and bacteria dynamics in a coastal sediment: seasonal variability in abundance . Master thesis . University of Copenhagen .
- Simon , M , Alldredge , AL and Azam , A . 1990 . Bacterial carbon dynamics on marine snow . Marine Ecology Progress Series , 65 : 205 – 11 .
- Suttle , CA . 2005 . Viruses in the sea . Nature , 437 : 356 – 61 .
- Thamdrup , B and Canfield , D . 1996 . Pathways of carbon oxidation in continental margin sediments off central Chile . Limnology and Oceanography , 41 : 1629 – 50 .
- Velji , MA and Albright , LJ . 1985 . Microscopic enumeration of marine bacteria of seawater, marine sediment, fecal matter and kelp blade samples following pyrophosphate and ultrasound treatments . Canadian Journal of Microbiology , 32 : 121 – 6 .
- Weinbauer , MG . 2004 . Ecology of prokaryotic ciruses . FEMS Microbiology Reviews , 28 : 127 – 81 .
- Wen , K , Ortmann , AC and Suttle , CA . 2004 . Accurate estimation of viral abundance by epifluorescence microscopy . Applied and Environmental Microbiology , 70 : 3862 – 7 .
- Wilhelm , SW and Suttle , CA . 1999 . Viruses and nutrient cycles in the sea . Bioscience , 49 : 781 – 8 .