ABSTRACT
Colour vision disorders, pose challenges in perceiving and distinguishing specific colours. To address these challenges, technologies such as colour-filtering glasses and contact lenses have been developed. However, customisation of eyewear for individual patients remains a challenge. This research aims to design multifunctional contact lenses combining colour filtering and pH sensing capabilities for personalised colour vision correction. A pH-responsive, Neutral red dye-doped hydrogel was developed and optimised for the vat photo polymerization-based 3D printing process. A novel in-house multimaterial 3D printing technique was employed for printing lenses exhibiting both colour filtering and pH sensing capabilities. 3D printed samples contained outer radial rings, made from porous and pH-responsive polymer. The optically tunable dye-doped resin was also applied to the central part of the lenses to achieve customised wavelength blockage. The lenses demonstrate potential for customised colour vision correction and pH monitoring of the tear film.
1. Introduction
Colour vision disorders, also known as colour blindness, affect a significant portion of the population, posing challenges in perceiving and differentiating specific colours [Citation1]. These visual impairments, caused by genetic mutations or abnormalities in the photopigments of the retina’s cones, can significantly impact an individual’s daily life, affecting tasks ranging from identifying colour-coded information to recognising traffic lights [Citation2]. To address these challenges, various technologies, such as colour-filtering glasses [Citation3] or contact lenses, have been developed to enhance colour perception for individuals with colour vision deficiencies [Citation2,Citation4]. For colour correction in colour vision deficiencies, filters typically target around 530–560 nm wavelength band for red-green blindness [Citation4,Citation5], and around 440–490 nm wavelengths band for green–blue colour blindness (as per most commercial glasses) [Citation2]. For instance, in a recent study by Wong et al. [Citation6], they explored the use of 3D-printed medicine labels to improve colour identification for individuals with colour vision deficiency (CVD). While the concept is still in its early stages of development, it shows promise in aiding CVD patients with medication management. The idea of using wavelength filter-based visual aids was initially proposed by Seebeck [Citation7], who demonstrated that red and green filters could enable colourblind individuals to distinguish between certain colours. Since then, significant progress has been made in improving the properties and materials of wearable devices for CVD, leading to several commercial products [Citation4,Citation8]. Notably, Enchroma has been a prominent company specialising in glasses for colourblind individuals since 2012 [Citation3], while Chromagen focuses on dye-tinted contact lenses for CVD patients. However, despite claims of effectiveness by these companies, the customisation of eyewear for individual patients remains a significant challenge. Advancements in additive manufacturing technology, particularly in 3D printing, have revolutionised various fields, notably in producing medical devices tailored to specific applications [Citation9], this includes the creation of magnetic nanocomposite scaffolds and biodegradable scaffolds for bone tissue regeneration, using techniques like 3D fiber deposition modelling and Stereolithography. These innovations have led to more precise and versatile structures, enhancing cell adhesion and proliferation, and improving bone grafting methods. This progress in scaffold design and fabrication marks a major step forward in reconstructive surgery, showcasing the crucial role of additive manufacturing in evolving medical treatments and technologies [Citation10,Citation11].
Several dyes and metallic nano-particles were reported to aid in the direction of colour vision enhancement [Citation2,Citation8]. Surface plasmon resonance (SPR), which gives metallic nanoparticles their ability to absorb and scatter light, is what causes this optical behaviour [Citation12]. The SPR band is mostly dependent on the nanoparticle morphology and can be tailored by modifying the morphology-related parameters. The produced polymer nanocomposites exhibited absorption corresponding to the SPR of nanoparticles, indicating effective coating. Depending on the coating’s layer thickness, the SPR ranged from 425 to 600 nm. The produced composites displayed suitable characteristics and appeared to hold promise for the production of ultra-thin colour filters [Citation13]. Salih et al. [Citation8] claimed that silver nanoparticles (of 60 nm radius) based nanocomposite contact lenses are capable of blocking up to 65% of the undesired wavelengths (488 and 494 nm) that blue–yellow CVD patients struggle the most with. Despite the abundance of similar studies, there is a scarcity of research in the existing literature concerning the customisation of colour filters to meet the specific needs of individual patients, considering the severity of their colour confusion and the particular wavelength that causes the confusion. In that context, Tian et al. [Citation5] designed customised contact lenses depending on the level of severity of different CVD patients with wavelength blockage adjusted from 540 to 575 nm with the incorporation of gold NPs with various sizes and concentrations depending on the targeted wavelength. Their customised lenses ensure relatively consistent success as compared to some commercial lenses in the market that can worsen the vision experience due to targeting unmatched wavelengths of a specific patient.
In addition to colour vision enhancement, it is crucial to monitor ocular health, as conditions such as dry eye syndrome or inflammation can further compromise visual function [Citation14]. Tear pH, reflecting the acidity or alkalinity of the tear film, is a vital parameter in assessing ocular surface health. The normal range of tear pH in a healthy individual typically falls within a slightly acidic to slightly alkaline range [Citation15]. The average tear pH is around 7.4. However, in certain ocular conditions or illnesses, tear pH can deviate from the normal range [Citation16]. For example, in dry eye syndrome or ocular surface inflammation, the tear pH can decrease to as low as 6.0 or even lower [Citation17]. This acidic shift in tear pH is associated with increased tear osmolarity, decreased buffering capacity, and inflammation. On the other hand, tear pH values can occasionally rise above the normal range. As in certain types of ocular infections or microbial keratitis, tear pH may become more alkaline, exceeding 8.0 [Citation18]. Therefore, pH sensing is considered as a valuable diagnostic tool. Several attempts reported some potential techniques to evaluate tear pH values. Tear pH can be measured using pH-sensitive fluorescent probes in combination with a fluorometer [Citation19]. This method has been reported for contact lenses as well [Citation20]. Another approach involves using simple colorimetric pH monitoring poly-HEMA contact lenses that are cross-linked with an anthocyanin dye, which has been demonstrated in [Citation21]. Despite not being directly related, it is worth noting that certain ocular conditions or diseases that can affect tear pH may also have secondary effects on visual function, including colour vision [Citation22].
Multi-material 3D printing is revolutionising various sectors with its ability to craft both complex and functional devices, particularly noted for the range of non-conventional materials it can employ. This technology has advanced from aesthetic to functional applications, significantly influencing mechanical performance and design, especially at the interfaces of different materials [Citation23]. A notable innovation in this field is a low-cost, high-resolution 3D printing platform that integrates machine vision systems, enhancing calibration and enabling the simultaneous use of diverse materials [Citation24]. In the field of ocular health, multi-material 3D printing has made remarkable strides, specifically in the development of contact lenses. These lenses, crafted for colour blindness correction, employ two different dyes, each targeting a distinct type of colour blindness. This advancement showcases the potential of multi-material 3D printing in creating tailored, wearable devices, opening new avenues for personalised medical solutions [Citation25].
This work aims to design tunable and multifunctional contact lenses that combine colour filtering and pH sensing capabilities that enable personalised vision correction. By tailoring the colour filtering properties and adjusting the lens parameters based on an individual’s specific colour vision deficiency and ocular health profile, these lenses could provide more precise and optimised vision correction, improving overall visual experience and comfort. Herein, a pH-responsive dye called Neutral red was employed ((a)). Using the novel multi-material 3D printing technique, rings containing the dye resin were printed onto the transparent lenses to enable pH sensing. Additionally, the tunable dye resin was applied at the centre of the lenses after being immersed in the suitable pH solution. This aims to create customised wavelength blockage as per each patient’s requirement. Unlike the ring dye, the central dye was enclosed between the transparent resin layers to ensure that it is not influenced by the pH value of the tears. To the best of the author’s knowledge, neither the combination of both applications nor Neutral red dye’s utilisation for tunable colouring filtering in contact lenses have been reported in the current literature.
2. Materials and methods
2.1. Materials
In the resin formulation, 2-hydroxyethyl methacrylate (HEMA) was employed as the monomer, polyethylene glycol dimethacrylate (PEGDA) acted as the cross-linker, and trimethyl benzoyl diphenylphosphine oxide (TPO) served as the photoinitiator. Deionised water (DI) and Isopropyl alcohol (IPA) was utilised for washing the 3D-printed samples. The hydrogel was combined with Neutral red (C15H17N4) dye, which was dissolved in deionised (DI) water. All the chemicals were purchased from Sigma-Aldrich and used without further purification. A commercial contact lens solution (Renu) was utilised to replicate the conditions of the ocular surface. The osmolality of Renu solution is within the range of 275–310 mOsm/kg, which is comparable to the average osmolality of human tears, typically around 305 mOsm/kg. This similarity in osmolality is a key factor in justifying the use of Renu as a tear-like solution [Citation26].
2.2. Methodology
To create the dye solution stock, the dye powder was dissolved in DI water, with a ratio of 5 mg dye powder per 100 ml ((b)). The mixture was then stirred using a magnetic stirrer for 20 min. For the photocurable resin, a combination of HEMA and PEGDA was prepared with different volume ratios. A mixture of HEMA and PEGDA with a 1:1 ratio along with 5% TPO was used for printing the clear part of the lens. Another mixture with HEMA:PEGDA ratio of 10:1, combined with 1% TPO, was mixed with the dye solution at a ratio of 4:1, for printing the tinted parts of the lens. After experimentation, it was determined that a 25% water content was optimal, as further increases had a negative impact on the quality of the photopolymerization and crosslinking processes. To enhance the swelling capabilities and pH responsivity of the dye resin, a higher proportion of HEMA was found to be suitable when mixed with the dye. However, for the clear part of the lens, a 1:1 ratio of HEMA and PEGDA was deemed more appropriate, striking a balance between swelling performance, sufficient rigidity, and flexibility. To prepare dye resin samples with varying pH levels, the dye resin was mixed with buffer solutions, resulting in dye resin samples at pH levels of 4, 5, 6, 7, 8, 9, and 10.
The CAD models for disks and lenses were meticulously designed using SOLIDWORKS software. These models were saved in the widely recognised (.STL) format, which enables the printer to interpret and convert the model into printable layers. To optimise the curing time and thickness, 12 mm diameter disks with varying thicknesses were designed. For the printing of multi-material contact lenses, a contact lens CAD model with a diameter of 14 mm, depth of 2.5 mm, and wall thickness of 200 μm was utilised.
To transform the STL files into a readable format (G-code) for the printers, a slicing software called Lychee was employed. The printing process involved the use of three different printers. Firstly, a Digital Light Processing (DLP) printer, the Wanhao duplicator 7, was specifically dedicated to studying the impact of curing time and thickness on pH responsivity. Another DLP printer, the Wanhao duplicator 8, was chosen for multi-material printing. However, for the precise printing of the concave lenses, a Monochrome LCD Stereolithography (MSLA) printer, the PRUSA, was the preferred option. DLP printers offer rapid printing speeds but may compromise on surface finish. Therefore, they were selected for material optimisation projects where surface quality is not of paramount importance. Conversely, when it comes to printing lenses, precision takes precedence over speed, necessitating the use of a more accurate printing method.
To produce multimaterial samples through printing, the printer was halted during specific time intervals. Consequently, the build plate ascended, permitting manual access to the partially printed sample. The transparent liquid resin in the container was then extracted manually and substituted with the coloured dye resin. Simultaneously, efforts were made to eliminate any remnants of resin adhering to the sample that was affixed to the build plate. This meticulous cleaning was essential to prevent any amalgamation between the new resin introduced into the container and the remnants of the prior material. The method employed involved applying isopropyl alcohol (IPA) over the sample, followed by a thorough drying wipe. Following this, the printing process was recommenced, enabling the production of the sample composed of two distinct types of resin. Upon completion, the sample was detached from the build plate and subjected to a 20-min sonication process while immersed in IPA. This sonic treatment effectively eliminated any lingering traces of uncured resin that might have been adhering to the sample. The outcome of this process was evident in the enhanced smoothness and cleanliness of the sample’s surfaces. The meticulous sonication after printing yielded a significant enhancement in the optical transmission capabilities of the sample. This improvement was of paramount importance for applications necessitating optical precision, such as contact lenses. The printing procedure involves two stages of pausing and cleaning, with each stage lasting 5 min, contributing to a total of 10 min. For the clear resin, we printed 12 layers, with each layer taking 75 s, amounting to a total of 900 s. The central resin comprised 6 layers, each requiring 50 s to print, adding up to 300 s. The ring portion of the lens consisted of 12 layers, with each layer taking 20 s, resulting in 240 s. Summing these times, the overall printing duration was 2040 s, which translates to approximately 34 min per print. This time frame is optimised to balance efficiency with the intricate requirements of each lens component, ensuring the highest quality of the final product. While the Wanhao Duplicator 8 allows for printing up to 50 lenses simultaneously, we recommend printing only 4 lenses per batch. This recommendation is based on maintaining the necessary quality standards, given the specific requirements of cleaning and pausing involved in the printing process.
2.3. Characterisation
To assess the pH responsiveness of the printed disks, optical and morphological characterisation was conducted. Transmission and absorbance measurements were performed using an Ocean Optics UV-vis spectrophotometer (USB 2000+) in conjunction with the OceanView software. The recorded transmission and absorbance spectra were exported to MS Excel for graphing intensity (%) and optical density (OD), respectively, across a wavelength range of 400–800 nm. The pH-responsive behaviour of the disks was evaluated by observing the shift in intensity dip when they were immersed in different pH solutions prepared using commercial buffer solutions ((d–e)). Additionally, RGB plots were generated as graphical representations of colour data based on the additive RGB colour model.
To ensure accurate testing, thorough sample washing was crucial to eliminate potential confusion caused by excess uncured resin. For this purpose, DI water was selected over normal water or isopropyl alcohol. Samples were soaked in a pH 7.4 buffer solution to allow complete swelling before use. Orange samples resulting from the swelling process served as the baseline, contrasting with the reddish colour observed initially at a pH of 4.
To test for leakage, freshly printed samples were submerged in DI water for seven days, and transmission graphs were captured at specific intervals to monitor any changes. Swelling tests involved drying the samples, immersing them in water, and plotting the net weight increase due to absorbed water over time. The wettability of the surface was evaluated by carefully placing a droplet on a freshly printed sample, capturing images of the wetted surface, and employing open-source software (ImageJ) for further post-processing and contact angle measurement. A temperature-controlled magnetic stirrer bed was employed to raise the solution’s temperature to match the ocular surface temperature levels.
3. Results and discussion
3.1. Dye resin solutions
Dye resin solutions exhibiting different pH values (see ) demonstrate the remarkable potential of this dye in producing a wide spectrum of colours that are easily distinguishable by the naked eye. The transition from a deep reddish colour at acidic pH levels to lighter yellowish shades at alkaline pH values indicates the dye’s ability to effectively sense tear pH with a decent level of accuracy in real-time, eliminating the need for additional signal post-processing as required by other sensors documented in the literature [Citation19,Citation21]. This attribute simplifies the detection of early indications of ocular inflammations. The pH responsivity of Neutral red is attributed to its molecular structure and protonation/deprotonation processes. In its neutral form, Neutral red has a specific colour appearance. However, as the pH of the solution changes, the dye molecules either gain or lose protons, resulting in a shift in their electronic structure and subsequent alteration in colour. This phenomenon is known as chromophore protonation/deprotonation and is the basis for the dye’s pH-responsive behaviour.
The transmission graph further illustrates the shift in the dip’s position corresponding to each pH value, fulfilling the necessary criterion for developing colour filters aimed at addressing colour blindness by selectively blocking specific wavelengths. Consequently, it appears feasible to design the central part of the lens with an optimised resin pH to target individual patients with wavelengths specific to their colour vision deficiency. It is worth noting that TPO, is responsible for the low transmission observed in the range of 400–430 nm. Furthermore, the low transmission in the spectrum band 400–500 nm can be attributed to the high concentration of the dye powder, which is intended to enhance and ensure pH responsiveness and can be further optimised. However, in the region beyond 550 nm, the transmitted light exhibits significantly higher levels, reaching up to 90% transmission.
3.2. Optimisation of printing parameters
presents an optimisation study investigating the interplay between curing time, disk thickness, and the pH responsiveness of 3D printed samples. Disks of 12 mm diameter were fabricated with thicknesses of 0.3, 0.45, and 0.6 mm, subjected to varying curing times from 20 to 45 s, and then immersed in a pH 10 buffer solution to observe colour changes. The study utilised the Wanhao Duplicator 7 printer, chosen for its precision in 3D printing applications. The results indicated that the 0.3 mm disks, while exhibiting rapid pH response, were mechanically fragile and prone to deformation, reducing their practicality. Conversely, the 0.6 mm disks showed greater structural integrity but had a slower pH response. The 0.45 mm disks displayed a balance between responsiveness and durability, suggesting an optimised compromise for practical use.
Figure 2. Optimisation of curing time, thickness, and pH responsiveness for disks with various thicknesses: (a) 0.3 mm, (b) 0.6 mm, and (c) 0.45 mm. Scale bar = 20 mm.
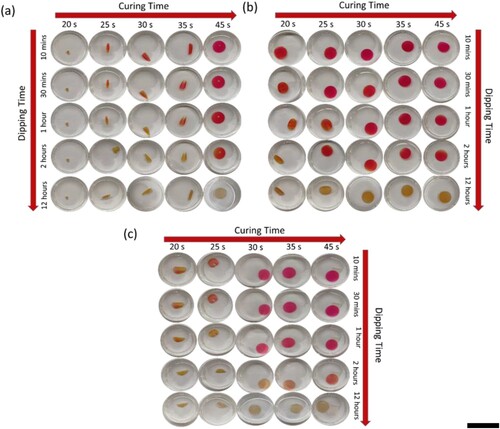
Analysis of the curing process revealed a clear trend: increased curing times lead to reduced porosity due to more extensive photopolymerization, thereby diminishing pH sensitivity. This finding is pivotal for applications in colour blindness correction, where stable, non-reverting colour properties are essential. For such applications, a longer curing time is recommended to ensure the stability of the coloured resin, which can be achieved by sandwiching the dyed resin between clear layers. In contrast, for pH sensing where quick responsiveness is crucial, a sparsely cured layer is advantageous, allowing for higher porosity and rapid detection of pH alterations, which is critical for timely user response.
3.3. pH sensor evaluation
To enhance the reliability of the pH sensor, the MSLA 3D printer (PRUSA) was used in this research due to its exceptional capability of producing thin disks with a precision of 200 µm or even less thickness wise. Building upon the optimisation experiments conducted earlier, the focus shifted towards developing thinner disks for applications requiring fast response times. The baseline for the disks was established by immersing the freshly printed samples in a buffer solution of pH 7, to simulate the normal tear pH range of 6.8–7.4. Ensuring full swelling of the disks was crucial to obtain accurate results in subsequent tests, thus the orange-coloured disks were considered the original state before conducting the sensor limitation test.
For the evaluation of the sensor, the orange disks were subjected to various pH buffers, and measurements of colour, transmission, and absorbance graphs were simultaneously recorded at specific time intervals. Notably, a significant change in the graphs was observed within the first 30–60 min of the immersion period, indicating a rapid response (). However, the subsequent plots from 1 to 2 h showed less noticeable variations. This suggests that a duration of approximately 1 h is sufficient for the sensor to reach saturation. Furthermore, the established dips in the transmission spectra for each pH sample can be utilised as effective colour filters for addressing colour blindness, highlighting the potential for customisation and tunability of the hydrogel resin, based on the severity of individual patients.
Figure 3. Sensor saturation in various pH buffers. Scale bar = 10 mm (a) 3D printed samples change colour across time intervals. Transmission and absorbance graphs recorded after (b) 30 min, (c) 1-hour, and (d) 2-hour.
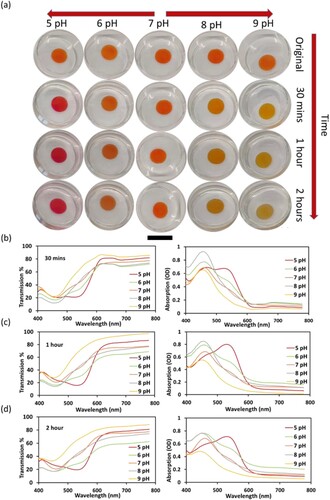
The observed shifts in the transmission dip for each pH sample further emphasize the capability to tailor the sensor to specific patients’ needs. This provides a valuable opportunity to optimise the sensor’s performance by adjusting the wavelength blockage to match the problematic colour perception of each individual. This customisation approach holds promise for improving the effectiveness and personalised experience of the sensor for users with colour vision deficiencies.
presents a comprehensive analysis of colour change kinetics and spectral properties of the samples, as referenced in , using the RGB colour model. The top panel (a) showcases the time-dependent colour response of the samples at different time intervals: half an hour (i), one hour (ii), and two hours (iii), across a pH range of 5–10. The red, green, and blue lines represent the respective RGB components, indicating the degree of colour change. Notably, the results demonstrate a significant trend towards colour saturation after one hour, with little to no progression observed after two hours. This finding suggests that a one-hour interval is optimal for achieving full colour change, marking an efficient point for potential practical applications.
Figure 4. Analysis of sensor change over time intervals. (a) Colour change measurements repeated across multiple samples. (b) Colour analysis using RGB model for three intervals. (i) 30 min. (ii) 1 h. (iii) 2 h. (c) The dip position and intensity recorded in the transmission spectra for the 1-h dipping interval.
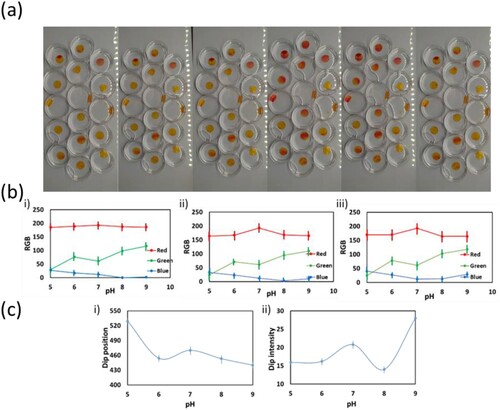
The bottom panel (b) delves into the spectral characteristics of the samples, with graph (i) illustrating the shift in the wavelength dip position within the transmission spectrum across the same pH range. This shift is crucial, as it confirms the samples’ capability to span the necessary spectral regions for effective colour correction – a feature particularly relevant for colour blindness correction technologies. Graph (ii) complements these findings by displaying the transparency profile against varying pH levels, affirming that the samples achieve a sufficient blocking rate of light in key regions, which is essential for the targeted filtering of wavelengths.
Statistical rigour is evident in the incorporation of standard deviation bars in the RGB analysis, indicating consistency across the 50 samples tested (a). The error bars underscore the reliability of the data, with a narrow spread denoting high precision in the observed colour responses. Such statistical validation, combined with the significant colour change within the first hour, underscores the effectiveness and potential of these samples for real-world applications in colour vision enhancement or correction.
Repeatability and reversibility tests were conducted to assess the performance of the sensor in terms of its ability to return to its original state and position after cycling between pH 4 and pH 10 (). The sample was initially saturated in a buffer of pH 4, then it was immersed in a pH 10 buffer to observe the change over time. After reaching saturation, the sample was carefully cleaned to prevent cross-contamination and then placed in a pH 4 buffer. It was observed that the sample fully recovered its original reddish colour, confirming the reversibility of the sensor.
Figure 5. Reversibility and repeatability tests. (a) Reversibility tests in buffers of pHs 4 and 10. Scale bar = 10 mm (b) Transmission and absorbance spectra for the sample after 3 cycles. (c) Dip intensity and position change after each cycle.
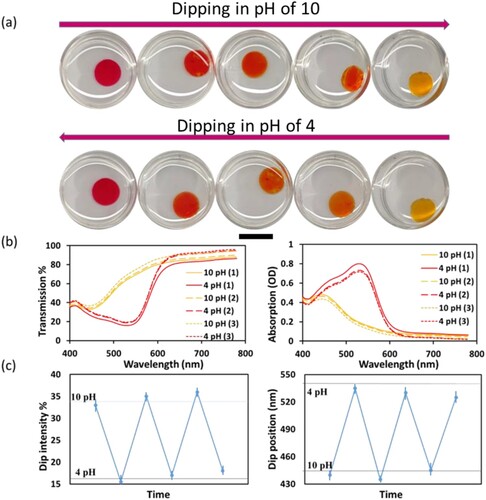
To evaluate the repeatability, the cycle of pH 4 to pH 10 and back was repeated three times, while the transmission and absorbance data were recorded for analysis. A slight upward shift in transmitted light intensity was observed, indicating sufficient repeatability (b). However, the complete recovery and return to the original state were not achieved, which can be attributed to several factors. c illustrates the ability of the samples to revert to their initial state, within an acceptable margin of error concerning intensity and wavelength dip position.
Chemical degradation or changes in the molecular structure of the neutral red dye can occur when exposed to extreme pH values repeatedly [Citation15]. These changes can lead to alterations in the dye’s absorption and transmission properties, resulting in deviations from the original transmission graph. Additionally, residual retention of dye molecules within the sensor material or matrix may hinder their complete return to the initial configuration during subsequent pH cycles. This cumulative effect of dye retention can gradually impact the transmission properties of the sensor. Moreover, the repeated cycling between pH 4 and pH 10 can lead to sensor fatigue or wear, particularly under harsh conditions over an extended period. Continuous exposure to extreme pH values can cause physical or chemical changes in the sensor material, such as surface degradation, alteration of the sensor structure, or contamination, all of which contribute to the incomplete recovery of the transmission graph. Regular calibration and validation of the sensors are essential to understand the magnitude of deviations from the expected transmission graph. These practices enable appropriate adjustments or corrections to be made during data interpretation and ensure the reliability and accuracy of the pH sensor.
To ensure the stability of the dye within the matrix and to prevent any potential leakages in the tear fluid, a comprehensive leakage test was conducted (). The samples were submerged in DI water for 7 days, and both visual observations and transmission graphs were analysed for any noticeable changes. As DI water lacks ions that could affect the colour of the samples, it was selected as the testing medium. The initial results of the samples remained relatively consistent during the first day, with slight variations observed after a week. The upward shift in the transmission data can be attributed to several factors, including the interactions between the dye and water molecules, the swelling behaviour of the disk, and surface effects. It is crucial to note that this upward shift in the transmission data does not necessarily indicate instability or degradation of the dye. To further confirm the stability of the dye, additional tests were performed, including the reversibility test, which involved examining the transmission graphs. The results of the reversibility test indicated that while there was a slight change in the transmission rate during the first half of the first cycle, no significant changes were observed during the second half. This suggests that the slight change in transmission was likely due to excess resin that was not fully washed out, which was not the case in subsequent cycles. The water content test demonstrated a gradual increase in water absorption over the 48-hour duration, starting at 20% and reaching 30% within the first six hours, ultimately reaching 35% at the end of the test. These results indicate favourable water absorption and retention properties of the sample, showcasing its ability to effectively hydrate and retain moisture. Additionally, the wettability test for our contact lenses, represented by an average contact angle of 53 degrees from 5 samples with a controlled droplet size of 1 microliter, indicates moderate wetting. This level of wettability, crucial for maintaining a stable tear film, strikes a balance between hydrophobic and hydrophilic properties, aligning with values reported in literature for high-quality contact lenses. Such a balance is key for ensuring wearer comfort, minimising the risks of dry eyes or irritation, and maintaining clear vision. The moderate contact angle suggests that our lenses effectively interact with tear fluid, avoiding issues associated with overly hydrophobic or hydrophilic surfaces, such as poor lens wetting or excessive tear film breakup. This balanced wettability is essential for preventing protein and lipid deposits on the lens, which can degrade vision quality over time. Overall, our results indicate that these lenses can provide a stable tear film and consistent vision, which are critical for comfortable and prolonged wear, as supported by comparable values found in existing literature [Citation27,Citation28].
Figure 6. Dye stability and surface interaction towards wetting. (a) Printed sample’s reaction towards DI water dipping. Scale bar = 10 mm. (b) Transmission spectra for samples after 7 days of DI water dipping. (c) Transmission spectra for buffer solutions after reversibility test. (d) Droplet contact angle. (e) water absorptivity after 48 h.
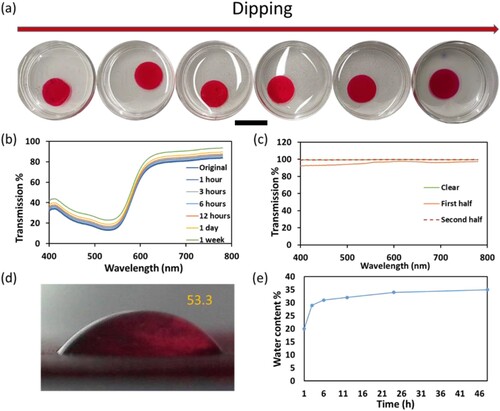
The exploration of the dye’s reversibility across an extensive pH range has provided us with a foundational understanding of its responsiveness and the dynamics of its colour-changing behaviour. Building on this, our focus has shifted to a narrower, yet highly significant, pH range of 6–8 – corresponding to that of human tears. By conducting experiments in a tear solution at 37°C modified with buffer solutions made of the same solution to reach the desired pH levels, we have not only replicated the conditions within the human eye but have also observed a rapid colour change within 30 min as shown in . This observation is pivotal, as it suggests that the dye’s sensitivity is finely tuned enough to detect subtle pH variations that could be critical in ocular health monitoring.
Figure 7. Comprehensive Evaluation of Dye Performance in a Simulated Ocular Environment. Scale bar = 10 mm (a) Progressive colour change in samples cycled through a pH range of 6–8 made of an artificial tear solution (Renu) modified with pH buffers made of the same solution. (b) Transmission and Absorbance spectra at the pH endpoints and the midpoint. (c) Samples on hot plate using tear-like solution, Renu.
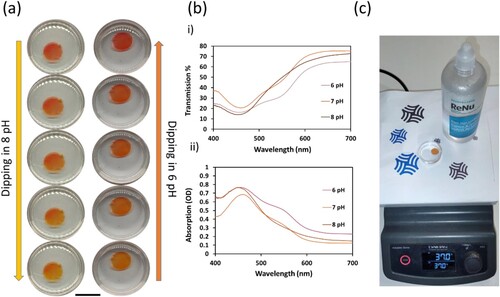
Moreover, the stability of the dye over a three-day period in such a biologically relevant setting warrants discussion. It raises important considerations regarding the longevity and reliability of potential pH sensing lenses that could be developed using this dye. The absence of significant colour change over this duration indicates a resilience that is indispensable for any continuous monitoring device intended for application in a dynamic biological environment such as the eye. This balance of rapid responsiveness, coupled with stability, positions the dye as an intriguing prospect for future development in the field of non-invasive diagnostic and health monitoring technologies.
3.4. Multifunctional lenses
The final stage of the study involved the fabrication of a multi-material lens, which required a systematic approach considering the sensor’s established capabilities and the dye’s colour-filtering properties (). The fabrication process commenced by 3D printing the clear part of the lens, followed by the addition of the selected resin for the specific colour filtering component in the central part. Sufficient curing was applied to ensure optimal performance (i.e. overexposure to achieve a slow pH response). Additionally, the dye resin was incorporated as an outer radial ring, with a shorter curing time, enabling enhanced pH sensing as previously investigated. To maintain the integrity of the central part and to preserve its colour-filtering functionality, an additional layer of clear resin was applied, effectively encapsulating the central part. This protective layer prevented any alteration in colour caused by tear impact and ensured sustained colour filtering performance (a). Subsequently, the printed lens was immersed in a buffer of pH 10 to evaluate its application (c). As anticipated, the ring region exhibited the expected shift towards a yellowish colour, indicating a successful pH response, while the central part retained its original colour, specifically designed to block the wavelength range of 530–560 nm (e). This outcome validates the successful integration of colour filtering and pH sensing functionalities within the multimaterial and multifunctional 3D printed lens design.
Figure 8. 3D printing multimaterial and multifunctional lenses. (a) Multimaterial 3D printing schematic. (b) 3D printed samples using multi-material techniques. (c) The sample dipped in a buffer of pH 10. (d) The sample fixed on an eye model. (e) Lens imaged in between the opposite pH limits. (f) Leeching test of multimaterial lens in tear-like solution (Renu).
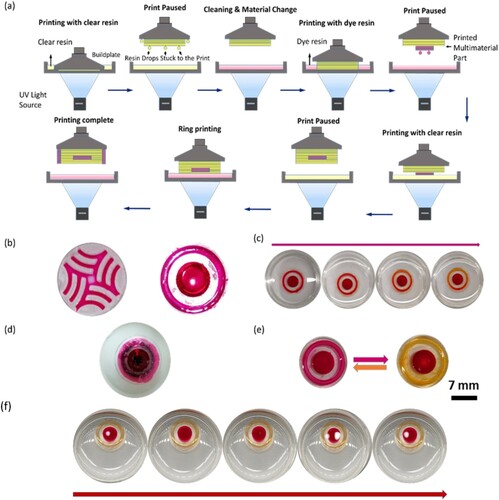
4. Conclusion
This study has successfully demonstrated the development of multimaterial and multifunctional contact lenses that integrate tunable colour filtering and tear pH sensing capabilities. These lenses can provide personalised colour vision correction and enable the monitoring of ocular surface health. By utilising 3D multimaterial printing technology and incorporating a pH-responsive dye, we have achieved rapid pH responsiveness, noticeable colour shifts, and favourable water absorption properties in the lenses.
The integration of colour filtering and pH sensing functionalities within a single lens offers a convenient and efficient solution for individuals with colour vision deficiencies and those requiring regular tear pH monitoring. The lenses can be tailored to address the specific needs of each individual, improving colour perception and facilitating early detection of ocular health issues such as dry eye syndrome and inflammation.
Acknowledgment
The authors acknowledge Sandooq Al Watan LLC for the research funding (SWARD Program—AWARD, Project code: 8434000391-EX2020-044). We also acknowledge Khalifa University for the Advanced Digital and Additive Manufacturing Center (ADAM) research funding (Award No. RCII-2019-003) in support of this research.
Data availability
The raw/processed data required to reproduce these findings cannot be shared at this time as the data also forms part of an ongoing study.
Disclosure statement
No potential conflict of interest was reported by the author(s).
Correction Statement
This article has been corrected with minor changes. These changes do not impact the academic content of the article.
Additional information
Funding
References
- Salih AE, Mohamed E, Murad A, et al. Ophthalmic wearable devices for color blindness management. Adv Mater Technol. 2020;5(8):1901134. doi:10.1002/admt.201901134
- Alam F, Salih Ahmed E, Mohamed E, et al. 3D printed contact lenses for the management of color blindness. Addit Manuf. 2022;49:102464. doi:10.1016/j.addma.2021.102464
- Alam F, Salih AE, Elsherif M, et al. Development of 3D-printed glasses for color vision deficiency. Adv Eng Mater. 2022;24(10):2200211. doi:10.1002/adem.202200211
- Salih AE, Elsherif M, Alam F, et al. Gold nanocomposite contact lenses for color blindness management. ACS Nano. 2021;15(3):4870–4880. doi:10.1021/acsnano.0c09657
- Tian Y, Tang H, Kang T, et al. Inverse-designed aid lenses for precise correction of color vision deficiency. Nano Lett. 2022;22(5):2094–2102. doi:10.1021/acs.nanolett.2c00262
- Bleau M, Jaiswal A, Holzhey P, et al. Applications of additive manufacturing, or 3D printing, in the rehabilitation of individuals with deafblindness: a scoping study. SAGE Open. 2022;12(3):215824402211178. doi:10.1177/21582440221117805
- Schornack MM, Brown WL, Siemsen DW. The use of tinted contact lenses in the management of achromatopsia. Optometry. 2007;78(1):17–22. doi:10.1016/j.optm.2006.07.012
- Salih AE, Shanti A, Elsherif M, et al. Silver nanoparticle-loaded contact lenses for blue-yellow color vision deficiency. Physica Status Solidi (A). 2022;219(1):2100294. doi:10.1002/pssa.202100294
- Lee JH, Kim H, Hwang J-Y, et al. 3D printed, customizable, and multifunctional smart electronic eyeglasses for wearable healthcare systems and human–machine interfaces. ACS Appl Mater Interfaces. 2020;12(19):21424–21432. doi:10.1021/acsami.0c03110
- De Santis R, D’Amora U, Russo T, et al. 3D fibre deposition and stereolithography techniques for the design of multifunctional nanocomposite magnetic scaffolds. J Mater Sci: Mater Med. 2015;26:1–9. doi:10.1007/s10856-015-5582-4
- Ronca D, Langella F, Chierchia M, et al. Bone tissue engineering: 3D PCL-based nanocomposite scaffolds with tailored properties. Procedia Cirp. 2016;49:51–54. doi:10.1016/j.procir.2015.07.028
- Amendola V, Pilot R, Frasconi M, et al. Surface plasmon resonance in gold nanoparticles: a review. J Phys: Condens Matter. 2017;29(20):203002. doi:10.1088/1361-648X/aa60f3
- Karimi S, Moshaii A, Abbasian S, et al. Surface plasmon resonance in small gold nanoparticles: introducing a size-dependent plasma frequency for nanoparticles in quantum regime. Plasmonics. 2019;14:851–860. doi:10.1007/s11468-018-0866-4
- Saboo US, Amparo F, Abud TB, et al. Vision-related quality of life in patients with ocular graft-versus-host disease. Ophthalmology. 2015;122(8):1669–1674. doi:10.1016/j.ophtha.2015.04.011
- Proksch E. pH in nature, humans and skin. J Dermatol. 2018;45(9):1044–1052. doi:10.1111/1346-8138.14489
- Callejo G, Castellanos A, Castany M, et al. Acid-sensing ion channels detect moderate acidifications to induce ocular pain. Pain. 2015;156(3):483–495. doi:10.1097/01.j.pain.0000460335.49525.17
- Rouen PA, White ML. Dry eye disease: prevalence, assessment, and management. Home Healthc Now. 2018;36(2):74–83. doi:10.1097/NHH.0000000000000652
- Tuft S, Somerville TF, Li J-PO, et al. Bacterial keratitis: identifying the areas of clinical uncertainty. Prog Retinal Eye Res. 2022;89:101031. doi:10.1016/j.preteyeres.2021.101031
- Chen FS, Maurice DM. The pH in the precorneal tear film and under a contact lens measured with a fluorescent probe. Exp Eye Res. 1990;50(3):251–259. doi:10.1016/0014-4835(90)90209-D
- Moreddu R, Wolffsohn JS, Vigolo D, et al. Laser-inscribed contact lens sensors for the detection of analytes in the tear fluid. Sens Actuators, B. 2020;317:128183. doi:10.1016/j.snb.2020.128183
- Riaz RS, Elsherif M, Moreddu R, et al. Anthocyanin-functionalized contact lens sensors for ocular pH monitoring. ACS Omega. 2019;4(26):21792–21798. doi:10.1021/acsomega.9b02638
- Koçtekin B, Gündoğan NU, Altıntaş AGK, et al. Relation of eye dominancy with color vision discrimination performance ability in normal subjects. Int J Ophthalmol. 2013;6(5):733.
- Lopes L, Silva A, Carneiro O. Multi-material 3D printing: the relevance of materials affinity on the boundary interface performance. Addit Manuf. 2018;23:45–52. doi:10.1016/j.addma.2018.06.027
- Sitthi-Amorn P, Ramos JE, Wangy Y, et al. Multifab: a machine vision assisted platform for multi-material 3D printing. ACM Trans Graph. 2015;34(4):1–11. doi:10.1145/2766962
- Hisham M, Salih AE, Butt H. 3D printing of multimaterial contact lenses. ACS Biomater Sci Eng. 2023;9(7):4381–4391.
- Dalton K, Subbaraman LN, Rogers R, et al. Physical properties of soft contact lens solutions. Optom Vis Sci. 2008;85(2):122–128. doi:10.1097/OPX.0b013e318162261e
- Ma L, Li K, Xia J, et al. Commercial soft contact lenses engineered with zwitterionic silver nanoparticles for effectively treating microbial keratitis. J Colloid Interface Sci. 2022;610:923–933. doi:10.1016/j.jcis.2021.11.145
- Khan SA, Shahid S, Mahmood T, et al. Contact lenses coated with hybrid multifunctional ternary nanocoatings (Phytomolecule-coated ZnO nanoparticles:Gallic Acid:Tobramycin) for the treatment of bacterial and fungal keratitis. Acta Biomater. 2021;128:262–276. doi:10.1016/j.actbio.2021.04.014