Abstract
Background Various cells and scaffolds have been used experimentally as interposition materials after physeal bar resection to treat partial physeal growth arrest. However, results of these cell therapies are unpredictable.
Methods We investigated the chondrogenic differentiation of rabbit perichondrial cells in vitro. We also investigated radiographic and histological changes after implantation of the perichondrium-derived chondrocytes, embedded in fibrin beads, into defects created in the medial aspect of the proximal tibial physis of 26 8-week-old New Zealand White rabbits. Physeal defects of the contralateral tibiae were left intact without any treatment, or were treated with fibrin beads not containing cells.
Results The perichondrial cells cultured in the alginate- fibrin beads were positive in immunohistochemical staining for S-100 protein and the extracellular area was stained with Safranin-O. RNase protection assay showed that the expression of type II collagen and aggrecan continued In the cell and bead-treated tibiae, varus deformity was reduced significantly and tibial length was maintained longer than that of the contralateral tibia. Histologically, cartilage clusters of varying size with intervening micro-bony trabeculae were found in the cell and bead-treated tibiae. The lateral, remaining physis in the untreated tibiae showed altered histology.
Interpretation Implantation of fibrin beads containing perichondrium-derived chondrocytes into the physeal defect helps to prevent angular deformation and shortening of long bone, but not completely. Physeal bony bar formation adversely affects the growth of the remaining physis from early stages onward.
Physeal bar resection with fat graft is a standard method for the treatment of partial arrest of physeal growth (Langenskiöld Citation1967). A number of clinical and experimental studies using this procedure, and also modifications of the interposition materials, have been reported (Bright Citation1974, Peterson Citation1980, Lennox et al. Citation1983, Peterson Citation1984, Broughton et al. Citation1989, Lee et al. Citation1998), with varying degrees of success. Recently, biological interposition materials—including cultured chondrocytes—have been tried for regeneration of the injured physeal cartilage. Many studies, however, have used cartilage of the articular surface or physis as the source of chondrocytes, resulting in morbidity of the donor site. Sometimes allogenic chondrocytes were implanted (Lee et al. Citation1998) and their carriers were not biodegradable. Moreover, many previous methods of creating physeal defects may not reflect the real clinical situation in physeal bar resection.
In this study, we implanted autogenous chondrocytes derived from chondrogenic differentiation of the perichondrial cells, with a biodegradable fibrin carrier, into a physeal defect. We evaluated the chondrogenic differentiation of the rabbit perichondrial cells in vitro, and the radiographic and histological changes after implantation of fibrin beads containing perichondrium-derived chondrocytes into the physeal defects of rabbit tibiae.
Material and methods
Harvest of perichondrium and alginate-fibrin bead culture
The experimental protocol was approved by the Institutional Animal Care and Use Committee (IACUC) of our hospital. 2 or 3 ribs were harvested from the right lower chest of 8-week-old New Zealand White rabbits (n = 39). Care was taken not to open the pleura. Perichondrium was peeled off and digested enzymatically (0.2% protease for 40 min and then 0.2% collagenase for 2 h). After centrifugation, the cell pellet was resuspended in Dulbecco's modified Eagle's medium (DMEM) supplemented with 10% fetal bovine serum, 50 μg/mL ascorbic acid, 100 IU/mL penicillin, 100 μg/mL streptomycin, and the cells were cultured as a monolayer until 90% confluence was reached. We used the method of Perka et al. Citation(2000) to make alginate-fibrin beads. Briefly, perichondrial cells from monolayer culture were suspended in cell suspension solution (140 mM NaCl, 5 mM KCl, 10 mM HEPES, 10 mM glucose, 0.02% EDTA), 4.8% alginate (low-viscosity alginate; Sigma, Munich, Germany) in isotonic NaCl, and 9.0% fibrinogen solution (Greenplast, Greencross PBM, Yongin, Korea), which were mixed in a 1:2:5 ratio. The cell density of the mixture was 3 × 106cells/mL. Using a syringe with a 23-guage needle, the cell suspension was dropped into a mixture of 102 mM calcium chloride (10 mL) and thrombin (Greenplast, 1 mL), to obtain the alginate-fibrin beads containing perichondrial cells. To prepare alginate beads, cells were suspended in 1.2% alginate solution, and the solution was dropped into 102 mM calcium chloride solution. The alginate beads and alginate-fibrin beads were maintained in the same medium used for monoclayer culture but supplemented with TGF-ß1 (5.0 ng/mL human TGF-ß1; Sigma, Munich, Germany).
In vitro study for the chondrogenic differentiation of perichondrial cells
The perichondrial cells were cultured as monolayers, in alginate-fibrin beads, and in alginate beads. At 3 weeks of culture, RNA was extracted from the cells for the RNase protection assay (RPA). At that time, the alginate component of the alginate-fibrin beads was removed by treating the beads with a solution of 55 mM sodium citrate, 150 mM NaCl, and 30 mM EDTA (Perka et al. 2000 a). After culture for 3 additional weeks, we extracted RNA from the cells in monolayer, in the fibrin beads, and in the alginate beads. RPA for collagen type I, II, X, aggrecan, and ß-actin was performed on the RNA samples using a RiboQuant ribonuclease protection kit (PharMin-gen, San Diego, CA). The base sequence for the probe template was sense: 5’-ACATGGATGAG-GAAACTGGC-3’, anti-sense:5’-GCAATATCAA-GGAAGGGCAA-3’for type I collagen (COL1A2), sense: 5’-CGAGATCCCCTTCGGAGAGT-3’, anti-sense:5’-TCGGCCACGAGGTCCAGGGG-3’for type II collagen (COL2A1), sense: 5’-CAGGACTCCCAGGAAAACA-3’, anti-sense: 5’-CCAGGAGCACCATATCCTGT-3’, for type X collagen (COL10A1), sense: 5’-GAGGTCGTG-GTGAAAGGTGT-3’, anti-sense:5’-AAGGTGA-ACTTCTCTGGCGA-3’for aggrecan, sense: 5’-GGACCTGACCGACTACCTCA-3’, anti-sense: 5’-GGCAGCTCGTAGCTCTTCTC-3’ for ß-actin. Linearized plasmids containing each of the selected genes were transcribed with [32P]-uridine triphosphate (UTP; PharMingen, San Diego, CA) and T7 RNA polymerase and then they were digested with DNase, extracted with phenol, and ethanol-precipitated. Probes were made from multiprobe template sets and hybridized overnight at 56°C with the various RNA samples. This was followed by RNase digestion, proteinase K digestion, phenol extraction, and ethanol precipitation. The products were then fractionated on a denaturing 6% polyacrylamide gel.
Histological sections of the alginate-fibrin beads containing perichondrial cells that had been cultured for 3 weeks were stained with Safranin-O for evaluation of proteoglycan synthesis, and immunohistochemically for S-100 protein which is expressed in chondrocytes (Wolff et al. Citation1992). Briefly, 4 μm-thick sections were treated with polyclonal rabbit antiserum to S-100 (1:1000; DAKO, Carpinteria, CA) for 90 min at room temperature. Consecutive treatments with biotinylated goat anti-rabbit IgG and horseradish peroxidase-conjugated streptavidin, and reaction with 3-3’diaminobenzidine were performed using the DN universal detection kit (Dinona, Seoul, Korea).
Implantation of cell-fibrin bead complex into physeal defects
One day before the operation, the alginate component was removed from the alginate-fibrin beads. A longitudinal skin incision was made on the anteromedial aspect of the proximal tibia bilaterally. We removed 40% of the cross-sectional area of the proximal tibial physis along with its adjoining epiphysis and metaphysis using a box osteotome bilaterally. The cross section of the proximal tibial physis is elliptical and its area was calculated by measuring the anteroposterior and mediolateral diameters on the preoperative plain radiographs. The custom-made osteotome had box-shaped blades, 6.5 mm wide and 3 mm long. The depth of punching of the osteotome was predetermined to make the 40% rectangular physeal defect (). The depth and width of the physeal defect was confirmed after removing pieces of bone. After irrigation with saline solution, Gelfoam sponge was inserted temporarily for hemostasis. We then implanted autogenous cell-fibrin beads into the defect of the left tibia. The right tibial defects, which served as controls, were left untreated (n = 30) or had fibrin beads without cells inserted (n = 9). The perichondrium, periosteum and skin were closed layer by layer. Postoperatively, the rabbits were allowed to move freely. They were assigned to 5 groups according to the time of killing: a 2-week group (n = 6), 4-week (n = 9), 8-week (n = 9), 12-week (n = 9), and 16-week group (n = 6).
Radiographic, histological and histomorphometric evaluation
Of the 39 rabbits, 13 could not be evaluated because of intraoperative death (n = 6), tibial plateau fracture (n = 6), and postoperative infection (n = 1). Among the 13 excluded cases, 10 rabbits had untreated defects and 3 rabbits had defects in which fibrin beads without cells had been inserted. Posteroanterior radiographs of the hindlimbs were taken at 2-week intervals in the prone position until 16 weeks, to evaluate temporal changes in the length of the medial and lateral columns, and varus angulation of the tibia. We defined medial column length as the length between the proximal medial tibial plateau and the distal medial tibial physis. Lateral column length was defined as the length between the proximal lateral tibial plateau and the distal lateral tibial physis. Angle of the tibia vara was defined as an acute angle between the line perpendicular to the proximal tibial plateau and the line perpendicular to the distal tibial physis. Rabbits were killed at 2 (n = 4), 4 (n = 5), 8 (n = 4), 12 (n = 8), and 16 (n = 5) weeks after operation. The proximal tibia was fixed in 10% neutral formalin, decalcified, and then embedded in paraffin. Coronal sections of the paraffin block were examined histologically after Safranin-O staining. The thickness of the lateral remaining part of the physis was measured using a light microscope connected to a CCD video camera (TK-C1380, JVC, Tokyo, Japan) with image-processing software Image-Pro Plus (version 4.5; Media Cybernetics, Silver Spring, MD). We defined the thickness of the physis as previously described (Lee et al. Citation1993): the length from the edge of the reserve zone to the last hypertrophic chondrocyte in the transverse cartilage septum before vascular ingrowth. The measurements were carried out at medial, middle, and lateral thirds of the remaining physis, 15 times at each region, and averaged.
Statistics
We used repeated ANOVA test for comparison of the changes in length and varus deformity of tibia, and t-test for the comparison of the height of the remaining physis (SPSS version 10.0; SPSS Inc., Chicago, IL). P-values < 0.05 were considered statistically significant.
Results
RNase protection assay showed that type II collagen and aggrecan gene expression, indicative of chondrocytic phenotype, continued in the perichondrium-derived cells cultured in the alginate-fibrin beads at 3 weeks and in fibrin beads at 6 weeks, although the expression was not as intense as in the alginate beads. In contrast, perichondrium-derived cells in monolayer culture had lost their type II collagen and aggrecan expression by 6 weeks (). Perichondrial cells cultured in the alginate-fibrin beads for 3 weeks were positive for immunohistochemical staining of S-100 protein and the extracellular area was stained by Safranin-O ().
Figure 2. Results of RNase protection assay.(a) 3 weeks after monolayer culture and three-dimensional culture using alginate beads or alginate-fibrin beads.(b) 3 additional weeks after removal of the alginate component from alginate-fibrin beads.Expression of type II collagen and aggrecan was disappearing in monolayer culture at 6 weeks, while it continued in three-dimensional cultures.Although the expression of type II collagen and aggrecan continued in fibrin beads at 6 weeks, it was much weaker than with alginate beads. COL: collagen.
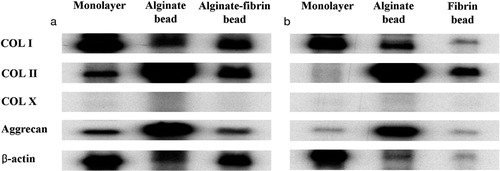
Figure 3. Safranin-O staining and immunohistochemistry for detection of S-100 protein 3 weeks after alginate-fibrin bead culture of perichondrial cells.(a) The alginate-fibrin scaffold is stained positively by Safranin-O (× 100).(b) The perinuclear cytoplasm is immunoreactive for S-100 protein (× 400).
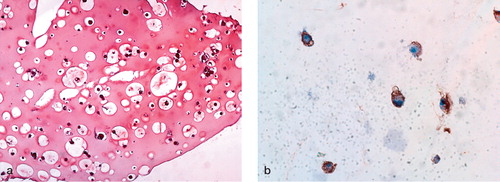
Radiographically, varus deformation of the cell and bead-treated left tibia was less than that of the right tibia. Medial column length of the left tibia was longer than that of the right tibia. Lateral column length was similar between right and left ().
Figure 4. Tibial length and varus angulation.(a) 12 weeks after operation:marked varus deformity and shortening of right tibia.(b) The average medial column length of the left tibia is greater than that of the right tibia (p = 0.05, repeated ANOVA).(c) The average lateral column length is similar in the right and left tibiae (p = 0.4, repeated ANOVA).(d) The average angle of tibia vara of the left tibia is smaller than that of the right tibia (p = 0.004, repeated ANOVA).Error bar shows 95% confidence interval.
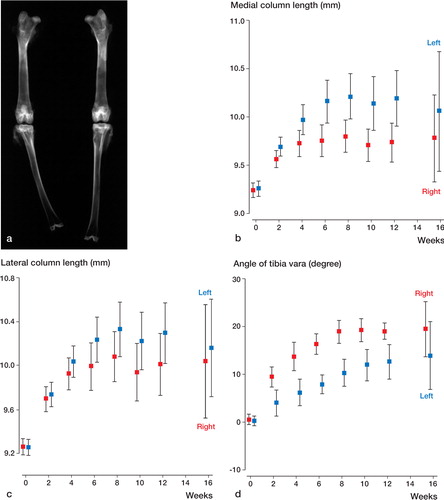
Histologically, we found cartilage clusters of varying size with intervening microbony trabeculae in the cell and bead-treated left tibiae in 17/26 cases, while bony physeal bar was observed in the right tibia in all cases, regardless of whether the defects remained untreated or were filled with fibrin beads alone, except three in the 2-week group. Some of the cartilage clusters were elongated along the longitudinal direction of physeal growth, and were composed of hypertrophied chondrocytes that were arranged haphazardly (). The lateral remaining physis of the cell and bead-treated left tibia was generally thicker than that of the right side at 2, 4, and 8 weeks after surgery (p = 0.004, p < 0.001, and p < 0.001, respectively, t-test), and the differences in the physeal height between the two sides increased with time. The normal columnar configurations of the physeal chondrocytes were altered and the cellularity was reduced in the remaining physis of the right tibia, while almost normal structure was maintained in the cell and bead-treated left side ().
Figure 5. Histological sections of proximal tibiae 12 weeks after operation.(a) Elongated cartilage clusters were observed in the defect of the left tibia (Safranin-O).(b) Hypertrophied chondrocytes in the cartilage clusters were arranged haphazardly.The extracellular space was stained positively with Safranin-O, but the staining was less intense than in the intact lateral physis (× 40).(c) Hypertrophied chondrocytes were immunoreactive for S-100 protein (× 200).(d) Bony physeal bar formed in the right tibial defect (Safranin-O).
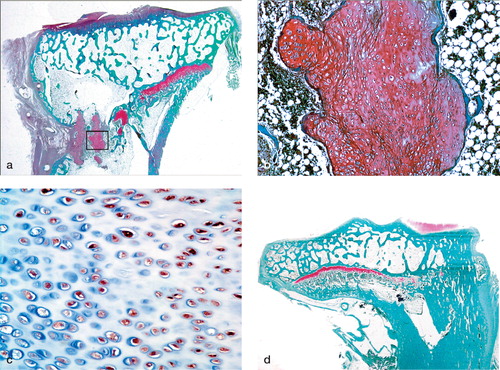
Figure 6. Photomicrographs of histological sections showing remaining lateral physis 8 weeks postoperatively.(a) Left tibial physis showing chondrocytes with near-normal columnar arrangement.(b) Right tibial physis showing reduced physeal height and irregularly arranged chondrocytes, suggesting premature physeal growth arrest (× 100, Safranin-O).
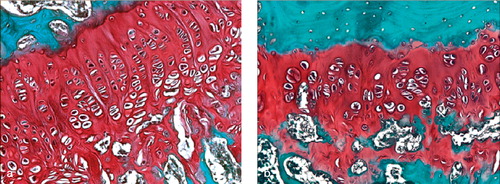
Discussion
Our experimental model differs from previous studies on the implantation of cultured cells into physeal defects, in terms of cells, scaffold, and methodology of creating the physeal defect. Considering the potential for differentiation and donor site morbidity, one of the best candidate cell types for the regeneration of injured physeal cartilage appears to be mesenchymal stem cells (MSC), but the chondrogenesis of MSC cannot be controlled completely yet, especially in vivo. We considered that perichondrium could be a possible alternative, because it contains a proportion of immature chondroprogenitor cells (Bulstra et al. Citation1990)—the chondrogenic potential of which has been proven both in vitro (Busltra et al. Citation1990, Dounchis et al. Citation1997) and in vivo (Chu et al. Citation1997, Dounchis et al. Citation2000). Control of chondrogenic differentiation is certainly much easier for chondroprogenitor cells than mesenchymal stem cells, and harvesting of perichondrium does not seem to be more harmful than harvesting of the cartilage of physis or articular surface.
The three-dimensional culture method using alginate-fibrin beads was introduced by Perka et al. (Citation2000a, Citationb, Citation2001). When chondrocytes are cultured in alginate-fibrin beads, chondrocytic phenotype survives well in the presence of alginate, while it is lost in monolayer culture. Removal of alginate component leaves biodegradable fibrin carrier with pores which may open additional spaces for extra-cellular matrix formation (Perka et al. Citation2000b). In our study, perichondrial cells showed the chondrocytic phenotype when they were cultured in alginate-fibrin beads and in fibrin beads after removal of the alginate component, and formed cartilage clusters after being implanted into the physeal defect with the biodegradable fibrin carrier. These results show that the advantages of the two-step culture method for chondrocytes are also applicable to perichondrial cells. Because fibrin has been used as biological glue, the fibrin scaffold can be especially beneficial in situations where hemostasis is important, as in physeal bar resection.
In many previous experiments on partial physeal growth arrest, a physeal defect was created with a scalpel or a curette (Martiana et al. Citation1996, Lee et al. Citation1998, Citation2002, Tobita et al. Citation2002). However, these methods may be accompanied by the risk of incomplete removal of physis, because many physes have undulating configuration and the resting zone of the physis is adjacent to the hard epiphyseal bone. We agree that these methods are appropriate for epiphysiodesis, but when physeal bar formation is prevented by any interposition materials, physeal remnants may proliferate and confuse the histological findings. Our method using a box osteotome not only creates bony defects of constant size, which is the major determining factor for the results of physeal bar resection, but also has the advantage of simulating the clinical situation in physeal bar resection—which involves removal of the adjacent epiphyseal and metaphyseal bone, allowing bone marrow to flow into the defect.
It is not an uncommon finding that some physes close earlier than their contralateral counterparts after physeal bar resection (Peterson Citation1984, Ogden Citation1987). In a study using MRI and CT scans, Hasler and Foster (Citation2002) showed that premature arrest of the remaining physis was found in 5 of 14 fair or poor results after physeal bar resection with fat graft. Some authors believe that latent damage to the uninjured part of the physis is responsible for this phenomenon, especially when the etiologies of physeal damage are infection (Langenskiöld Citation1981, Ogden Citation1987, Peters et al. Citation1992), ischemia (Ogden Citation1987), or irradiation (Peterson Citation1984). Insufficient latitudinal ingrowth of chondrocytes from the remaining physis was proposed as a possible mechanism when the resected bar is too large (Peterson Citation1984). However, the exact causes of this premature cessation of growth in the remaining physis are still uncertain. In our study, histological disorganization of the remaining physis was evident after extensive physeal bar formation (40% of physeal crosssectional area), and the differences in the remaining physeal heights between barformed physes and barprevented physes gradually increased over time. These results agree with a previous report (Phieffer et al. Citation2000) suggesting that the size of a physeal bar and its tethering time may affect the growth of the remaining physis. Synder et al. (Citation2001) described premature growth arrest of the normal central physis after peripheral epiphysiodesis in a rabbit model using MRI. In that study, the central part of the physis showed progressive narrowing as bone fusion occurred at the site of the epiphysiodesis, and MR changes showing altered architecture of chondrocytes were noted in advance of the development of mature bone bridge as early as between the first and the second postoperative week. In accordance with these results, we also observed narrowing and altered physeal configuration of the remaining physis as early as the second week postoperatively, when the radiological and histological evidence of physeal bar formation was not definite. Thus, we agree with the idea that there is another mechanism that affects physeal growth during the early stages of bony bar formation, in addition to the mechanical tethering effect of the mature bar. As early physeal growth arrest mostly occurred in the later barformed tibia even though both tibiae suffered equal amounts of surgical damage, altered blood supply to the remaining physis associated with surgical damage (which was proposed as a possible mechanism by Synder et al. (Citation2001)) does not appear to be a sufficient explanation in our model of physeal injury. Some biological changes occurring during the early stages of bony bar formation may be responsible for this phenomenon, but these require to be investigated further.
There were some drop-outs in our study (6 intraoperative deaths, 6 tibial plateau fractures, and 1 infection). Five intraoperative deaths were caused by pneumothorax and 1 by a problem arising from anesthesia. The tibial plateau fractures occurred in the earlier cases when the physeal defects had been made too proximally, which left the thin tibial plateau susceptible to fracture in weight bearing. We could reduce the fracture simply by lowering the osteotomy site.
There are several weak points in our experimental model. The expression of type II collagen and aggrecan continued, but was less pronounced in the cells cultured in alginate-fibrin beads or in fibrin beads than in the cells cultured in alginate beads. This finding suggests that the fibrin scaffold may be a harsh environment for the chondrocytic differentiation of perichondrial cells, although it is useful as a biodegradable carrier. The cartilage clusters were formed only in 65% of the cases with intervening microbony trabeculae. Culture of heterogenous cell populations including fibroblastlike cells and chondroprogenitors, resulting from the enzymatic digestion of the perichondrium, may have reduced the yield of cartilage clusters and may have been responsible for the persistent expression of type I collagen in bead culture. The cells in the cartilage clusters were arranged haphazardly—far from the normal physeal histological configurations. Induction of the normal stratified, columnar configuration from the chondrocytes in the cartilage cluster, both in vitro and in vivo, will be the ultimate target of investigations of physeal regeneration.
We thank Jin-A Kim for technical assistance with RPA and Harkmo Sung from Greencross Plasma Derivatives Co., Yongin, Korea, for supplying Greenplast.
This work was supported by grant no. R01-2002-000-00208-0 from the Basic Research Program of the Korea Sci-ence and Engineering Foundation.
- Bright R W. Operative correction of partial epiphyseal plate closure by osseous-bridge resection and silicone-rubber implant. An experimental study in dogs. J Bone Joint Surg (Am) 1974; 56: 655–64
- Broughton N S, Dickens D R, Cole W G, Menelaus M B. Epiphyseolysis for partial growth plate arrest. Results after four years or at maturity. J Bone Joint Surg (Br) 1989; 71: 13–6
- Bulstra S K, Homminga G N, Buurman W A. Terwindt-Rouwenhorst E, van der Linden A J. The potential of adult human perichondrium to form hyaline cartilage in vitro. J Orthop Res 1990; 8: 328–35
- Chu C R, Dounchis J S, Yoshioka M, Sah R L, Coutts R D, Amiel D. Osteochondral repair using perichondrial cells. A 1-year study in rabbits. Clin Orthop 1997, 340: 220–9
- Dounchis J S, Goomer R S, Harwood F L, Khatod M, Coutts R D, Amiel D. Chondrogenic phenotype of perichondrium- derived chondroprogenitor cells is influenced by transforming growth factor-beta 1. J Orthop Res 1997; 15: 803–7
- Dounchis J S, Coutts R D, Amiel D. Cartilage repair with autogenic perichondrium cell/polylactic acid grafts. A two-year study in rabbits. J Orthop Res 2000; 18: 512–5
- Hasler C C, Foster B K. Secondary tethers after physeal bar resection. A common source of failure?. Clin Orthop 2002, 405: 242-9
- Langenskiöld A. The possibilities of eliminating premature partial closure of an epiphyseal plate caused by trauma or disease. Acta Orthop Scand 1967; 38: 267–79
- Langenskiöld A. Surgical treatment of partial closure of the growth plate. J Pediatr Orthop 1981; 1: 3–11
- Lee C W, Martinek V, Usas A, Musgrave D, Pickvance E A, Robbins P. Muscle-based gene therapy and tissue engineering for treatment of growth plate injuries. J Pediatr Orthop 2002; 22: 565–72, (all names)
- Lee D Y, Chung C Y, Choi I H. Longitudinal growth of the rabbit tibia after callotasis. J Bone Joint Surg (Br) 1993; 75: 898–903
- Lee E H, Chen F, Chan J, Bose K. Treatment of growth arrest by transfer of cultured chondrocytes into physeal defects. J Pediatr Orthop 1998; 18: 155–60
- Lennox D W, Goldner R D, Sussman M D. Cartilage as an interposition material to prevent transphyseal bone bridge formation. An experimental model. J Pediatr Orthop 1983; 3: 207–10
- Martiana K, Low C K, Tan S K, Pang M W. Comparison of various interpositional materials in the prevention of transphyseal bone bridge formation. Clin Orthop 1996, 325: 218–24
- Ogden J A. The evaluation and treatment of partial physeal arrest. J Bone Joint Surg (Am) 1987; 69: 1297–1302
- Perka C, Schultz O, Spitzer R S, Lindenhayn K, Burmester G R, Sittinger M. Segmental bone repair by tissue-engineered periosteal cell transplants with bioresorbable fleece and fibrin scaffolds in rabbits. Biomaterials 2000a; 21: 1145–53
- Perka C, Spitzer R S, Lindenhayn K, Sittinger M, Schultz O. Matrix-mixed culture: new methodology for chondrocyte culture and preparation of cartilage transplants. J Biomed Mater Res 2000b; 49: 305–11
- Perka C, Arnold U, Spitzer R S, Lindenhayn K. The use of fibrin beads for tissue engineering and subsequential transplantation. Tissue Eng 2001; 7: 359–61
- Peters W, Irving J, Letts M. Long-term effects of neonatal bone and joint infection on adjacent growth plates. J Pediatr Orthop 1992; 12: 806–10
- Peterson H A. Operative correction of post-fracture arrest of the epiphyseal plate. Case report with ten-year follow-up. J Bone Joint Surg (Am) 1980; 62: 1018–20
- Peterson H A. Partial growth plate arrest and its treatment. J Pediatr Orthop 1984; 4: 246–58
- Phieffer L S, Meyer R A, Jr, Gruber H E, Easley M, Wattenbarger J M. Effect of interposed periosteum in an animal physeal fracture model. Clin Orthop 2000, 376: 15–25
- Synder M, Harcke H T, Conard K, Bowen J R. Experimental epiphysiodesis: magnetic resonance imaging evaluation with histopathologic correlation. Int Orthop 2001; 25: 337–42
- Tobita M, Ochi M, Uchio Y, Mori R, Iwasa J, Katsube K, et al. Treatment of growth plate injury with autogenous chondrocytes. A study in rabbits. Acta Orthop Scand 2002; 73: 352–8
- Wolff D A, Stevenson S, Goldberg V M. S-100 protein immunostaining identifies cells expressing a chondrocytic phenotype during articular cartilage repair. J Orthop Res 1992; 10: 49–57