Abstract
Biomimicry is the application of existing features in nature to human technologies, such as the invention of aircraft inspired by bird flight. In the development of medical solutions, biomimicry is a growing field of research, where a holistic understanding of nature can inspire cutting-edge design. The purpose of this study was to create an educational, visual resource exemplifying up-and-coming medical applications of biomimicry. A website was created to present 2D motion graphics (animations) and illustrations. Animation is an established and useful method of communicating health information to the public. This presents an accessible interface for the public to interact with and learn about this area of research, bridging the gap between the two. Increasing public knowledge, engagement, and interest can expand the reach and thereby influence future research. A survey was conducted to assess public engagement and opinions on both the resource and the topic of biomimicry and medical design. The results suggested that participants positively engaged with the resource; 95.7% strongly agreed/agreed that the animations were beneficial for learning. All responding participants agreed that biomimicry could provide useful solutions in medical design. This study suggests that graphic motions are effective at communicating complex ideas for public outreach.
Introduction
This project was undertaken as part of a master’s research project at the University of Dundee. The objective was to create a visual resource for the public on the topic of biomimicry applications in medicine. A feedback survey was carried out to assess public interest in the topic, engagement with the resource and the visual appeal of the animations and illustrations.
Literature review
This review investigated applications of biomimicry in medicine. Five examples were selected for the review focus, which included applications based on features of interest from parasitic wasps, mosquitoes, weakly electric fish, sharks, and cephalopods. The sections below describe the background research for each application (tables further summarising the development history that led to each design are included in the supplementary materials to this article), followed by a concluding section highlighting the benefits of visual resources and public outreach in science and medicine.
Parasitic wasps and tissue transportation
Tissue extraction from the human body is an important procedure in many fields of surgery, including for biopsy or removal of diseased, necrotic, or infected tissues during open or minimally invasive surgery (MIS). Tissue extraction from deeper locations in the body can be more challenging and may involve the use of surgical instruments, aspiration-based devices, or stent-retrievers (Sakes et al., Citation2020).
The egg-laying transport system of female parasitic wasps has inspired a number of prototypes for tissue transportation devices (Ma et al., Citation2022; Sakes et al., Citation2020). These wasps have an ovipositor, a ‘stinger-like appendage’, used to drill into bark, plant tissue and other insects, before depositing eggs (van Meer et al., Citation2020). Its ability to steer through different materials without buckling has motivated engineers to apply its mechanics to technology (Cerkvenik et al., Citation2017; Ma et al., Citation2022; Scali et al., Citation2019). The ovipositor is made up of three valve-like parts (Cerkvenik et al., Citation2017) that are thought to move reciprocally, alternating between protraction and retraction. The friction created by the sliding of valves pushes the eggs through the lumen. This sliding mechanism is the basis for prototype mechanics (Sakes et al., Citation2020).
The biomedical engineer, Dr Marta Scali, has been involved in several studies and the production of prototypes. This work was carried out through Delft University of Technology and includes a PhD thesis (Scali, Citation2020). Scali’s thesis (2020) and the corresponding study by Sakes et al. (Citation2020) put forth the most recent prototype design. The prototype () is made up of six semi-cylindrical valves, held together by an outer tube and actuated by an electromotor. As this represents the most up-to-date design, similar to how final products may work, it was selected as a visual reference for the graphics.
Figure 1. Tissue transportation prototype by Marta Scali (Sakes et al., Citation2020).
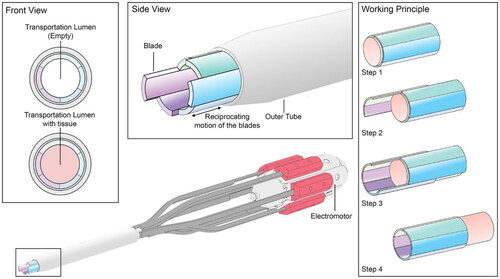
An ovipositor-inspired device could potentially be used for both diagnostic tissue sampling and drug delivery in MIS. In the future, longer-length devices designed with mechanics to overcome challenges like buckling may be able to reach deeper targets inside the body than those currently available (Scali et al., Citation2019).
Mosquitoes and percutaneous needle designs
Percutaneous means ‘performed through the skin’ (Cambridge Dictionary, 2023). Percutaneous needles are typically used during procedures such as biopsies and blood sampling (Ma et al., Citation2022). Several insect-based needle designs have been described in the literature, and are typically based on insect mechanisms, behaviours, shapes, and patterns. As identified in a review by Ma et al. (Citation2022), some common design objectives for needles based on insect capabilities include:
Minimising insertion force or puncture force
Increasing the manoeuvrability of the needle
Enhancing the adhesion ability of the needle
Reducing tissue deformation and strain
Current challenges with instrumentation used intraoperatively and areas for development, as outlined by Frasson et al. (Citation2010), are depicted in , alongside the potential benefits identified from insect-based designs (mosquitoes, wasps, honeybees, etc.).
The proboscis describes the mouthpart of mosquitoes. In females, it is used to penetrate human skin and to draw blood (needed for nourishment and egg production). It is a sophisticated component made up of six needles (stylets). Drawing of blood using the proboscis is practically painless. The stylets comprise two maxillae, two mandibles, a labrum, and a hypopharynx. The maxillae act as microsaws; they are sharp and small with serrated surfaces (Mosquito Magnet, Citation2020).
A review by Ma et al. (Citation2022) identified nine studies inspired by mosquitoes. Most studies replicated either the pattern or vibration of the mosquito’s proboscis. This review described how a needle’s insertional force has often been related to its potential ‘painfulness’, as well as being a cause of ‘mechanical damage in the tissue’ in other literature. Therefore, mimicking biomimetic patterns may be a favourable tactic to reduce the insertional force (Ma et al., Citation2022). The jagged surface of the maxillae has been replicated in several studies (Dixon & Vondra, Citation2022; Ma et al. Citation2022). Yang and Zahn (Citation2004) demonstrated that insertional force was further reduced by incorporating vibration. The final illustrations were created with reference to recent studies and prototypes from Gidde and Hutapea (Citation2021) and Li et al. (Citation2020). An image from Gurera et al. (Citation2018) was also utilised as it demonstrates a device closely resembling the proboscis. presents the graphic created based on these references.
Figure 3. Graphical representation of a mosquito inspired needle (prototype design) based on Gidde and Hutapea (Citation2021), Gurera et al. (Citation2018), and Li et al. (Citation2020).
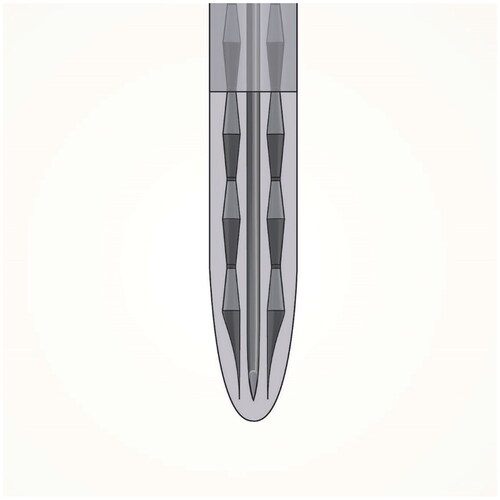
Weakly electric fish and endovascular treatment
Treatment and diagnosis of vascular disease often require navigation through the vascular tree via a catheter device. Currently, navigation of interventional devices endovascularly (within blood vessels) relies on multiple fluoroscopic images, the use of guide wires, and the formation of a plan based on pre-interventional images (Fuerst et al., 2016). Anatomical variances and reliance on two-dimensional images can make these procedures more challenging and time-consuming. An example of a condition requiring endovascular treatment is atrial fibrillation, which causes the heart to beat irregularly due to disruption of the electrical signals regulating it. The treatment, radiofrequency ablation, involves guidance and positioning of a catheter. Once in position, electrodes emit radio energy from the catheter’s tip to a target site of heart tissue – this destroys the tissue causing the irregularity (MedStar Health, Citation2023).
Studies have investigated the navigational techniques of weakly electric fish that could be applied to reduce the need for fluoroscopy and provide the interventionalist with a better understanding of the catheter’s surroundings. Weakly electric fish form an ‘electric image’ of their surroundings for navigation, through a process known as electrolocation (Zakon & Smith, Citation2002). They can be described as being both electrogenic and electroreceptive, as they produce and detect electric signals. They have an electric organ, which emits electric signals to generate an electric field (von der Emde, Citation1999). Electrical impedance: the ‘measure of the total opposition that a circuit or a part of a circuit presents to electric current’ (Britannica, Citation2023), can be considered a type of resistance and is an important factor in electro-reception. The electrical impedance of surrounding objects causes alteration to the electric field. The fish are able to measure the resulting changes to amplitude and phase to help detect objects and to determine an object’s ‘electrical and geometrical properties’ (von der Emde et al., Citation2009).
Boston Scientific has developed a navigation system called DIRECTSENSE™ Technology inspired by weakly electric fish. They have added electrodes to their existing radiofrequency ablation catheters. The study by Fuerst et al. (2016), a dissertation by Sutton (Citation2017) and a resulting study by Sutton et al. (Citation2020) describe research into similar technologies inspired by the fish. Sutton (Citation2017) and Sutton et al. (Citation2020) describe the theory behind this technology; it works through the creation of a weak electric field via electrodes on the catheter tip. Impedance is measured as it changes throughout the vascular pathway. Finally, the ‘impedance time series is then mapped to a preoperative vessel model to determine the relative position of the catheter within the vessel tree’ (Sutton, Citation2017). It can be used to identify bifurcations, stenoses and aneurysms in the catheter’s path. Reference was made to these studies and DIRECTSENSE™ Technology for the resource visuals ().
Sharks and antibacterial infection control
Biofouling is the adhesion and accumulation of micro- and macro-organisms (Hussein, Citation2023). It can occur on the surfaces of products that are placed inside the body and infection can develop (Reddy et al., Citation2011). Some examples of device types associated with biofilm infections include orthopaedic alloplastic devices, endotracheal tubes, intravenous catheters, and indwelling urinary catheters or urethral stents (Høiby et al., Citation2015). Biofouling can lead to the spread of infectious diseases, implant rejection or device malfunction. Currently, biofouling is managed by removing and replacing implants and devices, which can be costly and require invasive surgery. Changing the structural surface of such products to render them antibacterial may reduce infection incidence in the future. Hospitals and other healthcare environments pose a risk for spreading infection (healthcare-associated infection). This is concerning with the increasing problem of antibiotic resistance (Centers for Disease Control and Prevention [CDC], 2019). Methicillin-resistant Staphylococcus aureus (MRSA) can be prevalent in hospital settings and cause severe sickness.
Shark skin is covered with millions of dermal denticles; these are micro-scale placoids, also known as ‘dermal teeth’. They create a rough surface made up of grooves and ridges, a surface that is difficult to adhere to, preventing adhesion of micro-organisms to the skin. Chien et al. (Citation2020) found that ‘the microscale topography of shark skin slightly promoted bacterial attachment at an early stage but prevented bacteria from developing biofilms’.
A company called Sharklet Technologies, Inc. has developed a ‘sharklet’ micropattern inspired by dermal denticles that deters bacteria. Furthermore, bacteria that do attach to it are less likely to grow as the ridges prevent bacteria from linking and colonising the surface (Allerton, Citation2023; Mann et al., Citation2014). This product is the reference for the final illustrations (). Some up-and-coming applications were also explored. Mann et al. (Citation2016) described the development and testing of a micropatterned endotracheal tube. Endotracheal tube biofilms represent a major culprit in the development of ventilator-associated pneumonia (Alves et al., Citation2023). An earlier study by Mann et al. (Citation2014) looked at the effectiveness of the sharklet micropattern for deterring both MRSA and methicillin-sensitive S. aureus (MSSA). They compared it to other surfaces and the results showed that it was more effective.
Figure 5. Graphical representation of a shark inspired micropattern based on Mann et al. (Citation2016).
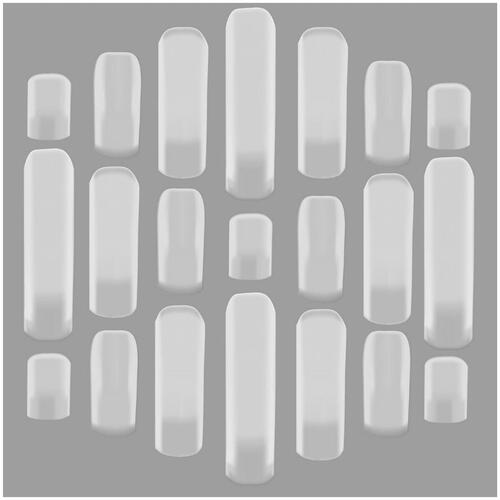
Future uses of the micropattern include structural changes, or alternatively, application of micropattern adhesives to surfaces in healthcare settings. This could be concentrated on surfaces at higher risk for spreading infection (MediPal, Citation2022).
Controlled adhesion for material transportation and cephalopods
Transportation of small, delicate components, such as ‘“living” cell sheets or bioelectronic chips’, during medical procedures can pose some difficulty and be time-consuming. Currently, this may involve the use of instruments (such as forceps) which are not always ideal and require a high level of dexterity from the interventionalist. Handling of such parts must be precise to avoid damage, deformation, and wrinkling (Kim et al., Citation2020).
Cephalopods (octopuses, squids, and cuttlefishes) have suction cups across their arms and tentacles. They consist of a ‘dense sheet of radial muscles and rings of circular and meridional muscles’, which are covered by ‘sheets of connective tissue and epithelia’ (Meyers, Citation2006). When the suction cup comes into contact with an object, it presses flat against it. Bioelectrical signals cause rapid contraction of the radial muscles. The muscles protrude, causing the circumference of the infundibulum to increase and the volume or space in the acetabulum to increase also. Water trapped in the acetabulum leads to a decrease in the internal pressure, resulting in higher pressure outside the cup than on the inside. This large pressure differential creates a seal between the suction cup and the object. Relaxation of the radial muscles and contraction of the circular and meridional muscles releases the grip (Kier & Smith, Citation2002; Meyers, Citation2006). There has been research into applying the same methods of attachment to medical devices and adhesives.
For this project, the transportation prototype described by Kim et al. (Citation2020) was selected as it represents one of the most recent studies with promising results and several applications. This device mimics the suction cup not by its shape and structure, but in the shift in pressure that occurs internally to create a seal. Below its base are two layers held together by a cyanoacrylate adhesive – a micro-electric heater and a micro-channelled hydrogel. The heater ‘converts electrical signals into heat’ and ‘small temperature changes’ are applied to the hydrogel. This causes rapid changes in the internal pressure of the gel as the micro-channels expand and contract in response to the heat. As the gel is heated, the micro-channels shrink, and as it cools, they expand. As the channels expand, this causes a decrease in the internal pressure. This means it can attach to objects with minimal external pressure being applied. The results of this research showed that the device can successfully attach to, lift, and detach from objects in 10–30 s, depending on the material and other variables. The prototype still requires further study and wider-scale testing to ensure its efficacy and safety. Potential applications listed by the researchers include its use as ‘a new soft handling tool for the fabrication of ultrathin film devices, tissue engineering, and transplant surgery’ (Kim et al., Citation2020). presents the graphic created with reference to the prototype by Kim et al. (Citation2020).
Figure 6. Graphical representation of an electrothermal soft manipulator based on Kim et al. (Citation2020).
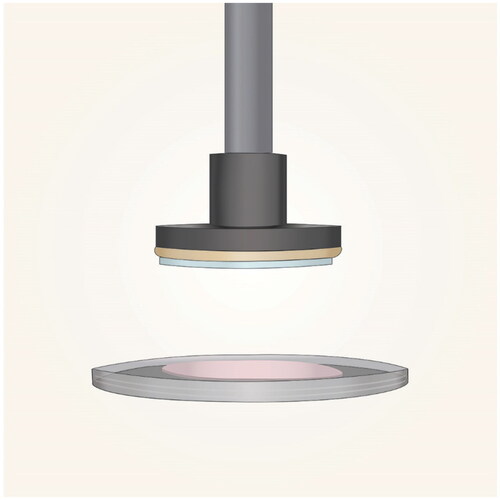
The benefits of visual resources and public outreach in science
Engaging the public with medical and scientific research empowers and gives greater impact to research. Dissemination of research can improve public health knowledge and outcomes (Sykes et al., Citation2022). Ideally, information should be accessible and open to everyone. Several studies have investigated public outreach in science and visual methods of communication (animations, comics, presentations etc.; Finkler & Leon, Citation2019; Leiner et al., Citation2004; Sykes et al., Citation2022; Tatalovic, Citation2009; Zimmerman et al., Citation2006). These are often limited and require further large-scale studies with control groups to define the most effective methods (Knapp et al., Citation2022; Moe-Byrne et al., Citation2022). Public outreach has been cited as being important to gain funding for research and for public engagement (Andrews et al., Citation2005; Stilgoe et al., Citation2014). Digital media is an exciting method to reach wider audiences and connect people; it is also cost-effective. In science, it provides a way to visualise an idea that may otherwise appear abstract (Trumbo, Citation2000). Animation, in particular, can be a useful means of relating health information to the public, with positive outcomes reported such as reduced alcohol use and increased awareness of COVID (Sykes et al., Citation2022). Finkler and Leon (Citation2019) cited realism, credibility, and characters that the audience identifies with as important features for videos/animations. They described their ‘SUCCESS’ method for communicating ethical whale watching to the public as merging ‘scientific literature’ and ‘marketing communication’ (Finkler & Leon, Citation2019). The results of their study suggested that this videography method was potentially very effective. Their method centres around having a story the audience understands and that holds their attention. Connecting the topic to the audience is important; the subject should be somewhat familiar. The story must also be memorable and give the audience hope by showing solutions.
Sykes et al. (Citation2022) created two animations targeted at healthcare leaders on the topic of hospital audits. Their findings suggested that the animations increased interaction with related research articles, and they hypothesised that ‘the use of animation distributed via Twitter may provide an effective way to disseminate research findings and increase stakeholder engagement with study findings’ (Sykes et al., Citation2022).
Some reviews of studies on the use of animations for health education describe potential limitations, including holding the audience’s attention and the risk of only providing ‘surface-level learning’ (Knapp et al., Citation2022; Moe-Byrne et al., Citation2022). Animation style and quality may also impact learning and more research is required on this.
Materials and methods
The methodology used for creating the resource, including the ethical approval process and the feedback survey are described below.
The resource
Motion graphics were created to translate for viewers the sometimes-complex mechanisms adapted from nature for technological use. The intent was to make it easy to compare and visualise the relationship between the natural and medical mechanisms. Longer, more in-depth animations were made for the parasitic wasp and weakly electric fish examples. Shorter animations were used for the other animals, presenting the underlying mechanism (from nature) and device design in separate interactive segments. The ‘SUCCESS’ method described by Finkler and Leon (Citation2019) was applied where possible – the overall idea was to show viewers something recognisable from nature, e.g., an octopus, and then relate it to a scientific concept (that may have previously been unknown to them). References were provided to give the animations credibility.
The longer animations included the animal in its natural setting, followed by a close-up of the mechanism in action, e.g., the sliding components of the wasp’s ovipositor or formation of the fish’s electric field. The prototype or device was then introduced and shown in action, focusing on the corresponding mechanism/feature employed in the design. The initial stage involved creating illustrations in Adobe Illustrator (version 28.0) for use in After Effects. Firstly, the illustration was blocked out by making basic shapes (), using the pen tool, adding further details using the gradient mesh tool and colour picker. The pencil tool, watercolour brush, and the ‘gaussian blur’ effect were used to create tone and additional texture.
Illustrator files were imported into Adobe AfterEffects (version 24.0.1). Separate layers were used for individually moving parts to facilitate animation in AfterEffects. This was done by re-adjusting the anchor points for each part and then setting up keyframes, particularly adjusting the position and rotation ones. ‘Easy ease’ was applied to make the movement smoother. For the wings and antennae of the wasp, the ‘wiggle’ expression [wiggle(amplitude, frequency)] was used on the rotation keyframes. The amplitude and frequency variables were adjusted to achieve just slight motion. Animation of the fish was more complex; videos of Elephant Nose fishes swimming were referenced to capture its movements well (Myaquariuminfo, Citation2020; Williamson, Citation2021). A pre-composition of the fish’s swimming motions was created first. This meant using 3D layers so that keyframes could also be adjusted in the y-axis (Purple Pie Studios, Citation2022). To add more dimension and realism, puppet pins were added to the fins and tail (Purple Pie Studios, Citation2022; TipTutZone, Citation2019). Once this was finalised, the main composition was adjusted so that the fish moved in the scene. Elements like the electric field and rocks were brought in to illustrate electrolocation. A mask was applied to provide an underwater effect (Grayson, Citation2010). Fade and zoom transitions were applied between scene changes in the long animations. Lastly, the animations were paired with relevant nature sounds from Pixabay (Citation2023), and background music from Bensound (Citation2023). An artificial intelligence voice was used to narrate the animations (Veed.io, Citation2023) along with descriptive text explaining the processes described to ensure that the animation was informative and accessible even without audio. Sound was adjusted using the waveform keyframes. Finally, the files were exported as MP3s, rendered, and reviewed.
The same process as described for the long animations was used to create the short ones. They consisted of short, motion graphics of close-ups of the natural mechanisms alongside graphics of the medical devices for comparison. In addition, these pages included written explanatory content, and illustrations created in Adobe Photoshop (version 25.0) of each animal.
Wix Website Builder (Citation2023) was used to design the website. The media and content created were imported and arranged on webpages using the ruler and border tools. Background colours were selected, and gradients were used to create a natural backdrop. Descriptions were written based on the literature review but were presented as simply as possible to cater for a wide audience. Further information and links were provided. present images of some of the graphics and illustrations, and present images from the long animations and presents an image showing a section of the website.
Ethical approval
All research methods were carried out in accordance with the principles stated in the Declaration of Helsinki. Ethical approval was applied for and granted by the University of Dundee Research Ethics Committee to carry out a feedback survey (Approval number: UOD_SSEREC_CAHID_STAFF_2022_001).
Survey feedback
Jisc Online Surveys was selected as the survey tool as it provides a secure and confidential service, protecting participant information. The survey was aimed at the public to gain feedback on the final resource. The questionnaire collected both quantitative and qualitative data via Likert scale questions and open comment questions (optional), respectively. The questions were to evaluate the usability of the website, content, and artwork (animations and illustrations), in addition to determining a baseline of any prior knowledge or interest in the topic among participants, and their opinions on the topic after viewing the resource. Participants were recruited by sharing a link to the survey on Instagram, a social media platform. Participants had to first read the participant information sheet and provide informed consent ahead of viewing the resource and answering the questionnaire. The survey was available to the public for four days.
Results
The results of the feedback survey are outlined below. Twenty-three participants were recruited. Quantitative and qualitative results are presented in full in the additional supporting materials.
Quantitative results
On average, participants spent 6–10 min viewing the website. Over half of the participants (65.2%) had no prior knowledge or interest in biomimicry, and 52.2% reported they had prior knowledge of medical design. Participants were asked four questions about the website interface and design. In terms of how participants found the aesthetics of the website, 91.3% of participants either strongly agreed (69.6%) or agreed (21.7%) that it was aesthetically pleasing, while 8.6% strongly disagreed or were neutral. while 8.6% strongly disagreed or were neutral. Participants strongly agreed (73.9%) and agreed (21.7%) that it was easy to navigate and user-friendly. Participants (95.7%) also found that the website presented information clearly and was a helpful tool and a useful learning resource for those interested in biomimicry. Participants were asked three questions about the animations in terms of quality, clarity, and knowledge transfer, and strongly agreed or agreed (95.7%) that the animations were professional, of high quality, communicated the medical technology effectively, and were beneficial for learning about biomimicry.
Qualitative results
The survey included open-comment questions asking participants for comments and feedback on the website and animations. Participants were also asked, having viewed the resource, if they believed that biomimicry could offer useful solutions in medical design. At the end of the survey, they were again prompted to provide any other general feedback they might have. The open-comment questions were optional for participants to complete (with no word limit). The written transcripts can be viewed in the supplementary materials. This qualitative data was assessed by thematic analysis using the method described by Braun and Clarke (Citation2006). This method involves six steps including coding key words and recognising themes in the written comments. Three key themes were identified: visuals, communication, and learning.
Visuals related to comments about the illustrations and animations. Communication described comments about layout, design and how information was conveyed. Learning encompassed comments about the transfer of knowledge, how engaged participants were with the content, and if they felt the resource was educational. Regarding animations and illustrations, participants commented: I loved it, ‘AMAZING illustrations’. Throughout the written feedback, the word ‘clear’ was used most often, a total of nine times, the word ‘interesting’ was used eight times, and the word ‘learn’ seven times. Feedback the website received included being ‘well organised’ and ‘user friendly’. Participants reported learning from the resource and having gained an interest in the topic: ‘I am also now interested to learn more about this topic’. Some suggestions were made for improving the website design, including two comments recommending adjustment of the background colours as they were ‘a bit dark at times’.
Feedback on the animations included two comments that relayed how the nature sounds made the animations even more immersive: ‘Another thing that I liked were the ocean sounds during the animation they really made it an immersive experience’. Participants suggested that the animations helped them to understand the concepts more easily: ‘the graphics are excellent and add another dimension’; ‘it really simplifies the connection between the concepts and how they can be applied in medicine - the animations are very valuable in conveying this’; ‘the sounds and colours were so immersive’. One suggestion was made to: ‘Give a snapshot or write a line from the evidence base under the actual medical device animation to demonstrate its use in a more realistic manner’.
When asked if they believed if biomimicry could provide useful solutions in medical design, 96% of participants responded and all agreed that it could be a useful solution: ‘a fascinating subject and biomimicry is a rich source for medical design’; ‘definitely, I now think there must be lots of things we can learn from animals and nature that could be incredibly useful or could change current practices in medical design’.
Discussion
Objectives
The primary objective was to create a learning resource that provided examples of existing technology and up-and-coming research on applications of biomimicry in medicine, by designing a highly illustrated and engaging resource on an interactive website. Animations were created as they provide a format that is accessible to the public and can convey complex design concepts in a way that is both visually appealing and easier to understand. The second objective was to assess how participants interacted with the resource, to collect data on user feedback and suggestions, and to find out if participants believed that it was worthwhile to consider biomimicry when developing design solutions in medicine.
Interpretation of results
Based on the feedback received, the primary objective of this project was met. The most common words in the open-comment sections were ‘clear’ and ‘interesting’. The written feedback indicated that most participants enjoyed the resource and found the topic to be interesting. Three answers mentioned learning from the resource: ‘I am also now interested to learn more’; ‘I learned a lot from the animations’; and ‘I felt able to learn from them’. The word ‘learn’ was mentioned seven times in the open comment sections. The identified themes suggested that participants found the resource content to be visually appealing, to communicate the information well, and to be of educational value.
Constructive feedback primarily related to the website design and layout. There were two suggestions to change the colours on the webpage to make them lighter/pastel: ‘the website design could be improved a little more and some pastel colours could be used to make it easier on the eye’; ‘the background colours are a bit dark at points and maybe a bit distracting’. Conversely, some of the feedback received indicated that the colours made the webpages more ‘immersive’ and ‘match[ed] the different organisms well’. The background colours could be adjusted to find a middle ground between the two lines of feedback and more time spent on developing the website design. There were other useful suggestions, such as the recommendation to add a search tool and to present the shorter animations side-by-side so that they could be more easily compared.
Participants knowledge and opinions of biomimicry having viewed the resource were assessed. Participants were asked if they knew what biomimicry was before viewing the resource and 65.2% of them reported having no prior knowledge or interest in it. Interestingly, all participants (22/23) who responded to the question: ‘Having viewed the resource do you think biomimicry could provide useful solutions in medical design?’ said ‘yes’. This suggests that this resource could be beneficial and inspiring to the public.
Limitations
Twenty-three participants were recruited (likely due to the limited survey timeframe of four days). A larger sample size would have offered a greater representation of the public and provided further insight. The small sample size prevents meaningful conclusions from being made. However, diverse feedback and suggestions were received. On review of the data collected, more specific questions could have been included in the survey to better understand what worked and what did not, e.g., animation preferences. Questions about the website background colours and design elements would have been useful to gain an insight into how each participant found these. For the optional open-comment questions, 61% of participants responded, a non-optional field may have prompted more feedback. Another limitation of the survey is the potential for self-selection bias as it was shared on social media. Participants who chose to take part may have had an existing interest in biomimicry/art/medical design that influenced engagement and responses.
Future directions
The resource could be developed further based on the feedback collected. Participants also suggested expanding the site and adding more examples. Therefore, it would be recommendable to create additional content and develop the design and interactivity of the website, as well as making it more mobile friendly. This would also help to maximise the audience reach and increase the accessibility of the website. A larger survey could also be created with specific questions about participants’ views of biomimicry, even testing their pre- and post-knowledge to assess the educational value of the resource (University of Washington, Citation2008).
Supplemental Material
Download MS Word (1.1 MB)Disclosure statement
No potential conflict of interest was reported by the author(s).
References
- Allerton, E. (2023). The world’s first germ resistant material, inspired by… sharks? https://goodness-exchange.com/worlds-first-germ-resistant-pattern-sharks/
- Alves, D., Grainha, T., Pereira, M. O., & Lopes, S. P. (2023). Antimicrobial materials for endotracheal tubes: A review on the last two decades of technological progress. Acta Biomaterialia, 158, 32–55. https://doi.org/10.1016/j.actbio.2023.01.001
- Andrews, E., Weaver, A., Hanley, D., Shamatha, J., & Melton, G. (2005). Scientists and public outreach: Participation, motivations, and impediments. Journal of Geoscience Education, 53(3), 281–293. https://doi.org/10.5408/1089-9995-53.3.281
- Bensound. (2023). Royalty free music for videos. https://www.bensound.com/
- Braun, V., & Clarke, V. (2006). Using thematic analysis in psychology. Qualitative Research in Psychology, 3(2), 77–101. https://doi.org/10.1191/1478088706qp063oa
- Britannica. (2023). Electrical impedance, Encyclopædia Britannica. https://www.britannica.com/science/ electrical-impedance
- Cambridge Dictionary. (2023) Percutaneous. https://dictionary.cambridge.org/us/dictionary/english/percutaneous
- Centers for Disease Control and Prevention. (2019). Antibiotic resistant germs in hospitals. https://www.cdc.gov/hai/patientsafety/ar-hospitals.html
- Cerkvenik, U., van de Straat, B., Gussekloo, S. W. S., & van Leeuwen, J. L. (2017). Mechanisms of ovipositor insertion and steering of a parasitic wasp. Proceedings of the National Academy of Sciences, 114(37), E7822–E7831. https://doi.org/10.1073/pnas.1706162114
- Chen, Y., Liao, S., Mensah, A., Wang, Q., & Wei, Q. (2023). Hydrogel transformed from Sandcastle-worminspired powder for adhering wet adipose surfaces. Journal of Colloid and Interface Science, 646, 472–483. https://doi.org/10.1016/j.jcis.2023.05.009
- Chien, H.-W., Chen, X.-Y., Tsai, W.-P., & Lee, M. (2020). Inhibition of biofilm formation by rough shark skin-patterned surfaces. Colloids and Surfaces. B, Biointerfaces, 186, 110738. https://doi.org/10.1016/j.colsurfb.2019.110738
- Dixon, A. R., & Vondra, I. (2022). Biting innovations of mosquito-based biomaterials and medical devices. Materials, 15(13), 4587. https://doi.org/10.3390/ma15134587
- Esa, N. E. F., Ansari, M. N. M., Razak, S. I. A., Ismail, N. I., Jusoh, N., Zawawi, N. A., Jamaludin, M. I., Sagadevan, S., & Nayan, N. H. M. (2022). A review on recent progress of Stingless Bee Honey and its hydrogel-based compound for Wound Care Management. Molecules, 27(10), 3080. https://doi.org/10.3390/molecules27103080
- Finkler, W., & Leon, B. (2019). The power of storytelling and video: A visual rhetoric for science communication. Journal of Science Communication, 18(05), A02. https://doi.org/10.22323/2.18050202
- Frasson, L., Ko, S. Y., Turner, A., Parittotokkaporn, T., Vincent, J. F., & Rodriguez y Baena, F. (2010). Sting: A soft-tissue intervention and neurosurgical guide to access deep brain lesions through curved trajectories. Proceedings of the Institution of Mechanical Engineers. Part H, Journal of Engineering in Medicine, 224(6), 775–788. https://doi.org/10.1243/09544119jeim663
- Fuerst, B., Sutton, E. E., Ghotbi, R., Cowan, N. J., & Navab, N. (2016). Bioelectric navigation: A new paradigm for Intravascular device guidance. In Medical image computing and computer-assisted intervention (pp. 474–481). Springer. https://doi.org/10.1007/978-3-319-46720-7_55
- Ghosh, A., Li, L., Xu, L., Dash, R. P., Gupta, N., Lam, J., Jin, Q., Akshintala, V., Pahapale, G., Liu, W., Sarkar, A., Rais, R., Gracias, D. H., & Selaru, F. M. (2020). Gastrointestinal-resident, shape-changing microdevices extend drug release in vivo. Science Advances, 6(44), abb4133. https://doi.org/10.1126/sciadv.abb4133
- Gidde, S. T., & Hutapea, P. (2021). Design and experimental evaluation of mosquito-inspired needle structure in soft materials [Paper presentation]. Biomedical and Biotechnology, 5. https://doi.org/10.1115/IMECE2020-24320
- Grayson, L. (2010). After effects tutorial - Underwater scene with light rays and bubbles, YouTube. Short-form video. https://www.youtube. com/watch?v=pjdmXYRzAWg
- Gurera, D., Bhushan, B., & Kumar, N. (2018). Lessons from mosquitoes’ painless piercing. Journal of the Mechanical Behavior of Biomedical Materials, 84, 178–187. https://doi.org/10.1016/j.jmbbm.2018.05.025
- Høiby, N., Bjarnsholt, T., Moser, C., Bassi, G. L., Coenye, T., Donelli, G., Hall-Stoodley, L., Holá, V., Imbert, C., Kirketerp-Møller, K., Lebeaux, D., Oliver, A., Ullmann, A. J., & Williams, C. (2015). ESCMID guideline for the diagnosis and treatment of biofilm infections 2014. Clinical Microbiology and Infection , 21 (1), S1–S25. https://doi.org/10.1016/j.cmi.2014.10.024
- Hussein, A. (2023). Biofouling. In Essentials of flow assurance solids in oil and gas operations (pp. 449–483). Elsevier. https://doi.org/10.1016/b978-0-323-99118-6.00008-3
- Kier, W. M., & Smith, A. M. (2002). The structure and adhesive mechanism of octopus suckers. Integrative and Comparative Biology, 42(6), 1146–1153. https://doi.org/10.1093/icb/42.6.1146
- Kim, B. S., Kim, M. K., Cho, Y., Hamed, E. E., Gillette, M. U., Cha, H., Miljkovic, N., Aakalu, V. K., Kang, K., Son, K.-N., Schachtschneider, K. M., Schook, L. B., Hu, C., Popescu, G., Park, Y., Ballance, W. C., Yu, S., Im, S. G., Lee, J., Lee, C. H., & Kong, H. (2020). Electrothermal soft manipulator enabling safe transport and handling of thin cell/tissue sheets and bioelectronic devices. Science Advances, 6(42), abc5630. https://doi.org/10.1126/sciadv.abc5630
- Knapp, P., Benhebil, N., Evans, E., & Moe-Byrne, T. (2022). The effectiveness of video animations in the education of healthcare practitioners and student practitioners: A systematic review of trials. Perspectives on Medical Education, 11(6), 309–315. https://doi.org/10.1007/s40037-022-00736-6
- Leiner, M., Handal, G., & Williams, D. (2004). Patient communication: A multidisciplinary approach using animated cartoons. Health Education Research, 19(5), 591–595. https://doi.org/10.1093/her/cyg079
- Li, A. D. R., Putra, K. B., Chen, L., Montgomery, J. S., & Shih, A. (2020). Mosquito proboscis-inspired needle insertion to reduce tissue deformation and organ displacement. Scientific Reports, 10(1), 12248. https://doi.org/10.1038/s41598-020-68596-w.
- Ma, Y., Xiao, X., Ren, H., & Meng, M. Q.-H. (2022). A review of bio-inspired needle for percutaneous interventions. Biomimetic Intelligence and Robotics, 2(4), 100064. https://doi.org/10.1016/j.birob.2022.100064
- Mann, E. E., Manna, D., Mettetal, M. R., May, R. M., Dannemiller, E. M., Chung, K. K., Brennan, A. B., & Reddy, S. T. (2014). Surface micropattern limits bacterial contamination. Antimicrobial Resistance and Infection Control, 3(1), 28. https://doi.org/10.1186/2047-2994-3-28
- Mann, E. E., Magin, C. M., Mettetal, M. R., May, R. M., Henry, M. M., DeLoid, H., Prater, J., Sullivan, L., Thomas, J. G., Twite, M. D., Parker, A. E., Brennan, A. B., & Reddy, S. T. (2016). Micropatterned endotracheal tubes reduce secretion-related lumen occlusion. Annals of Biomedical Engineering, 44(12), 3645–3654. https://doi.org/10.1007/s10439-016-1698-z
- MediPal. (2022). Keeping high-touch areas safe and clean. https://www.palinternational.com/en/healthcare/knowledge-hub/learning-resources/keeping-high-touch-areas-safe-and-clean-hospital/
- MedStar Health. (2023). Radiofrequency ablation. https://www.medstarhealth.org/services/radiofrequency-ablation-atrialfibrilation
- Meyers, P. Z. (2006). How octopus suckers work. https://scienceblogs.com/pharyngula/2006/05/17/howoctopus-suckers-work
- Moe-Byrne, T., Evans, E., Benhebil, N., & Knapp, P. (2022). The effectiveness of video animations as information tools for patients and the general public: A systematic review. Frontiers in Digital Health, 4, 1010779. https://doi.org/10.3389/fdgth.2022.1010779
- Mosquito Magnet. (2020). How mosquitoes bite. https://www. mosquitomagnet.com/articles/how-mosquitoes-bite (Accessed: 01 June 2023).
- Myaquariuminfo. (2020). Elephant nose fish care and information. https://www.youtube.com/watch?v=buvQ4rQ8Y8Q
- Phee, L., et al. (2003). Analysis of robotic locomotion devices for the gastrointestinal tract. In Springer tracts in advanced robotics (pp. 467–483). https://doi.org/10.1007/3-540-36460-9_31
- Pixabay. (2023). Pixabay. https://pixabay.com/ sound-effects/.
- Purple Pie Studios. (2022). Fish animation in After Effects tutorial. https://www.youtube.com/watch?v=DbBb7WOwfKM
- Reddy, S. T. (2014). Surface micropattern resists bacterial contamination transferred by healthcare practitioners. Journal of Microbiology & Experimentation, 1(5), 32. https://doi.org/10.15406/jmen.2014.01.00032
- Reddy, S. T., Chung, K. K., McDaniel, C. J., Darouiche, R. O., Landman, J., & Brennan, A. B. (2011). Micropatterned surfaces for reducing the risk of catheter-associated urinary tract infection: An in vitro study on the effect of Sharklet micropatterned surfaces to inhibit bacterial colonization and migration of uropathogenic Escherichia coli. Journal of Endourology, 25(9), 1547–1552. https://doi.org/10.1089/end.2010.0611
- Sakes, A., van de Steeg, I. A., de Kater, E. P., Posthoorn, P., Scali, M., & Breedveld, P. (2020). Development of a novel wasp-inspired friction-based tissue transportation device. Frontiers in Bioengineering and Biotechnology, 8, 575007. https://doi.org/10.3389/fbioe.2020.575007
- Scali, M. (2020). Self-propelling needles: From biological inspiration to percutaneous interventions [Published PhD thesis]. Delft University of Technology. https://repository.tudelft.nl/islandora/object/uuid:523e3e5f-08f0-4acb-ab45- abaa7ace3967?collection=research
- Scali, M., Breedveld, P., & Dodou, D. (2019). Experimental evaluation of a self-propelling bio-inspired needle in single- and multi-layered phantoms. Scientific Reports, 9(1), 19988. https://doi.org/10.1038/s41598-019-56403-0
- Sprang, T., Breedveld, P., & Dodou, D. (2016). WASP-inspired needle insertion with low net push force. In Biomimetic and biohybrid systems (pp. 307–318). Springer. https://doi.org/10.1007/978-3-319-42417-0_28
- Stilgoe, J., Lock, S. J., & Wilsdon, J. (2014). Why should we promote public engagement with science? Public Understanding of Science, 23(1), 4–15. https://doi.org/10.1177/0963662513518154
- Sutton, E. E. (2017). Bioelectric sensing and navigation: Multimodal control in electric fish and endovascular device guidance [Published dissertation]. John Hopkins University. http://jhir.library.jhu.edu/handle/1774.2/60228
- Sutton, E. E., Fuerst, B., Ghotbi, R., Cowan, N. J., & Navab, N. (2020). Biologically inspired catheter for endovascular sensing and navigation. Scientific Reports, 10(1), 5643. https://doi.org/10.1038/s41598-020-62360-w
- Sykes, M., Cerda, L., Cerda, J., & Finch, T. (2022). Disseminating implementation science: Describing the impact of animations shared via social media. PLOS One, 17(7), e0270605. https://doi.org/10.1371/journal.pone.0270605
- Tatalovic, M. (2009). Science comics as tools for Science Education and Communication: A brief, exploratory study. Journal of Science Communication, 08(04), A02. https://doi.org/10.22323/2.08040202
- TipTutZone. (2019). The puppet pin tool. https://www.youtube.com/watch?v=KJWBosNSJv8
- Trumbo, J. (2000). Essay: Seeing science. Science Communication, 21(4), 379–391. https://doi.org/10.1177/1075547000021004004
- University of Washington. (2008). Guidelines for pre- and post-testing, I-Tech Technical Implementation Guide. Available at: https://studylib.net/doc/8246780/guidelines-for-pre--and-post-testing---i-tech#:∼:text=Guidelines%20for%20Pre-%20and%20Post-Testing%20I-TECH%E2%80%99s%20Technical%20Implementation,knowledge%20gained%20from%20participating%20in%20a%20training%20course (Accessed: 30 June 2024).
- van Meer, N. M. M. E., Cerkvenik, U., Schlepütz, C. M., van Leeuwen, J. L., & Gussekloo, S. W. S. (2020). The ovipositor actuation mechanism of a parasitic wasp and its functional implications. Journal of Anatomy, 237(4), 689–703. https://doi.org/10.1111/joa.13216
- Veed.Io, (2023). Video editor. https://www.veed.io/workspaces/a37ecc24-4816- 42f2-a12e-17d980033257/home
- Von der Emde, G. (1999). Active electrolocation of objects in weakly electric fish. Journal of Experimental Biology, 202(10), 1205–1215. https://doi.org/10.1242/jeb.202.10.1205
- von der Emde, G., Mayekar, K., Bousack, H., & Pabst, M. (2009). Simulation of bionic electrolocation sensor based on weakly electric fish [Paper presentation]. 2009 World Congress on Nature & Biologically Inspired Computing (NaBIC) (pp. 830–834). https://doi.org/10.1109/NABIC.2009.5393562
- Williamson, A. (2021). The elephant nose knife fish a shockingly cool oddball fish. https://www.youtube.com/watch?v=wZ0wRHssZD0#:∼:text=now%20this%20fish%20has%20a,and%20basically%20a%20negative
- Wix Website Builder. (2023). Wix. https://manage.wix.com/account/sites?referralAdditionalInfo=Route
- Yaghoobi, R., Kazerouni, A., & Kazerouni, O. (2013). Evidence for clinical use of honey in wound healing as an anti-bacterial, anti-inflammatory anti-oxidant and anti-viral agent: A review. Jundishapur Journal of Natural Pharmaceutical Products, 8(3), 100–104. https://doi.org/10.17795/jjnpp-9487
- Yang, M., & Zahn, J. D. (2004). Microneedle insertion force reduction using vibratory actuation. Biomedical Microdevices, 6(3), 177–182. https://doi.org/10.1023/b:bmmd.0000042046.07678.2e
- Zakon, H. H., & Smith, G. T. (2002). Weakly electric fish. In Hormones, brain and behaviour (pp. 349–374). Academic Press. https://doi.org/10.1016/b978-012532104-4/50026-3
- Zimmerman, D. E., Akerelrea, C., Smith, J. K., & O’Keefe, G. J. (2006). Communicating forest management science and practices through visualized and animated media approaches to Community Presentations. Science Communication, 27(4), 514–539. https://doi.org/10.1177/1075547006288004