Abstract
Background Limited data exist on the performance of low-viscosity cement in clinically realistic cadaver models.
Methods Paired stem/cement/femur constructs were generated with low-viscosity and standard-viscosity cements. The constructs were created and tested under simulated in vivo conditions, for which novel techniques were developed during this study. Mantle function was quantified by stem/cortex micromotions over 105cycles of “stair-climbing”. Mantle morphology was determined from transverse sections.
Results Penetration of low-viscosity cement was greater proximally but less distally (p = 0.02). Low-viscosity cement resulted in more stem retroversion (p = 0.04), but there was no difference in subsidence (p = 0.4). Low-viscosity cement mantles had greater fractions of non-apposed interface (p = 0.006). Fraction of non-apposed interface predicted stem retroversion (R2 = 0.64, p = 0.002).
Interpretation Low-viscosity cement resulted in inferior cement mantles. Early micromotion was reduced by better interface apposition. The greater stem retroversion of low-viscosity cement would probably lead to higher revision rates. Early stem migration is due to interface non-apposition. Techniques should be developed to reduce non-apposition of cemented interfaces.
The aim of this study was to assess the mechanical effectiveness of low-viscosity cement for hip arthroplasty using a clinically realistic cadaver model incorporating blood back-pressure. The effect of cement viscosity on the success of total hip replacement (THR) has been the subject of discussion and experimentation for some time. Cement viscosity is influenced by many factors, some arising from the composition of the cement and some being due to the storage conditions, preparation method and timing of use. Although much has been published on the subject, the literature contains contradictions and has yet to reach an evidence-based consensus. Of the cements that are incontrovertibly low-viscosity, data from clinical follow-ups have been published for 2: LVC and Osteopal. An analysis of 508 patients followed for 7–9 years using the Swedish National Arthroplasty Register revealed that clinical outcomes were poor for LVC (Granhed et al. Citation1991). In contrast, after a prospective randomized trial comparing Osteopal with Palacos in 226 patients followed for 5 years, Carlson et al. (Citation1993) reported that cement viscosity had no measurable effect on implant loosening. The authors speculated that the poor results for LVC might have been due to the technical difficulties of handling very-low-viscosity cement.
Given the conflicting clinical data, we decided that it would be useful to investigate the effect of cement viscosity in a cadaver model using standard clinical cement techniques and simulated blood back-pressure. The present study evaluated mantle morphology and function in a cadaver model of the stem/cement/femur construct using low-viscosity and standard-viscosity cements. Cement mantle function was established by measuring micromotions in response to fatigue loading. Cement mantle morphology was measured in transverse sections. We hypothesized (1) that low viscosity cement would result in greater stem/bone micromotion; (2) that low-viscosity cement mantles would have more cement penetration; (3) that low-viscosity cement mantles would have more interface gaps; and (4) that interface gaps would correlate with micromotion.
Material and methods
Specimen preparation
8 pairs of stem/cement/femur constructs were prepared from cadaver femora, which had been screened for pathological abnormalities (mean age 75 (68–83) years). The cadaver tissue was obtained through the Anatomical Gift Program of our teaching hospital. Low-viscosity cement (Osteopal; Biomet-Merck, Sjöbo, Sweden) and standard-viscosity cement (Simplex-P; Stryker, Mahwah, NJ) were randomly assigned to left and right femora. All femora were implanted with an appropriate size of collared, satin-finished (Ra = 0.35 μm), macro-textured cobalt-chrome stem with centralizer (Eon; Stryker)—4 pairs of size 8, and 4 of size 6. Stems were the same shape; size 6 stems were 11 mm shorter than size 8 stems.
The femora were stripped of all soft tissue and the distal third was removed. Neck resection and broaching was carried out according to the surgical procedures defined by the stem manufacturer. After broaching, the femoral canal was vigorously cleaned by bottlebrush lavage under warm running water. Care was taken to ensure identical preparation procedures across pairs. The femora were then “potted” distally in a block of acrylic cement. The position of the femur in the pot was carefully controlled by a jig attached to the final broach; this jig ensured that the center of the femoral head would be consistently positioned relative to the pot. After potting, the femora were fitted with an appropriately sized distal plug.
We have observed that the mobility of marrow in cadaver specimens is strongly influenced by temperature, so it seemed reasonable to assume that clinically realistic cement flow would require that the cadaver bone be at physiological temperature. In addition, the final form of curing cement is critically dependent on its mechanical and thermal boundary conditions. Thus, methods were devised to simulate in vivo fluid conditions during specimen preparation (Race et al. Citation2002).
During implantation, a realistic fluid/thermal environment was created by surrounding the femur with blood analog at 37°C (). The shear-thinning viscosity characteristics of blood were simulated by incorporating a water-soluble long-chain polymer (polyacrylamide) into the blood analog, which was a solution of the following components: 0.9 wt% NaCl, 0.08 wt% polyacrylamide, 0.0057 wt% CaCl2, 0.1 v% Rocal (a bacteriostatic agent), and 0.1 v% methylene blue. The fluid was contained in a neoprene and acrylic chamber that was fixed to the bone. The chamber was connected to an elevated reservoir of blood analog to give a simulated venous back-pressure of 3.5 kPa. This was the maximal back-pressure reported from intraoperative studies (Benjamin Citation1987). This system allowed blood analog to flow through the cortex and drain from the canal via the neck resection. Conversely, cement pressure could drive blood/marrow out through the cortex and into the surrounding fluid.
Figure 1. Apparatus to simulate physiological conditions during cement application and stem insertion. The femur was encased in a chamber created using neoprene and acrylic tubing. The proximal neoprene cowl was fixed to the femur using cyanoacrylate adhesive. The chamber was filled with a blood analog at 37°C and connected to an elevated reservoir, which raised the pressure to 3.5 kPa. Stem position was controlled by a sliding clamp.
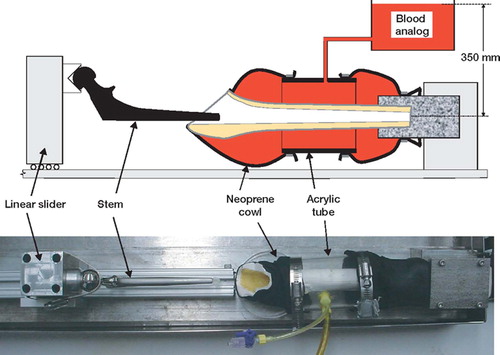
The cements were vacuum-mixed and introduced with a cement gun, using retrograde fill, in the middle of the manufacturers' recommended application times (4.0 min for standard viscosity and 3.7 min for low viscosity, at 21°C ambient temperature). The apparent viscosity at this time (measured at 100/sec shear-rate) averaged 150 Pa. s and 30 Pa.s for standard viscosity and low viscosity, respectively. Following common clinical practice, a narrow suction tube collected blood analog and marrow as the canal was filled with cement. The canal was pressurized for 30 sec using the cement gun and a proximal seal. Immediately following cement pressurization, the stem was inserted. This was done using a sliding rail system () to ensure that the center of the femoral head was properly located. The construct was clamped in position for 30 min prior to removal. The constructs were subsequently allowed to cure and hydrate for two weeks in 37°C blood analog. When specimens were removed from the fluid for mechanical testing, they were wrapped in wax film to prevent dehydration.
Mechanical testing
In vivo cyclic fatigue loading was simulated using loads representative of stair climbing (Race et al. Citation2003, Citation2005). A single-axis loading frame (Mini Bionics 858 with FlexTest IIm control and data-acquisition unit; MTS Systems Corp., Eden Prairie, MN) applied cyclic loads, via a custom fixture, to the prosthetic femoral head and the greater trochanter (). Physiological temperature was maintained during testing by wrapping a heated water circuit around the femur (). Temperature was monitored by a small thermocouple imbedded into the greater trochanter. Bone size and quality varied between donors, so appropriate loads had to be chosen for each pair of constructs in order to reduce femoral cortex failure (Race et al. Citation2005). Peak loads were adjusted such that initial valley-peak crosshead displacements were the same (to within ± 10%) for all specimens. This meant that stiffer, stronger constructs were subject to greater applied loads. The baseline normal displacement was defined by a synthetic composite femur (3rd Generation Large Composite Femur; Pacific Research Laboratories Inc., Vashon, WA) with an assumed body weight of 75 kg, loaded to a head-load of 3 body-weights (2.2 kN). When the contralateral femur had already been tested, the previously determined load was used for the second femur. Load was applied sinusoidally at 3 Hz until either 1 million cycles had been reached, the bone fractured, or the limit of the previously tested contralateral specimen had been reached.
Figure 2. Fatigue loading system. Loads representative of stair climbing were applied to the femoral head and greater trochanter. An array of LVDTs, attached to the cortex, monitored the position of a triad of spheres, which were attached to the femoral stem (inset) via a portal through the cortex and cement mantle. During testing, physiological temperature was maintained by wrapping the bone with a heated water circuit. Hydration was preserved by covering the bone with wax film.
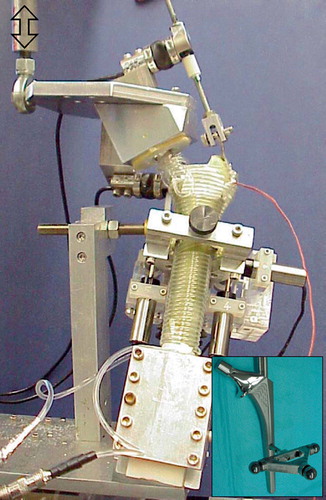
A triad of steel reference spheres was rigidly attached to the stem by a 6-mm diameter rod, 93 mm distal to the center of the implant head (). The rod passed through a 10-mm diameter hole drilled into the posterior side of the femur. The position of the triad was monitored by an orthogonal array of six linear variable displacement transducers (LVDT) that were rigidly attached to the cortex. The LVDT data were used to calculate the translations of the point of intersection between the long-axes of the stem and triad support rod relative to the femoral cortex in the same transverse plane (Berzins et al. Citation1993). The rotations of the stem about the intersection point were also calculated. Data were recorded at the peaks and valleys of the applied load. Micromotion data were described in an anatomical coordinate system based on the femoral stem. Permanent stem migrations were estimated from the “valley” micromotion data.
Two migrations were used as outcome measures in the current study: subsidence and retroversion. These variables were chosen because they represented the most commonly reported clinical motions that have been shown to correlate with revision risk in clinical RSA studies (Kärrholm et al. Citation1997).
Morphological analyses
In order to determine cement mantle morphology, specimens were transversely sectioned using a water-irrigated abrasive saw (Buehler Isomet 2000; Buehler, Inc., Lake Bluff, IL); cuts were made 10 mm, 40 mm, 80 mm and 100 mm distal to the collar of the femoral stem. The entireties of these surfaces were digitally imaged at a resolution of 6 μm/pixel using a microscope (Nikon SMZ800; Nikon Instruments Inc., Melville NY) with ring illuminator mounted onto a computer-controlled milling machine (Benchman 2000; Light Machines, Manchester, NH).
The measure of cement penetration was fractional fill of the endosteal space at each section level. Since the amount of cancellous bone varied in the superior/inferior direction, these data were kept separate and not averaged into whole bone measurements. The fractional areas of gaps at the cement/bone interface, at the stem/cement interface and in mid-mantle voids were estimated using standard stereological methods (randomly positioned regular point grids covering four transverse slices).
A further morphological measure, more directly related to interface function, was the fraction of non-apposed interface. This was defined as the linear fraction of the stem/cement or cement/bone interface that was not in apposition. The techniques for estimating this parameter have been described previously (Race et al. Citation2005). The fraction of non-apposed stem/cement interface was simple to measure: for each section, the lengths of gaps along the perimeter of the stem were summed and the total was divided by the stem perimeter. The values for the sections were then averaged to give the fraction of non-apposed stem/cement interface for each stem/cement/femur construct. The interdigitation of cement with cancellous bone made the estimation of the fraction of non-apposed cement/bone interface more complicated. The effective degree of apposition was estimated as follows. An ellipse was fitted to the cement/bone interface and 50 lines, normal to the perimeter, were distributed evenly around the ellipse. A count was made of lines that passed through fully non-apposed regions. For each line that passed through mixed regions, a value of one-half was added to the count. For each section, an estimate of the fraction of non-apposed cement/bone interface was given by the total count divided by 50.
Statistics
The hypothesis that micromotions would be greater for low-viscosity cement than for standard viscosity cement was tested using ANCOVA of subsidence and retroversion, with cement type as a factor and log10(cycles) as covariate. The hypothesis that cement penetration would be greater for low-viscosity cement than for standard-viscosity cement was tested using ANCOVA of fractional fill with cement type as a factor and section level as covariate.
The hypothesis that low-viscosity cement would result in more interface gaps was tested by multiple paired t-tests for fractional area of gaps and for fraction of non-apposed interface. For each group of t-tests, p-values were corrected using Hochberg's Bonferroni procedure for multiple comparisons (Hochberg Citation1988). Because sample numbers were small, this hypothesis was also tested using the Wilcoxon signed rank test, which is less powerful for normal data but independent of distribution. The hypothesis that interface gaps would be predictive of micromotions was tested by linear regressions of fraction of non-apposed interface with subsidence and retroversion as dependent variables.
Results
Although the original intention was to run the experiments for one million cycles, all of the specimens experienced earlier cortical failure. Most failures were due to the unavoidable non-physiological stress concentrations at the abductor insertion. One femur suffered abductor failure at 14,000 cycles, and the remaining seven pairs survived at least 105cycles. The latter time point was used for analyses. In one pair, both femora suffered mid-shaft spiral fracture and so could not be analyzed for morphology.
Subsidence was minimal for both groups and not statistically significant (). All stems rotated into retroversion, significantly further as loading continued (). The low-viscosity specimens displayed significantly more retroversion ().
Figure 3. Graphs showing mean stem migrations for low-viscosity and standard-viscosity cements (n = 7 pairs). Bars represent SD. (a) Stem subsidence vs. cycle number. ANCOVA: log10(cycles) (p = 0.1); cement type (p = 0.4); cement*cycles (p = 0.4). (b) Stem retroversion vs. cycle number. ANCOVA: log10(cycles) (p < 0.0001); cement type (p = 0.04); cement*cycles (p = 0.2).
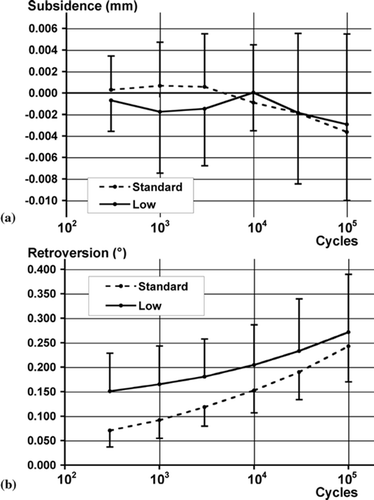
Cement penetration was significantly dependent on section level; the fractional fill of the endosteal space increased in going from proximal to distal (). There was no difference in the mean fractional fill, which was 83% for both cement types, but the effect of section level was significantly different. Low-viscosity cement had greater penetration in the most proximal section but less in distal sections ().
Figure 4. Graph of cement fill vs. axial position of transverse section. The percentage fill was the fraction of the available endosteal space occupied by the cement mantle. Low-viscosity cement resulted in greater penetration proximally but less distally (n = 6 pairs). Bars represent SD. ANCOVA: cement type (p = 0.07); section (p < 0.0001); cement*section (p = 0.02).
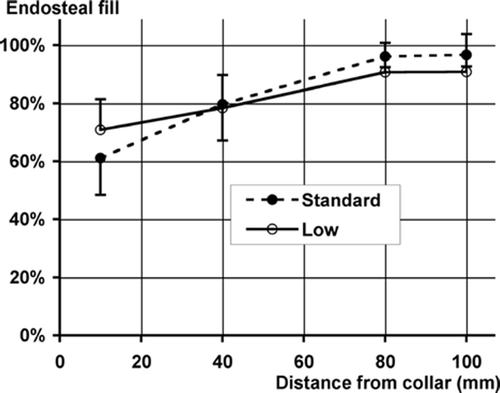
The total fractional area of gaps was significantly higher in the low-viscosity group (). The fractional area of gaps at the stem/cement interface and mid-mantle were similar for low-viscosity and standard-viscosity groups. At the cement/ bone interface, the fractional area of gaps was significantly higher in the low-viscosity group. In the interdigitated regions of the low-viscosity mantles, we often found gaps that were clearly caused by marrow/fluid inclusions rather than cement shrinkage ().
Figure 5. White light micrographs of proximal transverse sections showing interdigitated regions. (a) Standard-viscosity cement mantle. Narrow gaps can be seen between the trabeculae and the cement (arrows). These gaps were consistent with cement shrinkage. (b) Low-viscosity cement mantle. Parts of the low-viscosity mantles resembled those made with standard-viscosity cement. However, the low-viscosity mantles alone displayed extended regions where it was clear that fluid/marrow had not been displaced by the advancing cement. This resulted in gaps (arrows) that were readily distinguishable from those caused by cement shrinkage.
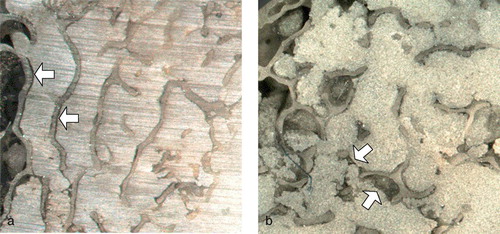
Table 1. Gap and void data (mean and SD), measured in transverse sections. Void area was expressed as a fraction of cement area, and interface non-apposition as a fraction of interface perimeter. P-values are for paired t-tests (Wilcoxon signed ranks test led to the same results in terms of statistical significance at α = 0.05, but with higher p-values)
The total fraction of non-apposed interface was significantly greater for low-viscosity cement (). The fraction of non-apposed stem/ cement interface was significantly higher for low-viscosity cement mantles but the fraction of non-apposed cement/bone interface was not. Final stem retroversion was best predicted by the total fraction of non-apposed interface ( and ). Interface non-apposition was not predictive of final stem subsidence ().
Figure 6. Graph of final stem retroversion versus total fraction of non-apposed interface. Lines show linear regression fit with 95% confidence intervals.
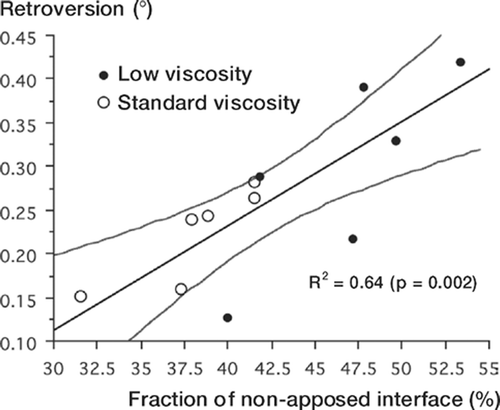
Table 2. Linear regression analyses showing the degree to which interface non-apposition predicted stem migrations. Total interface non-apposition was a good predictor of stem retroversion
Discussion
Interpretation of the bone-cement literature has been made more difficult by the fact that there is no standard definition of “low viscosity”. Both Sulfix-6 and CMW3 have often been described as low-viscosity, although their viscosities during the working phase were similar to Palacos (Noble and Swarts Citation1983) and Simplex (Watkins Citation2003, Race et al. Citation2005), respectively. Because of the perception of poor results for low-viscosity cements (largely based on inappropriate data), their use has been substantially reduced. However, we believe that a sizeable minority of surgeons continue to insert stems into lower-viscosity cement, believing that this will enhance the stem-cement bond—particularly for roughened stems (Shepard et al. Citation2000).
Previous workers have reported that in studies of isolated bone plugs, lower-viscosity cement increases the penetration of cement into cancellous bone (Noble and Swarts Citation1983, Rey et al. Citation1987). Greater cement penetration has been considered desirable, as it was thought that this would increase the strength of the cement-bone interface, as has been found in laboratory studies (Stone Citation1996, Mann et al. Citation1997, Reading et al. Citation2000). In shear-strength tests of cement-bone interface specimens created in vitro using clinical techniques (including stem insertion), it has been shown that low-viscosity cement results in increased interface strength (Bannister and Miles Citation1988). However, the same study found no difference in shear-strength when the bone surfaces were first contaminated with blood.
Other workers have warned that low-viscosity cement may be more susceptible to displacement by active bleeding, which could potentially weaken the cement-bone interface. An in vivo study of cement penetration into human femoral heads (prior to neck resection for arthroplasty) showed that active bleeding reduced cement penetration (Juliusson et al. Citation1995). Benjamin (Citation1987) used a simple plastic model to demonstrate that cement can be displaced by bleeding pressures equivalent to those that had been recorded intraoperatively. The displacement of cement by back-pressure was worse with low-viscosity cement. Majkowski (Citation1994) reported that in a simplified in vitro bovine model, simulated bleeding reduced the strength of the cement-bone interface when low-viscosity cement was used (although it did not reduce cement penetration).
In the present study, which included venous back-pressure, we found that low-viscosity cement increased penetration proximally but reduced it to some extent distally. This finding is in agreement with a previous cadaver study that did not include bleeding effects (Reading et al. Citation2000). The penetration of cement into cancellous bone is affected by both its viscosity and the pressure driving it. In the studies that used isolated bone plugs, pressure was generated by an external piston, so greater penetration by lower-viscosity cement would be expected. The peak pressure in the clinical situation, which is generated by stem insertion (Markolf Citation1976, Song Citation1994, McCaskie Citation1997), is limited by the flow of cement back out of the canal. Since the flow rate of the cement is a function of its viscosity, the pressure within the canal is also a function of viscosity. This means that higher viscosity cements can generate higher pressures in the distal canal, which allows them to penetrate further. Proximally, near the open end of the canal, there can be less difference in pressure, so lower-viscosity cements will penetrate further. In addition to flowing back out of the canal, cement can also flow out of the cortex via the vascular system. Clinically, this manifests as “posterior distal cement extrusion” (Knight Citation1999). In the present study, this was observed once in a low-viscosity specimen. The tendency of low-viscosity cement to “take the path of least resistance into the venous system” has been dramatically demonstrated using an in vivo ovine model (Breusch et al. Citation2002). In that study, lower-viscosity cement showed significantly less penetration of cancellous bone but a greater tendency to enter the vascular system.
The finding that low-viscosity cement was less able to displace blood/marrow within the cement-bone interface was to be expected. However, we were surprised to find a difference in the fraction of non-apposed stem-cement interface. We had expected that the low-viscosity cement would wet the stem better and resist pulling away as the polymerizing PMMA shrank. We now believe that lower viscosity made it easier for shrinkage voids to nucleate at the stem-cement interface and that this factor outweighed the greater wetting of the stem. It is possible that the balance between these two factors would be different for other stem surface finishes.
The most striking finding of the present study was that stem rotation correlated with interface non-apposition. This suggests that interface apposition may be more important than cement penetration. This contention is supported by recent work on the strength of the cement-bone interface. Miller et al. (Citation2004) found that, for cement-bone specimens generated under clinically realistic conditions, interface strength correlated with cement-bone apposition but not with cement penetration.
Experimental limitations
The cement techniques used in this study represented the “worst reasonable case” for low-viscosity cement. Bleeding back-pressure was set to the highest level reported from intraoperative studies and the commonly given advice to maintain pressurization and delay stem insertion was not followed. Later stem insertion was not practiced, since it would have removed part of the reported clinical motivation for using low-viscosity cement—better wetting of the stem. We observed a few instances where it was clear that backflow had occurred, displacing cement and causing a large (up to 5mm diameter) defect in the cement mantle. These defects may have been prevented by maintaining pressurization for a longer time and delaying stem insertion. It should be noted that these defects had only a small effect on the fraction of non-apposed interface, as they were localized.
We used bottlebrush lavage under warm running water. If we had used pulsatile lavage, the differences found between the cement-bone interfaces may have been reduced. With pulsatile lavage, the failure of low-viscosity cement to displace marrow would have been less evident, since there would have been less marrow to displace. However, the more open cancellous structure may have increased the effect of “blood” backflow. Cement pressurization and stem insertion rate were controlled manually. (Stem position was controlled by the sliding rail system; see ). Whilst this mirrors clinical practice, the variability of the data could have been reduced by using a reproducible automated system to pressurize and/or control stem insertion rate.
With regard to morphological measurements, our technique for measuring cement-bone interface non-apposition was somewhat arbitrary. It is possible that more direct measurements of interface quality could be developed that would correlate better with mantle function. Due to the time-frame of mechanical testing, our model could not determine the effects of long-term creep. We demonstrated that differences in interface non-apposition have an immediate effect on stem motion, but long-term creep could amplify or reduce this effect. Determination of creep effects will require further work.
Stem micromotions were measured relative to the femoral cortex. We could not determine the relative contributions of elastic deformation, stem/ cement slip and cement/bone slip. Since we found substantial non-apposition at both interfaces, which correlated with retroversion, it seems reasonable to believe that slip occurred at both interfaces. However, demonstrating this conclusively will require further work. Based on microscopic observations of serial sections, and the fact that motions were small, we do not believe that outright failure of the cement-bone interface or bone contributed much to the motions measured.
An important experimental limitation was the lack of biological response to loading. It is possible that biological factors would have increased the differences between the cement types. In some interdigitated areas, it appeared that the low-viscosity cement had failed to displace fluid/marrow as it flowed through trabecular bone (). This resulted in numerous voids adjacent to trabecular bone. The resulting network of cement/bone voids would have allowed greater space for osteoclasts, the activity of which might have been increased by debris and/or fluid pressure cycling generated by loads across these poorly integrated volumes of cement and bone. If trabecular bone had been resorbed from these regions, the remaining discontinuous cement structure would have been vulnerable to fatigue failure, which in turn might have led to greater stem migration.
Clinical relevance
Early stem retroversion has been shown to correlate with revision risk (Kärrholm et al. Citation1994), so the results of this study suggest that low-viscosity cement would result in higher stem revision rates. The exact relationship between retroversion and revision is unclear. The maximum retroversion from this study was substantially lower (about 50%) than that measured in a similar study using a cement with a clinical record of elevated revision risk (Race et al. Citation2005).
The most important result of the present study is that, in agreement with past reports (Race et al. Citation2005), stem retroversion is predicted by interface non-apposition (). This suggests that the results of cemented arthroplasties could be improved by developing techniques to ensure consistent interface apposition.
Figure 7. Graph of final stem retroversion vs. total fraction of non-apposed interface, including previously published data from the study of Race et al. (Citation2005). Lines show linear regression fit with 95% confidence intervals. The previous study used the same stem and loading protocol, but different specimen preparation techniques.
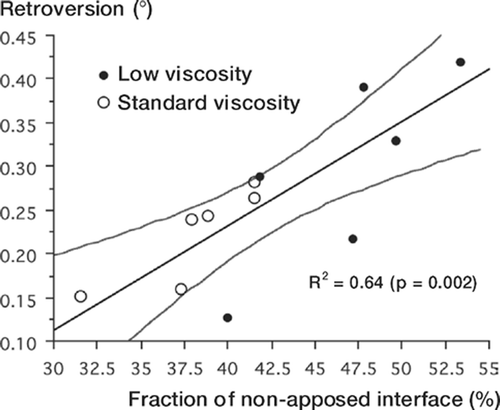
This study was made possible by a grant from Stryker Orthopaedics. The sponsor did not participate in the design of the study or the evaluation of results. The authors wish to thank Sameer Gupta, and Michael Lee for their assistance in image analysis.
Contributions of authors
The study was conceived by PAH and designed by AR and KAM. AR and MAM performed the research and collected and analyzed the data. MTC oversaw the surgical procedures. AR wrote the paper incorporating input from all authors.
- Bannister G C, Miles A W. The influence of cementing technique and blood on the strength of the bone-cement interface. Engin Med 1988; 17(3)131–3
- Benjamin J B. Cementing technique and the effects of bleeding. J Bone Joint Surg (Br) 1987; 69(4)620–4
- Berzins A, Sumner D R, Andriacchi T P, Galante J O. Stem curvature and load angle influence the initial relative bone-implant motion of cementless femoral stems. Journal Orthop Res 1993; 11(5)758–69, (Erratum in J Orthop Res 1995; 13(1): 151)
- Breusch S, Heisel C, Muller J, Borchers T, Mau H. Influence of cement viscosity on cement interdigitation and venous fat content under in vivo conditions: a bilateral study of 13 sheep. Acta Orthop Scand 2002; 73(4)409–15
- Carlsson A S. Low- vs high-viscosity cement in hip arthroplasty. No radiographic difference in 226 arthrosis cases followed for 5 years. Acta Orthop Scand 1993; 64(3)257–62
- Granhed H, Malchau H, Herberts P, Johansson O. A 7-9 year follow-up of THR operated on with low viscosity cement. Acta Orthop Scand (Suppl 246) 1991; 62: 2
- Hochberg Y. A sharper Bonferroni procedure for multiple tests of significance. Biometrika 1988; 75: 800–3
- Juliusson R, Flivik G, Nilsson J, Ryd L, Önnerfalt R. Circulating blood diminishes cement penetration into cancellous bone. In vivo studies of 21 arthrotic femoral heads. Acta Orthop Scand 1995; 66(3)234–8
- Kärrholm J, Borssen B, Lowenhielm G, Snorrason F. Does early micromotion of femoral stem prostheses matter? 4-7-year stereoradiographic follow-up of 84 cemented prostheses. J Bone Joint Surg (Br) 1994; 76(6)912–7
- Kärrholm J, Herberts P, Hultmark P, Malchau H, Nivbrant B, Thanner J. Radiostereometry of hip prostheses. Review of methodology and clinical results. Clin Orthop 1997, 344: 94–110
- Knight J L. Posterior distal cement extrusion during primary total hip arthroplasty: a cause for concern. J Arthroplasty 1999; 14(7)832–9
- Majkowski R S. The effect of bleeding on the cement-bone interface. An experimental study. Clin Orthop 1994, 299: 293–7
- Mann K A, Ayers D C, Werner F W, Nicoletta R J, Fortino M D. Tensile strength of the cement-bone interface depends on the amount of bone interdigitated with PMMA cement. J Biomech 1997; 30(4)339–46
- Markolf K L. In vitro measurement of bone-acrylic interface pressure during femoral component insertion. Clin Orthop 1976, 121: 60–6
- McCaskie A W. Cement pressurisation during hip replacement. J Bone Joint Surg (Br) 1997; 79(3)379–84
- Miller M A, Race A, Gupta S, Damron L A, Mann K A. Strength of the cement bone interface depends on viscosity of cement. 2004; 29: 1434, ORS. San Francisco, California
- Noble P C, Swarts E. Penetration of acrylic bone cements into cancellous bone. Acta Orthop Scand 1983; 54(4)566–73
- Race A, Miller M A, Ayers D C, Mann K A. Simulation of in vivo conditions during preparation and testing of cadaveric stem/cement/femur constructs. The 15th Annual Symposium of the ISTA., OxfordEngland, 2002, 211
- Race A, Miller M A, Ayers D C, Mann K A. Early cement damage around a femoral stem is concentrated at the cement/bone interface. J Biomech 2003; 36(4)489–96
- Race A, Miller M A, Clarke M T, Mann K A. Cement/implant interface gaps explain the poor results of CMW3 for femoral stem fixation: a cadaver study of migration, fatigue and mantle morphology. Acta Orthop 2005; 5: 679–87
- Reading A D, McCaskie A W, Barnes M R, Gregg P J. A comparison of 2 modern femoral cementing techniques: analysis by cement-bone interface pressure measurements, computerized image analysis, and static mechanical testing. Jo Arthroplasty 2000; 15(4)479–87
- Rey R M, Jr, Paiement G D, McGann W M, Jasty M, Harrigan T P, Burke D W, Harris W H. A study of intrusion characteristics of low viscosity cement Simplex-P and Palacos cements in a bovine cancellous bone model. Clin Orthop 1987, 215: 272–8
- Shepard M F, Kabo J M, Lieberman J R. The Frank Stinchfield Award. Influence of cement technique on the interface strength of femoral components. Clin Orthop 2000, 381: 26–35
- Song Y. An in vitro study of femoral intramedullary pressures during hip replacement using modern cement technique. Clin Orthop 1994, 302: 297–304
- Stone J J. Cement viscosity affects the bone-cement interface in total hip arthroplasty. J Orthop Res 1996; 14(5)834–7
- Watkins N D. The type of cement and failure of total hip replacements. J Bone Joint Surg (Br) 2003; 85(5)775–6, author reply 6