Abstract
Background Bone defects after failed total hip arthroplasty can be reconstructed with impacted morselized bone grafts and a cemented cup. On the acetabular side, the effects on initial cup stability of washing bone grafts prior to impaction and bone graft size remain unclear. Related to these variables, cement penetration and inter-particle shear resistance have been suggested to be critical factors to ensure initial cup stability.
Methods Using a synthetic acetabular model, we studied the effects of (1) washing bone grafts prior to impaction, and (2) bone graft size on the initial stability of cemented cups. In addition, cement penetration was measured using CT scans.
Results Reconstructions with large, washed bone grafts provided the highest stability during mechanical compression and in a lever-out situation. Washing of the bone grafts had a positive effect on initial cup stability, but the size of the bone grafts appeared to be more important. Cement penetration was affected by bone graft size but not by washing.
Interpretation From a mechanical standpoint, large bone grafts that have been washed prior to impaction may be preferable in order to obtain optimal cup stability using the bone impaction grafting technique.
The loosening process associated with a failed total hip arthroplasty (THA) revision often results in massive loss of bone stock. The bone defect can be reconstructed with impacted morselized bone grafts (Slooff et al. Citation1984). The bone impaction grafting technique utilizes vigorous impaction of morselized bone grafts in contained defects. This technique has provided good long-term clinical results on the acetabular side (Gross Citation1999, Welten et al. Citation2000, Schreurs et al. Citation2001, Citation2004, Ornstein Citation2002) Initial stability of the surgical reconstruction is essential for long-term survival, and can be assessed by preclinical in-vitro testing. Reconstructive failure is mainly caused by either excessive shear or compressive stress, or a combination of both (Brodt et al. Citation1998, Bolder et al. Citation2002). Ace-tabular tilting of the cup is an example of failure due to shear forces. Excessive axial migration of the acetabular cup can be considered failure under merely compressive loading.
Mechanical tests of impacted bone grafts have been reported (Brodt et al. Citation1998, Brewster et al. Citation1999, Ullmark and Nilsson Citation1999, Ullmark 2000, Hostner et al. Citation2001, Dunlop et al. Citation2003). These were performed using relatively simple models, which possibly failed to reveal all the major factors that play a role in the initial stability of these reconstructions. This might explain why different factors such as cement penetration properties, bone graft grading and inter-particle shear resistance have independently been suggested to be critical factors in determining initial cup stability after bone impaction grafting (Brewster et al. Citation1999, Ullmark and Nilsson Citation1999, Ullmark Citation2000,Bolder et al. Citation2003, Dunlop et al. Citation2003). In turn, these factors can be influenced by several variables such as bone graft size and bone graft preparation.
The aim of this study was to determine the effects of bone graft washing and bone graft size on initial acetabular cup stability. We hypothesized that washing would remove bone marrow and fat, thereby resulting in higher cup stability due to better particle interlock and better cement penetration. Another aim was to assess what underlying mechanisms determine the stability of acetabular constructions. We used mechanical compression tests and lever-out tests (for testing under shear loading) to address these questions.
Material and methods
The in-vitro tests were performed with cylindrical synthetic acetabular models (Bolder et al. Citation2002, Citation2003) produced by Sawbones (Sawbones Europe, Malmo, Sweden). The models contained a cortical wall and a spongy core with a thickness of 3 mm and 68 mm, respectively. The porosity of 22–23% resembles that of cancellous bone. Using a 60-mm acetabular reamer, a central cavitary defect was created in all acetabular models. Next, a segmental defect was created using a template. This segmental defect involved about 25% of the acetabular wall and was contained using a flat X-change metal mesh (Stryker Orthopaedics, Limerick, Ireland) and 4 AO cortical bone screws (). The combined defects we created in these models were comparable to AAOS type 3 defects observed in revision surgery. For RSA measurements, 4 tantalum markers were press-fit inserted and glued into standardized placed drill holes in the acetabular model, while 8 other tantalum markers were press-fit inserted and glued into standardized drill holes in the acetabular cup.
To reconstruct the defects using the impaction bone grafting technique, 40 freshly frozen human femoral heads were bisected. From all femoral head parts, large morselized cancellous bone grafts (8–12 mm) were harvested (nibbled with a rongeur) and all of these bone grafts were collected in a single pool of bone grafts to compensate for bone graft variability. Thereafter, this pool was divided into two parts: one for large grafts (8–12 mm) and one for small grafts. Small bone grafts were prepared by downsizing the large bone grafts with the flnest rasping blade of a bone mill (Noviomagus bone mill; Spierings Medical Technologies, Nijmegen, the Netherlands). Afterwards, these downsized bone grafts were pooled and sieved to produce uniformsized grafts. The size of these small bone grafts ranged from 2 to 4 mm. Subsequently, in the group of large grafts and the group of small grafts, 50% of the bone grafts were washed. In this way, 4 test methods (each applied to 5 models) were created to reconstructthe simulated defect in the synthetic models (). To wash the grafts, they were placed in a sieve and rinsed with 2 L of saline directly before the experiment. In this experiment, we used the Surgilav Puls lavage set (Stryker Orthopaedics). The bone grafts were first impacted against the mesh and subsequently into the entire defect using metal acetabular impactors (46 mm, 48 mm and 50 mm in diameter) and a metal hammer.
Table 1. Properties of the test group.
At the end of the reconstruction procedure, a standardized impaction was performed by dropping a weight of 1.5 kg 10 times, from a height of 35 cm, on a 46-mm-diameter impactor. The entire process created a graft layer of 4 mm inferiorly and 10 mm superolaterally. After impaction, Simplex-P bone cement (Stryker Orthopaedics) was prepared. 4 min after mixing the powder and the monomer, the cement was inserted in the reconstructed defect. The cement was pressurized for 2 min with a 65-mm-diameter acetabular seal (DePuy International, Blackpool, UK). Next, the acetabular cup (Stryker Contemporary cup, outer diameter 40 mm, inner diameter 28 mm) was inserted under displacement-controlled conditions, using an MTS loading device (MTS Systems Corp., Minneapolis, MN). In this way, the cups were placed at the same position in all models. Afterwards, the reconstructed models were wrapped in saline-soaked cloths and stored at 6°C for 24 h to allow the cement to polymerize.
Compression test
For the mechanical compression tests, the acetabular cups were oriented under 45 degrees abduction and loaded using an MTS machine. Dynamic loads (frequency 1 Hz) of 0–1,500 N and 0–3,000 N, combined with a constant rotation torque (applied to the rim of the cup around its axis of symmetry) of 3 Nm (Walker and Gold Citation1971) were applied for 15 min at each level (). After loading, the grafts were unloaded for 15 min to allow for visco-elastic recovery.
Figure 2. Loading schedule during the mechanical compression test.Three loading periods were applied of 15 min each.First a dynamic load ranging from 0 N to 1,500 N was applied.Subsequently the model was loaded with a dynamic load ranging from 0 N to 3,000 N after it remained unloaded (0 N) during the last 15 min.
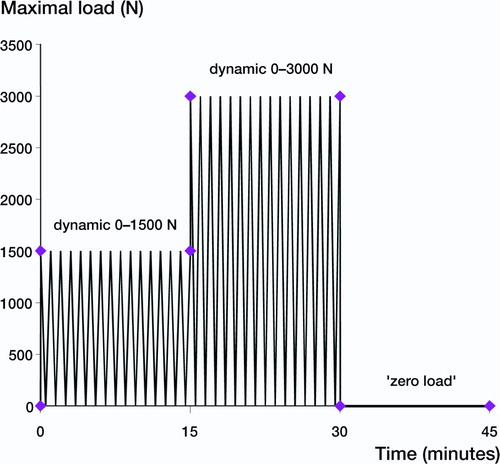
RSA was used at each loading step to measure 3-D cup migration relative to the acetabular model. The RSA measurement error was calculated with repeated examination of measurements. The accuracy was 0.01–0.03 mm for translation and 0.01–
0.08 degrees for rotation. Translations and rotations around the x-axis (medial-lateral), y-axis (cranial-caudal) and z-axis (dorsal-ventral) were calculated and combined into one cup displacement value (mm) to quantify the migration of the cups.
Lever-out test
After the mechanical compression test, the reconstructions were exposed to a lever-out test, which was primarily designed to study the stability of the reconstruction under shear loading conditions. During the lever-out test, the acetabular models were fixed at 8 degrees relative to an upright position (). A metal rod was connected to the inner surface of the cup. Subsequently, a force was applied perpendicular to the rod axis at a height of 150 mm by means of a wire connected to the MTS machine by a pulley (). The lever-out test was displacement controlled (16°/min) and the required force was monitored. We defined a sharp reduction in measured force as failure of the reconstruction. The force was transformed to a lever-out moment in Nm.
Cement penetration
All the lever-out tests resulted in a loosened ace-tabular cup. All cups, with their intact bone cement layer, were cleaned from adherent bone graft and immersed in a 30% potassium chloride solution for 6 h at 55°C to dissolve all remnants of bone. The cups were subsequently scanned using a CT scanner (Siemens Somatom Volume Zone, Siemens Erlangen, Germany) with a slice thickness of 1.0 mm and an image distance of 0.5 mm. We developed a custom-made software program to quantify the roughness profile of the cement layer, based on a 3-D CT reconstruction (Crowninshield et al. Citation1998). The assumption was made that roughness is a good indicator of cement penetration. Thus, the rougher the cement mantle, the greater is the cement penetration in the graft layer. To quantify the roughness, the outer surface of the cement was first 3-D reconstructed and then 50 sections through the axis of symmetry were used (each spaced at 3.6 degrees; ). The roughness was calculated on each section and finally an average value was obtained from the 50 slices.
Statistics
We used a linear mixed model with repeated measurements to analyze differences in the mean level of cup displacement during mechanical compression. Displacement (mm) was the dependent variable. Random variation was allowed between the acetabular models (i.e. random intercept).
The independent class variables were: size of the bone grafts (large and small), washing conditions (washed and unwashed), and loading condition (6 levels: start 1,500 N, 1,500 N after 15 min, start 3,000 N, 3,000N after 15 min, start 0 N and 0 N after 15 min). The interaction term between size and loading was included in the model, as this was the only significant first-order interaction term. The estimated mean levels of displacement (with 95% confidence intervals) were calculated by model and by each loading condition. Post-hoc, the appropriate adjusted Tukey-Kramer contrast test was used to determine differences in mean levels.
We used two-way analysis of variance (ANOVA) to analyze differences in the mean level of lever-out (Nm) and cement penetration (mm) separately. Again, the independent class variables were bone graft size and bone graft washing conditions. Post-hoc, the Tukey-Kramer contrast test was used to determine differences in mean levels. SPSS statistical software version 9 was used for the analyses.
Results
During testing, a technical error occurred in 2 specimens. Unfortunately, both errors involved reconstructions with small and unwashed bone grafts. We refrained from using these models in the lever-out test.
Average cup displacement values due to the mechanical loading test were 0.9 mm and 1.85 mm at loading levels of 1,500 N and 3,000 N (). Large washed bone grafts showed significantly less migration during loading, compared to all other groups. Statistical analysis of the linear mixed model showed systematic differences for the factors size (p = 0.02) and washing (p = 0.08), indicating that significantly less cup migration was obtained with large and washed bone grafts.
Figure 5. Mean cup displacement (mm) during loading and subsequent unloading, using linear mixed model analysis. SW: small, washed bone grafts; SU: small, unwashed bone grafts; LU: large, unwashed bone grafts; LW: large, washed bone grafts.
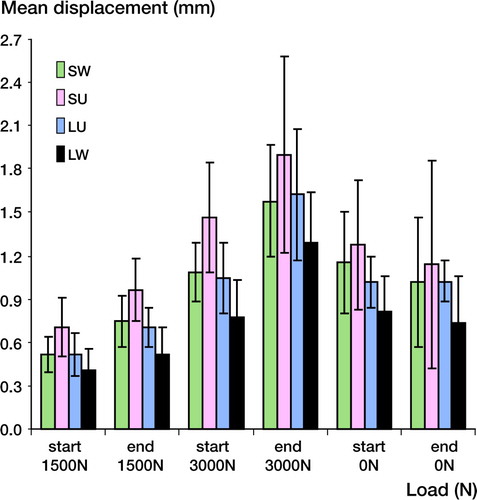
The lever-out test showed that reconstructions with large washed bone grafts provided an average lever-out resistance moment of 11.45 Nm (). This was significantly higher than for all other groups (p < 0.05). Washing of the bone grafts had a positive effect with both bone graft sizes (p = 0.045 for large bone grafts and p = 0.06 for small bone grafts).
Table 2. Mean lever-out moment values (SD) at model failure, and depth of cement penetration
In summary, for the small grafts, we could find no statistically significant evidence that washing had a positive effect on initial cup stability—either in the mechanical compression test or in the lever-out test. For the larger bone grafts, washing did show a significant improvement on initial cup stability in both tests.
Analysis of the CT images showed that all groups possessed an average cement layer thickness of approximately 0.45 cm (SD = 0.12) around the cup. Cement penetration depth, however, depended on bone graft chip size (). We found average cement penetration depths of 1.4 mm for the large bone grafts and 0.9 mm for the small bone grafts (p < 0.001). Washing did not increase depth of cement penetration for either small (p = 0.1) or large (p = 0.1) bone graft sizes.
Discussion
Loading of THA implants is generally a combination of shear and compression, both on the ace-tabular and the femoral side. With the mechanical compression test we mainly studied compressive stress, although a 3 Nm rotational loading component was also applied, generating shear stresses. In the lever-out test we mainly studied the reconstructive stability under shear loading conditions. Both the mechanical compression test and the lever-out test showed that acetabular defects reconstructed with large, washed human bone grafts provided the best stability.
The artificial models in our study represented a simplified human acetabulum. These models are suitable to simulate clinical conditions, as demonstrated by Bolder et al. (Citation2003), even though they may deform differently as compared to the human pelvic bone and do not include a bleeding bone bed. Artificial models have the advantage of producing highly reproducible results, so the number of specimens in a test group need not be high. Despite the use of this reproducible model, we found a rather large standard variation for lever-out moment values in the groups containing large bone grafts. This was most likely caused by cement penetration up to the metal mesh in a few specimens. In these cases, a higher lever-out force was obtained. This could be an important clinical finding, supporting the use of large bone grafts for acetabular bone impaction grafting. However, clinically, this excessive cement penetration is a potential risk factor, because it could hamper revascularization of the graft layer (Bolder et al. Citation2002). Despite these differences between the specimens, we believe that our findings give important insights into the mechanisms contributing to initial cup stability.
The initial cup stability ofcemented cups after acetabular bone impaction grafting is affected by many parameters, because failure of a cemented cup can occur either at the bone graft-host bone interface, the bone graftcement interface, or in the bone graft layer itself. Based on the residual materials in the model and on the cemented cup, we believe that failure occurs in the bone graft layer. Cement penetration and inter-particle shear resistance are important factors in cemented cup stability and they can be influenced by both bone graft size and bone graft washing. We identified bone graft size as the more influential factor—confirming the findings of Ullmark and Nilsson(Citation1999), who concluded from experiments using simplified models that larger-sized bone grafts would lead to a more stable graft bed. Previously, Bolder et al. (Citation2003) reported more stable acetabular reconstructions with large bone grafts. Our findings are also supported by long-term clinical results using the bone impaction grafting method on the acetabular side with large bone grafts (Welten et al. Citation2000, Schreurs et al. Citation2001, Citation2004).
We found a positive effect of bone graft washing on the initial cup stability. Washing did improve the stability without increasing the cement penetration, suggesting that inter-particle shear resistance may be a more important factor than cement penetration in determining reconstructive stability. In addition to other studies that have reported advantageous mechanical effects of bone graft washing (Ullmark and Nilsson Citation1999, Ullmark 2000), Dunlop et al. (Citation2003) also reported enhanced mechanical strength of reconstructions with washed bone grafts and explained this as being due to the increased friction characteristics between bone graft particles. In turn, this increase was thought to be due to the removal of fat and marrow, allowing tighter impaction. Next to these mechanical advantages, biological advantages of washing such as reducing the risk of bacterial infection (Hirn et al. Citation2001) and promotion of bone graft incorporation (Hostner et al. Citation2001, van der Donk et al. Citation2003)have been reported; thus, washing may be clinically advisable.
In conclusion, we found that inter-particle shear resistance was positively influenced by both washing the bone grafts and using large-sized bone grafts. Cement penetration was hardly affected by washing the bone grafts. On the contrary, cement penetration is mainly influenced by bone graft size. Hence, large bone grafts probably facilitate a high lever-out force by allowing a higher inter-particle shear resistance and deeper cement penetration—and can therefore be critical for initial cup stability. Based on the fact that failure occurs in the bone graft layer, it is likely that initial acetabular cup stability is mostly determined by inter-particle shear resistance whereas cement penetration may not be as important.
From a mechanical standpoint, we advocate using large bone grafts that have been washed prior to impaction, in order to achieve optimal cup stability when using the bone impaction grafting technique.
This study was sponsored by a grant from the Dutch Technology Foundation (STW; NKG 5061). It was also sponsored in part by Stryker Orthopaedics, Limerick, Ireland. The femoral heads used in the study were generously donated by the Leiden Bone Bank Foundation (Leiden, the Netherlands). The authors also wish to thank Huub Peters, Rene van der Venne, and Willem van de Wijdeven for their technical assistance.
Author contributions
JJCA did most of the work. NV supervised the project from a biomechanical perspective. PB supervised the project from a biological perspective. BWS supervised the project from a surgical perspective.
- Bolder S B, Verdonschot N, Schreurs B W, Buma P. Ace-tabular defect reconstruction with impacted morselized bone grafts or TCP/HA particles. A study on the mechanical stability of cemented cups in an artificial acetabulum model. Biomaterials 2002; 23: 659–66
- Bolder S B T, Schreurs B W, Verdonschot N, Van Unen J M J, Gardeniers J W M, Slooff T J J H. Bone graft particle size and method of impaction influence initial stability of cemented cups in bone impaction grafting. Acta Orthop Scand 2003; 74(6)652–7
- Brewster N T, Gillespie W J, Howie C R, Madabhushi S P G, Usmani A S, Fairbairn D R. Mechanical considerations in impaction bone grafting. J Bone Joint Surg (Br) 1999; 81: 118–24
- Brodt M D, Swan C C, Brown T D. Mechanical behaviour of human morsellized cancellous bone in triaxial compression testing. J Orthop Res 1998; 16: 43–9
- Crowninshield R D, Jennings J D, Laurent M L, Maloney W J. Cemented femoral component surface finish mechanics. Clin Orthop 1998, 355: 90–102
- Dunlop D G, Brewster N T, Madabhushi S P G, Usmani A S, Pankaj P, Howie C R. Techniques to improve the shear strength of impacted bone graft. J Bone Joint Surg (Am) 2003; 85: 639–46
- Gross A E. Revision arthroplasty of the acetabulum with restoration of bone stock. Clin Orthop 1999, 369: 198–207
- Hirn M Y, Salmela P M, Vuento R E. High-pressure saline washing of allografts reduces bacterial contamination. Acta Orthop Scand 2001; 72: 83–5
- Hostner J, Hultmark P, Kärrholm J, Malchau H, Tveit M. Impaction technique and graft treatment in revisions of the femoral component: laboratory studies and clinical validation. J Arthroplasty 2001; 16: 76–82
- Ornstein E. Hip revisions with impacted morsellized allograft bone and cement. Patient outcome, prosthetic fixation and risks. Acta Orthop Scand 2002; 73: 1–66, (Suppl 306)
- Schreurs B W, Slooff T J, Gardeniers J W, Buma P. Ace-tabular reconstruction with bone impaction grafting and a cemented cup: 20 years'experience. Clin Orthop 2001, 393: 202–15
- Schreurs B W, Bolder S B, Gardeniers J W, Verdonschot N, Slooff T J, Veth R P. Acetabular revision with impacted morsellised cancellous bone grafting and a cemented cup. A 15- to 20-year follow-up. J Bone Joint Surg (Br) 2004; 86(4)492–7
- Slooff T J, Huiskes R, van Horn J, Lemmens A. Bone grafting in total hip replacement for acetabular protrusion. Acta Orthop Scand 1984; 55: 593–6
- Ullark G. Bigger size and defatting of bone chips will increase cup stability. Arch Orthop Trauma Surg 2000; 120: 445–7
- Ullmark G, Nilsson O. Impacted corticocancellous allografts: recoil and strength. J Arthroplasty 1999; 14: 1019–23
- Walker P S, Gold B L. The tribology (friction, lubrication and wear) of all-metal artificial hip joints. Wear 1971; 17: 285–99
- Welten M L M, Schreurs B W, Buma P, Verdonschot N, Slooff T J J H. Acetabular reconstruction with impacted morselized cancellous bone autograft and cemented primary total hip arthroplasty. A 10-17 year follow-up study. J Arthroplasty 2000; 15: 819–24
- van der Donk S, Weernink T, Buma P, Aspenberg P, Slooff T J J H, Schreurs B W. Rinsing allografts improves bone and tissue ingrowth. Clin Orthop 2003, 408: 302–10