Abstract
Background and purpose Bones used in mechanical studies are frequently harvested from human cadavers that have been embalmed in a buffered formaldehyde solution. It has been reported that formaldehyde fixation or freezing hardly affects the mechanical properties of bone after a storage period of several weeks. However, human cadaver bones are usually stored for longer periods of time before use. We therefore investigated the effects of long-term embalming or freezing on the mechanical properties of cortical bone.
Methods After 5 different storage periods (ranging from 0 to 12 months), goat femora and humeri were used to evaluate the effect of embalming and freezing on torsion, and on bending stiffness and strength. The effect on hardness and bone mineral density (BMD) was also evaluated.
Results Even after 1 year, no statistically significant differences could be found in stiffness, strength, and energy absorption when we compared embalmed or frozen bones to a fresh reference group. In addition, although we found no significant change in BMD, there appears to be a tendency to increasing hardness.
Interpretation We found that there was no effect on the mechanical properties of bone after storage periods of 1 year. We conclude that embalmed or frozen bones can safely be used for mechanical testing, at least for storage periods of up to one year.
Cadaver bones are widely used in studies investigating the mechanical behavior and efficacy of orthopedic devices and trauma devices (Gollwitzer et al. Citation2004). Given the difficulty in obtaining a sufficient number of fresh bones (at least in Europe), the increasing degree of complexity regarding logistics, and the risk of transmission of pathogens to investigators working with fresh bones (Cavanaugh and King Citation1990, Healing et al. Citation1995, Duma et al. Citation1999, Sterling et al. Citation2000, Nogler et al. Citation2001, Demiryurek et al. Citation2002), embalmed or frozen bones are an attractive alternative. Cadaver bones also have advantages over artificial bones, as the handling and the mechanical properties of cadaver bones match reality in the best possible way, whereas composite bones differ in some of their mechanical characteristics (Cristofolini and Viceconti Citation2000).
Generally, bones are harvested from human cadavers that have been embalmed in a buffered formaldehyde solution and stored for long periods of time before use. Studies have already been performed to investigate the effects of embalming or freezing on the mechanical properties of bones (McElhaney et al. Citation1964, Sedlin Citation1965, Pelker et al. Citation1984, Panjabi et al. Citation1985, Roe et al. Citation1988, Goh et al. Citation1989, Linde and Sorensen Citation1993, Currey et al. Citation1995, Kang and Kim Citation1995, Hamer et al. Citation1996). However, in these studies the maximum storage time was 3 months, whereas cadaver specimens are generally stored for much longer periods. We investigated the long-term effects of embalming or freezing on the mechanical properties of bone.
Materials and methods
Study design
30 mature Dutch milk goats were killed for studies unrelated to ours (a lumbar spine fusion study, with no effects expected on bone metabolism or mechanical properties). The femora and the humeri on both sides were harvested. Soft tissue was left in situ, thereby preventing dehydration of the bones and mimicking the normal storage conditions.
All left femora and humeri were allocated to the frozen group. These specimens were stored in plastic bags at −20°C until testing. Before the mechanical tests were performed, the specimens were thawed to room temperature. All right femora and humeri were assigned to the embalmed group. Embalming was performed by injecting the soft tissue under high pressure with a 10% buffered formaldehyde solution, which is the standard solution in our institution. The specimens were stored in container filled with this solution until testing. At 0, 1.5, 3, 6, and 12 months, mechanical tests were performed on the bones (6 goats per time-point). The humeri were used for a torsion test, and the femora were used for a 4-point bending test. During the tests, the specimens were kept moist with gauze soaked in an isotonic saline solution. After the tests, rings of cortical bone from the proximal part of the diaphysis of the humeri and femora were used for hardness measurements.
Directly after killing and before mechanical testing, a bone mineral density (BMD) measurement was done (DEXA-scan, QDR-2000; Hologic Corporation, Waltham, MA). All femora were measured in order to monitor any demineralization of the bones.
Four-point bending
A previously described and validated 4-point bending method was used (Bramer et al. Citation1998). Both ends of the femora were placed in cylindrical metal cups. The axis of the bone was centered by positioning the condyles and the middle of the collum in the center of the cups. Fixation took place by filling the cups with a low-melting-point bismuth alloy (A301; Degussa, Wolfgang, Germany) (47°C) in the liquid state. The specimens were then placed in a material-testing device (Instron 8872; Instron Corporation, Canton, MA), which recorded the applied loads and displacement online. The orientation of the bones in the testing device was standardized. The 4-point bending test was performed by applying two equal forces to the edges of the cups. To prevent tension in the axial direction of the bones due to displacement, the cups were allowed to translate in the horizontal plane under bending of the specimen (). The load was applied with a constant cross-head speed of 1 mm/ min. The test was destructive, so the ultimate bending moment as well as bending strength could be assessed.
Figure 1. The 4-point bending device. The force (F) was applied with a cross-head speed of 1 mm/min. The applied load (F) and the vertical displacement (d) were recorded online by the Instron machine. The angular displacement was calculated by dividing the cotangent of the vertical displacement (d) by the length of the lever arm (x = 43 mm). The bending moment was calculated by multiplying half of the applied load by the length of the lever arm.
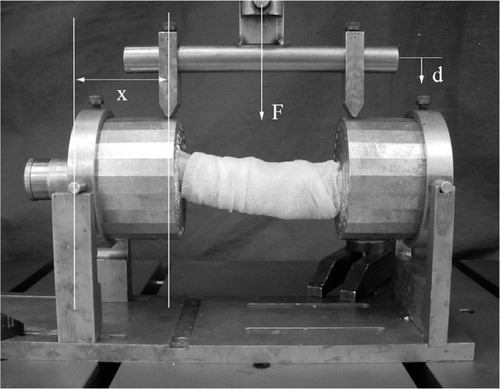
Torsion
For the torsion tests, both ends of the humeri were placed in cylindrical cups, the axis of the bones were centered identically as in the four-point bending test, and the bones were fixed with the bismuth alloy. The humeri were placed in a torsion-testing device, with the distal cylindrical cup rigidly fixed in the testing device (). The proximal part was allowed to rotate in a suspension system. Via an arm of 100 mm, an increasing load was applied perpendicular to the axis of the bones until failure. The angular velocity was set at 5 degrees per min. During both the 4-point bending tests and torsion tests the specimens were kept moist with gauze soaked in an isotonic saline solution.
Figure 2. Schematic drawing of the torsion device. Both ends of the specimen (Sp) were placed in cylindrical cups, and the axes of the bones were centered. The proximal part was allowed to rotate in a suspension system, and the distal part was rigidly fixed. Via an arm of 100 mm, an increasing load (F) was applied perpendicular to the axis of the bones until failure. The angle rotation was set at 5 degrees/min. During the test, the vertical displacement (d) and the applied load were recorded. The angular displacement was calculated by dividing the cotangent of the vertical displacement (d) by the length of the lever arm (100 mm).
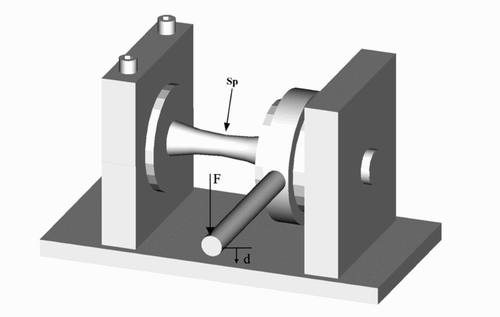
Processing of the test results
The stiffness of the bones was calculated from the vertical displacement and the applied load recorded by the Instron. Angular displacement was calculated by taking the cotangent of the vertical displacement divided by the length of the lever arm (in 4-point bending 43 mm, and in torsion 100 mm). Torsion and bending moments were calculated by multiplying the applied load by the length of the lever arm. With the least-squares method, a regression line was fitted to the linear part of the plot between the angular displacement and the moment (). The slope—the inclination of the regression line—is given as a tangent. A slope of higher value means a higher material stiffness.
Figure 3. Typical angular displacement vs. moment curve from a torsion test. There is a correlation between the slope of the regression line (R) and the stiffness of the bone. The area under the curve is a measure of the energy absorption. The highest point of the regression line represents the strength (St).
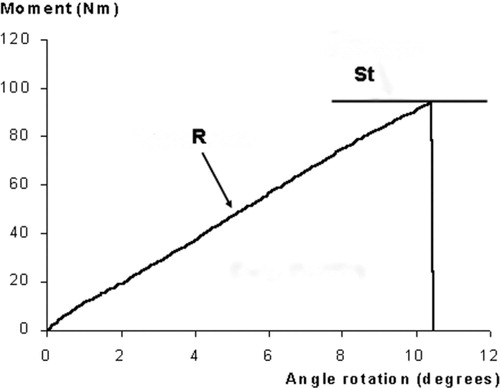
Bending strength (Sb) was calculated by multiplying half of the applied load (F) by the distance between the point of suspension and the point of contact on the edge of the cup (x = lever arm = 43 mm): Sb = 0.5 F · x.
The torsion strength (St) was calculated by multiplying the applied load (F) by the length of the lever arm (x = 100 mm): St = F · x.
The area under the curve between the angular displacement and the bending or torsion moment is a measure of the energy absorption under deformation.
To correct for the individual variability between the bones, the BMD values were used to correct the data from the torsion and the 4-point bending tests, by dividing the slope and the strength values by the BMD.
Measurement of hardnes
After the mechanical tests, rings of cortical bone were machined with a water-cooled diamond saw (Exact, Norderstedt, Germany) at standardized points. These rings were polished with progressive grades of silicon carbide paper and finished with 9- μm diamond polisher slurry until the osteons were clearly visible by microscopy. The samples were cleaned in an ultrasonic water bath, and embedded exactly horizontally with a specially designed press. A Vickers automatic hardness measurement device (Buehler 1600–6400; Bluehler, Lake Bluff, IL) was used to perform 6 measurements on each specimen. Indentations were made randomly with a diamond indenter and an indent weight of 50 g (Broz et al. Citation1995). Ominimet MHT software (Bluehler, Lake Bluff, IL) was used to analyze the indentations.
Statistics
To analyze data between time groups, ANOVA tests, with an LSD-post hoc test, were performed. To analyze differences between the embalmed and frozen bones within one time group, paired Student t-tests were used. Significance was set at p-values of < 0.05.
Results
In the 4-point bending tests, no statistically significant differences in stiffness were found between the reference group and the different time groups for both the frozen and embalmed bones. In addition, the differences in bending strength between the different time groups and the reference group were not statistically significant ( and ). When comparing the test results for the embalmed bones with those for the frozen bones within one time group, no difference could be found in either stiffness or bending strength in any of the time groups ( and ). In the torsion tests also, no statistically significant differences in stiffness and strength could be found for both the frozen and the embalmed bones, when comparing the reference group to all other time groups ( and ). Furthermore, comparison of the embalmed bones with the frozen bones for any particular time group did not reveal any statistically significant differences in stiffness or bending strength in any of the time groups ( and ).
Figure 4. Results of the 4-point bending tests and torsion tests for the frozen group and the embalmed group. For both groups, the average slope of the regression line of the plot between the moment and angular displacement (N/degree) and the strength (Nm) are given. All values have been corrected for BMD. The post hoc test between the reference group and the different time groups did not show any significance. A: reference group; B: 1.5-month group; C: 3-month group; D: 6-month group; E: 12-month group.
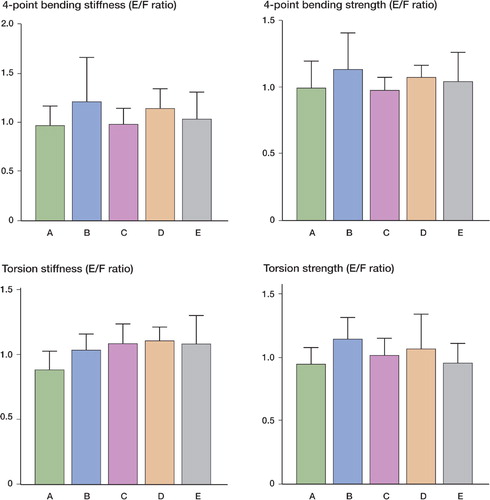
Figure 5. The ratios of the embalmed (E) and frozen (F) bones for both stiffness and strength in the 4-point bending and torsion tests. In the reference group, the ratio between values for left and right bones is shown. For both types of test, no significant differences were found between the embalmed and the frozen bones, or the left and right bones, in any of the groups. A: reference group; B: 1.5-month group; C: 3-month group; D: 6-month group; E: 12-month group.
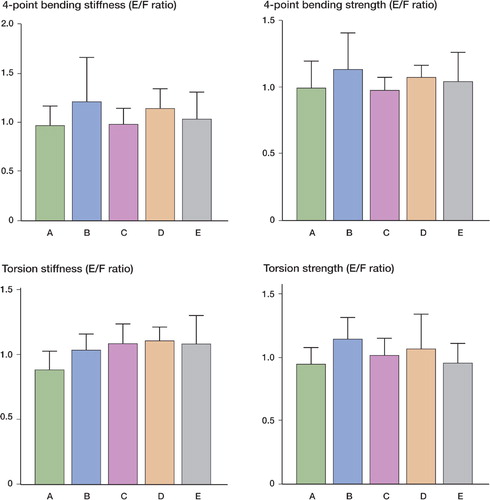
Results for the 4-point bending and torsion tests. All results have been corrected for bone mineral density. Values in parenthesis are the 95% confidence intervals. Post hoc analysis did not reveal any significant differences between the reference group and other time groups, or between freezing and embalming
In the reference group, the stiffness in the 4-point bending tests of the left bones and the right bones was 53 degrees/N and 57 degrees/N, respectively. The strength of the left bones and the right bones was 260 N and 272 N, respectively. Neither parameter was statistically significant (Student's t-test, p = 0.6 and 0.7). In addition, no left-right differences were found in stiffness or in strength for the torsion tests (p = 0.2 and 0.5).
No differences could be found in energy absorption values during bending and torsion for all the groups, except for an increase in torsion in the 3-month frozen group (p = 0.04). The average BMD of the reference group was 0.76 (SD 0.13) g/cm2. No statistically significant differences were found between the reference group and all other time groups. Also, no significant differences were found when comparing the BMD values before and after the follow-up periods within one group (paired Student t-tests; p-values between 0.07 and 0.9).
The average hardness of femora in the reference group was 68 (SD 7.9) Vickers, and 63 (SD 4.4) Vickers for humeri in the reference group. No significant difference was found between the average hardness of both femora and humeri in the reference group and the 1.5-, 3-, and 6-month groups. With an average hardness of 80 (SD 6.8) Vickers, femora in the 12-month frozen group had greater hardness than those in the reference group (p = 0.007). For femora in the 12-month embalmed group and for humeri in the 12-month embalmed and frozen groups, significance was not reached (p = 0.06, 0.07, and 0.07, respectively) ().
Figure 6. Hardness of the humeri and femora in the time groups. The femora of the 12-month frozen group were harder than in the reference group (a p = 0.007); no significant differences were seen for the embalmed bones (p = 0.06). No significant difference was found in any of the other groups in comparison with the reference group, although the 12-month frozen and 12-month embalmed groups for the humeri were almost statistically significantly harder (p = 0.07 and 0.07, respectively). A: reference group; B: frozen group; C: embalmed group.
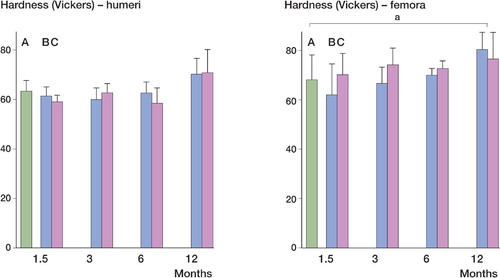
Discussion
Several studies have already evaluated the effect of different storage methods on the mechanical properties of bone (McElhaney et al. Citation1964, Pelker et al. Citation1984, Roe et al. Citation1988, Goh et al. Citation1989, Linde and Sorensen Citation1993, Kang and Kim Citation1995, Currey et al. Citation1995, Wilke et al. Citation1996), but the maximum storage time in these studies was 3 months. Cadaver bodies are generally stored for 1 year or more after they are embalmed. It is quite possible that the effect of the embalming fluid on the mechanical properties of bone becomes effective after longer storage periods. As little is known about the long-term effects of embalming or freezing, and since it is important that the mechanical properties of these bones should closely reflect the in vivo situation, we have evaluated the mechanical effects of longterm preservation of cortical bone by embalming and freezing.
Embalming took place by injecting the soft tissue with embalming solution under high pressure; the specimens were then placed in containers with embalming solution. This is somewhat different from the procedure of fixing human cadavers, as in that case the embalming solution is injected into the vascular system of the cadavers. Thus, it can be expected that a higher penetration rate of embalming fluid in the bones would be reached. However, by injecting the soft tissue under high pressure and placing the specimens in a container with embalming fluid, fast diffusion of embalming fluid in the bone can also be expected.
We used standardized, destructive 4-point bending and torsion tests to evaluate the effects of embalming and freezing on the mechanical properties of long bones. The mechanical tests were performed after 4 different storage periods, with a maximum follow-up of one year. Thus, the timedependent changes in stiffness and strength could be followed within the course of one year, and it showed that neither stiffness nor strength in torsion and bending were influenced by freezing or embalming over this period.
In each time group, the embalmed bone had a paired frozen counterpart from the same goat. At all time points, we could therefore make a paired comparison between the embalmed and the frozen bones. This comparison did not reveal statistically significant differences for any test at any time point. Thus, neither embalming nor freezing had any influence on the mechanical properties of cortical bone over time, and there was no difference in the direct comparison between embalmed and frozen bones at each time point.
In this observational study, a power analysis could not been done. Although we had a rather small number of goats per time group (n = 6), the 95% confidence intervals showed an acceptable distribution of the results. We therefore consider our results to be reproducible and valid.
The left and right bones were not randomly allocated to the frozen and embalmed groups, because we did not expect left-right differences based on findings in the literature (Bramer et al. Citation1998, Jonsson and Stromberg Citation1984). This was confirmed, as no significant left-right differences for bending and torsion were found in the reference group.
No statistically significant difference was found between the BMD directly after killing and after embalming or freezing for each time interval. These results indicate that the mineral content is not affected by embalming or freezing. This is in agreement with reports by Boskey et al. (Citation1982) and Lochm et al. (Citation2001), who found no effect of formaldehyde fixation on the mineral parameters of bone. As there is a correlation between BMD and the mechanical properties of bone (Alho et al. Citation1988, Wachter et al. Citation2001, Citation2002, Hernandez et al. Citation2001), BMD was used to correct for the individual variability between bones.
The hardness measurements on the femora revealed a statistically significant increase in hardness in bones that were frozen for 12 months relative to the reference group, whereas no statistically significant differences could be found between the other groups. Although the average degrees of hardness of the femora that were embalmed for 12 months and of humeri that had been embalmed or frozen for 12 months were not statistically different from their reference group, the average hardness was relatively high.
Our finding that freezing does not alter the mechanical properties of bone is in agreement with the findings of other authors (Sedlin Citation1965, Pelker et al. Citation1983, Citation1984, Panjabi et al. Citation1985, Goh et al. Citation1989, Hamer et al. Citation1996). Investigations on the effects of embalming on the mechanical properties of bone found no or little effect after short to intermediate storage periods. Currey et al. (Citation1995) used a brief (3-hour) fixation protocol and found almost no effect in loading tests, but a significant reduction in impact strength. Sedlin (Citation1965) reported that the tensile elastic modulus of human femoral cortical bone following three weeks of fixation in 10% formalin was the same as for fresh specimens. Goh et al. (Citation1989) investigated the effects of freezing and formaldehyde fixation for 3 and 21 days on the mechanical properties of cat bones. Torsion and bending stiffness, and ultimate strength, were not found to be affected by formaldehyde fixation or freezing, whereas storage in formalin reduced the energy absorption value during torsion by 40%, and during bending by 50%. This contrasts with our findings, as we did not find any statistically significant changes in energy absorption values. Only the energy absorption in the three-month frozen group was significantly increased. As this is an isolated finding, not supported by any increase in energy absorption in the 6- and 12-month groups or by any change in mineral density, we interpret this as a coincidence. There was a high portion of relatively small animals in the study by Goh et al., which might explain their findings.
We conclude that bones used for studies on the mechanical behavior of orthopedic and trauma devices can be preserved by formaldehyde fixation or freezing for up to 1 year without any ill effect.
Contributions of authors
EHH: design of study, performance of mechanical tests, processing of data, writing of the manuscript. BCZ: design of study, performance of biomechanical tests, processing of data (statistical analysis). AJV: design of study, performance of biomechanical tests, processing of data (biomechanical engineering). ICH, PIJMW: design of study, assistance during manuscript preparation. THS: design of study, processing of data (biomechanical engineering), manuscript preparation, principal investigator.
No competing interests declared.
- Alho A, Husby T, Hoiseth A. Bone mineral content and mechanical strength. An ex vivo study on human femora at autopsy. Clin Orthop 1988, 227: 292–7
- Boskey A L, Cohen M L, Bullough P G. hard tissue biochemistry: a comparison of fresh-frozen and formalin-fixed tissue samples. Calcif Tissue Int 1982; 34: 328–31
- Bramer J A, Barentsen R H, vd E M, de Lange E S, Patka P, Haarman H J. Representative assessment of long bone shaft biomechanical properties: an optimized testing method. J Biomech 1998; 31: 741–5
- Broz J J, Simske S J, Greenberg A R. Material and compositional properties of selectively demineralized cortical bone. J Biomech 1995; 28: 1357–68
- Cavanaugh J M, King A I. Control of transmission of HIV and other bloodborne pathogens in biomechanical cadaveric testing. J Orthop Res 1990; 8: 159–66
- Cristofolini L, Viceconti M. Mechanical validation of whole bone composite tibia models. J Biomech 2000; 33: 279–88
- Currey J D, Brear K, Zioupos P, Reilly G C. Effect of formaldehyde fixation on some mechanical properties of bovine bone. Biomaterials 1995; 16: 1267–71
- Demiryurek D, Bayramoglu A, Ustacelebi S. Infective agents in fixed human cadavers: a brief review and suggested guidelines. Anat Rec 2002; 269: 194–7
- Duma S M, Rudd R W, Crandall J R. A protocol system for testing biohazardous materials in an impact biomechanics research facility. Am Ind Hyg Assoc J 1999; 60: 629–34
- Goh J C, Ang E J, Bose K. Effect of preservation medium on the mechanical properties of cat bones. Acta Orthop Scand 1989; 60: 465–7
- Gollwitzer H, Karampour K, Hauschild M, Diehl P, Busch R, Mittelmeier W. Biomechanical investigation of the primary stability of intramedullary compression nails in the proximal tibia: experimental study using interlocking screws in cryopreserved human tibias. J Orthop Sci 2004; 9: 22–8
- Hamer A J, Strachan J R, Black M M, Ibbotson C J, Stockley I, Elson R A. Biochemical properties of cortical allograft bone using a new method of bone strength measurement. A comparison of fresh, fresh-frozen and irradiated bone. J Bone Joint Surg (Br) 1996; 78: 363–8
- Healing T D, Hoffman P N, Young S E. The infection hazards of human cadavers. Commun Dis Rep CDR Rev 1995; 5: R61–R68
- Hernandez C J, Beaupre G S, Keller T S, Carter D R. The influence of bone volume fraction and ash fraction on bone strength and modulus. Bone 2001; 29: 74–8
- Jonsson U, Stromberg L. Uniformity in mechanics of long bones at torque. A dog experiment. Acta Orthop Scand 1984; 55: 347–8
- Kang J S, Kim N H. The biomechanical properties of deep freezing and freeze drying bones and their biomechanical changes after in-vivo allograft. Yonsei Med J 1995; 36: 332–5
- Linde F, Sorensen H C. The effect of different storage methods on the mechanical properties of trabecular bone. J Biomech 1993; 26: 1249–52
- Lochmuller E M, Krefting N, Burklein D, Eckstein F. Effect of fixation, soft-tissues, and scan projection on bone mineral measurements with dual energy X-ray absorptiometry (DXA). Calcif Tissue Int 2001; 68: 140–5
- McElhaney J, Fogle J, Byars E, Weaver G. Effect of embalming on the mechanical properties of beef bone. J Appl Physiol 1964; 19: 1234–6
- Nogler M, Lass-Florl C, Ogon M, Mayr E, Bach C, Wimmer C. Environmental and body contamination through aerosols produced by high-speed cutters in lumbar spine surgery. Spine 2001; 26: 2156–9
- Panjabi M M, Krag M, Summers D, Videman T. Biomechanical time-tolerance of fresh cadaveric human spine specimens. J Orthop Res 1985; 3: 292–300
- Pelker R R, Friedlaender G E, Markham T C. Biomechanical properties of bone allografts. Clin Orthop 1983, 174: 54–7
- Pelker R R, Friedlaender G E, Markham T C, Panjabi M M, Moen C J. Effects of freezing and freeze-drying on the biomechanical properties of rat bone. J Orthop Res 1984; 1: 405–11
- Roe S C, Pijanowski G J, Johnson A L. Biomechanical properties of canine cortical bone allografts: effects of preparation and storage. Am J Vet Res 1988; 49: 873–7
- Sedlin E D. A rheologic model for cortical bone. A study of the physical properties of human femoral samples. Acta Orthop Scand 1965, Suppl 77
- Sterling T R, Pope D S, Bishai W R, Harrington S, Gershon R R, Chaisson R E. Transmission of Mycobacterium tuberculosis from a cadaver to an embalmer. N Engl J Med 2000; 342: 246–8
- Wachter N J, Augat P, Mentzel M, Sarkar M R, Krischak G D, Kinzl L, Claes L E. Predictive value of bone mineral density and morphology determined by peripheral quantitative computed tomography for cancellous bone strength of the proximal femur. Bone 2001; 28: 133–9
- Wachter N J, Krischak G D, Mentzel M, Sarkar M R, Ebinger T, Kinzl L, Claes L, Augat P. Correlation of bone mineral density with strength and microstructural parameters of cortical bone in vitro. Bone 2002; 31: 90–5
- Wilke H J, Krischak S, Claes L E. Formalin fixation strongly influences biomechanical properties of the spine. J Biomech 1996; 29: 1629–31