Background and purpose Hip resurfacing arthroplasty has had resurgence recently and is now a popular option for younger, active patients. We used an in vitro model to assess range of motion and impingement profile for hip resurfacing and compared them to those for conventional total hip arthroplasty.
Methods 8 different hip replacement designs were implanted into adult composite femurs and pelvises. These were mounted onto a 3-dimensional compass allowing all motions, with the degrees and impingement recorded. The designs tested were the Conserve Plus Hip Resurfacing System, Depuy ASR, Birmingham Hip Resurfacing System, Charnley, McKee-Farrar metal-on-metal, SROM Hip metal-on-metal, SROM Hip metal-on-polyethylene, Prodigy metal-on-metal, and also a native intact composite femur and pelvic articulation. Femoral stems were tested at 0 and 20 degrees of anteversion.
Results Conventional hip arthroplasty exhibited statistically significantly greater range of motion than resurfacing arthroplasty. Resurfacing showed neck impingement in 29/30 motions. Conventional arthroplasties showed femoral neck impingement in 41/100 motions.
Interpretation In situ range of motion of resurfacing arthroplasty was less than that of conventional total hip arthroplasty. Resurfacing systems impinged almost entirely on the femoral neck, while conventional hip arthroplasties had a varied impingement profile. Our findings raise concern for early neck-on-cup impingement, which may cause component loosening and femoral neck fracture, both of which are observed after hip resurfacing.
Hip resurfacing arthroplasty has been used by surgeons for over 50 years to treat arthritic and osteonecrotic hips, with varying results (Howie et al. Citation1990, Amstutz Citation1996, Rieker et al. Citation1998). Resurfacing is considered by some to be particularly attractive for a younger, more active population (Amstutz et al. Citation1998) as it is considered to be bone sparing, anatomically restorative, and potentially capable of inducing less stress shielding than conventional hip arthroplasty (Harty et al. Citation2005). Proponents suggest that if a conversion to conventional hip arthroplasty is required in the future, the conversion is easier and theoretically performs similarly to a primary hip arthroplasty rather than a revision hip arthroplasty (Ball et al. Citation2007). Long-term results after such conversion have, however, not been reported.
Resurfacing designs differ from conventional total hip arthroplasty in several respects: the retention of the femoral neck, the deletion of a load-bearing stem, and the use of large femoral head articulations. These properties approximate the native femoral head-to-neck ratio and have been proposed to be a design improvement over conventional hip arthroplasty (). However, this conflicts with the traditional tenet of head-to-neck ratio, in particular that maximizing head diameter and minimizing neck diameter enhances hip performance by allowing greater range of motion before component impingement (Chandler et al. Citation1982, Barrack Citation2003, Alberton et al. Citation2004).
Figure 1. A synthetic composite model femur with a femoral resurfacing component implanted to optimize fixation and minimize bone loss.
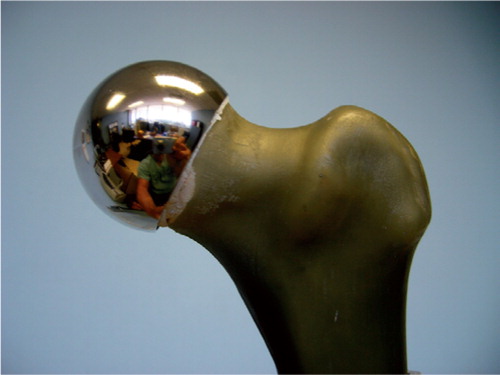
In this study, we hypothesized that the effective range of motion and mode of impingement are different for resurfacing and for conventional hip replacements. We used a model that has an advantage over clinical models, which include numerous confounding variables (e.g. soft tissue attachments), and still reflects component and anatomic restrictions on range of motion.
Materials and methods
We studied 3 contemporary hip resurfacing arthroplasty systems and 5 conventional hip arthroplasty systems representing widely implanted, historic, and current systems: A Wright Conserve Plus, Depuy ASR, Birmingham Hip Resurface, Charnley flat back, Mckee-Farrar metal-on-metal, SROM metal-on-polyethylene, SROM metal-on-metal, and Prodigy metal-on-metal. An intact, native hip model was also included ().
Table 1. Component specifications
Validated adult composite femur and pelvic models (Cristofolini et al. Citation1996) (size Adult/Large) were used to create the in vitro hip model; they were of uniform size throughout the study. All components were implanted with standard operative technique by a single surgeon using concentric acetabular reaming, oscillating saws, cylindrical femoral head reamers, and burrs.
The acetabular components were press-fitted into the pelvis with molding clay if necessary. For reasons of reproducibility, the component was placed with an orientation parallel to a plane created by 3 points along the acetabular rim. The result was an abduction angle of 45 degrees (± 2 degrees) determined by goniometry.
The femoral components were placed according to each manufacturer's recommended technique, again using molding clay. For stemmed implants, the femoral neck was cut with an oscillating saw, the canal broached and prepared for 0 or 20 degrees of anteversion with restoration of offset, and the center of the head maintained at the level of the tip of the greater trochanter (). Care was taken to ensure coaxial alignment of stem and femoral shaft. For resurfacing components, cylindrical reamers and burr were used to resect only the bone that would be replaced by the resurfacing component with particular attention paid to center of neck stem position, avoidance of femoral neck notching, and recreation of concentric and flush transition from the metal surface to the femoral neck.
Figure 2. Composite model femur with a Prodigy stem and a 32 mm head. The center of the femoral head is in alignment with the tip of the greater trochanter
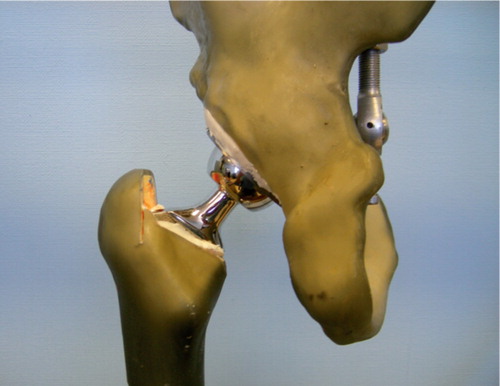
Implanted hip models were then mounted inside a 3-dimensional compass that has been used in previous publications, with a measurement accuracy of one degree (Amstutz et al. Citation1975, Chandler et al. Citation1982) (). This compass allowed controlled, selective motions of the hip, specifically rotations in the sagittal, coronal, and axial planes. The compass also allowed femoral and pelvic adjustments to establish a single, concentric point of rotation. The implanted hips were then moved in 10 different motions, with the range and point of impingement recorded. Stemmed femoral systems were tested at both 0 degrees and 20 degrees of anteversion.
Figure 3. The 3-dimensional compass used to simulate and measure rotations in the sagittal, coronal, and transverse planes, within one degree of accuracy.
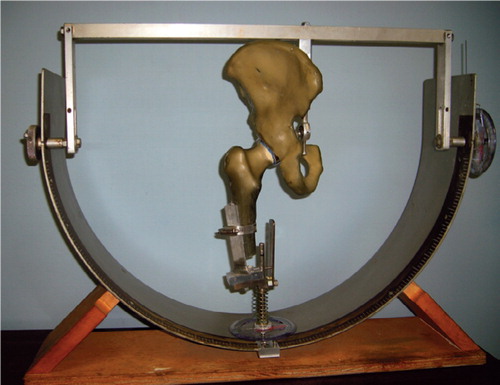
In order to minimize variation due to anatomical differences, composite model femurs and pelvises were used rather than cadaver specimens. These models provide almost identical geometry from one specimen to the next, representing typical/aver-age cadaveric anatomy and mechanical properties. One pelvis was used for all acetabular components, whereas a fresh femur was used for each type of femoral component implantation.
The range of motion of the intact femur within the acetabulum was first measured without any implant. Then, ranges of motion in each plane (10 separate motions) for each of the combinations of femoral and acetabular components were conducted. With each of the 10 motions, each measurement was repeated twice by the same observer.
Statistics
For each of the 10 motions (hyperextension, abduction, adduction, internal rotation, etc.), mean and standard error of the 2 measurements (in degrees of rotation) were plotted and compared using a separate Student's t-test for each comparison. For illustrative purposes, motions that corresponded to the same plane were added to equal the arc of motion in that plane (e.g. the degree of internal rotation was added to the degree of external rotation to equal the arc of motion in the axial plane). Impingement profiles of components were recorded as 2 groups: neck impingement (component or native) and non-neck impingement (typically greater trochanter on pelvis and lesser trochanter on pubic ring).
Results
Mean and standard error of each range of motion are depicted graphically for zero degrees of femoral anteversion, separately for those in full extension () and for those in 90 degrees of pelvic flexion (). The results are similarly depicted for 20 degrees of femoral anteversion, separately for those in full extension () and for those in 90 degrees of pelvic flexion (). In general, compared to conventional hip replacements, resurfacing arthroplasties showed less range of motion. Specifically, of the 20 movements evaluated (10 with zero femoral anteversion, and 10 with 20 degrees of femoral anteversion), conventional hip replacements had greater range of motion in 12 movements, while resurfacing implants exhibited greater range of motion in 2 movements ().
Table 2. Mean (SE) for each range of motion (in degrees), comparing conventional hip replacements to resurfacing implants
Average ranges of motion corresponding to each of the sagittal, coronal, and axial planes were then calculated by combining averages of hyperextension and full flexion, abduction and adduction, and internal and external rotation (). Resurfacing arthroplasty showed arcs of motion in the sagittal, coronal, and axial planes of 135, 78, and 115 degrees. Conventional hip arthroplasty with 0 degrees of femoral anteversion showed average arcs of motion of 174, 87, and 150 degrees. Conventional hip arthroplasty with 20 degrees of femoral anteversion showed average arcs of motion of 158, 90, and 147 degrees ( and ).
Table 3. Summed motion arcs. Values are mean degree
The impingement profile of resurfacing arthroplasty showed cup-on-neck impingement in 29/30 motions. Conventional hip arthroplasty had a more varied profile with 20/50 motions causing neck-on-cup impingement for the 0-degrees anteverted group and 21/50 motions for the 20-degrees anteverted group. The native hip showed neck impingement in 12/20 of movements.
Discussion
We found that overall, in situ hip resurfacing arthroplasty exhibits less range of motion than conventional hip arthroplasty. While not every individual motion showed this difference, the model showed that conventional hips had statistically significantly more range of motion in 12 of the 20 movements evaluated, while resurfacing exhibited increased range in only 2 movements. These data coincide with the computer model of Dr Doherty et al. from Dr. Phil Noble's group (Houston, Texas; personal communication), which suggests resurfacing range of motion is reduced in all 3 planes of motion.
Perhaps just as important, the impingement profiles of hip resurfacing were essentially all neck-on-cup impingement, whereas conventional hip arthroplasty had a more heterogenous profile.
We feel that the excellent range of motion of the native, intact hip used in our study is somewhat of an artifact and should only be used for contextual purposes when evaluating implanted hips. This artifact arose from the fact that the native diameters of the femoral head and acetabulum are less congruent than that of the instrumented hip, yielding less constraint and allowing slight subluxation, which enhanced range of motion.
The in vitro model we used has been used previously (Amstutz et al. Citation1975, Chandler et al. Citation1982). Recently, an in vitro model using only component geometry constraints was used to investigate hip stability (Bader et al. Citation2004). The model we used has the same advantage of simplicity, devoid of the numerous confounding variables found in clinical, in vivo, or cadaveric models. It does, however, retain anatomic skeletal constraints as well as component geometry constraints.
Our study has several shortcomings that are readily apparent. Most importantly, this was an in vitro study, and therefore could not incorporate the complex nature of an in vivo implanted hip. Furthermore, human hips move in concert with the lumbar spine and pelvic mobility (Perron et al. Citation2000, Watelain et al. Citation2001, Vogt et al. Citation2003), thus potentially compensating for impingement profiles of hip arthroplasty.
Another shortcoming is that the variations in anatomy or other characteristics among patients were not included. Rather, the model was constructed using standardized composite femurs and pelvises. A new composite model femur was used for each implant design, and each setup was measured twice. Consequently, the statistical analysis is based on variance in outcome due to measurement error rather than differences between patients. Such a model offers the ability to more easily detect differences in range of motion due to differences in implant design; however, this comes at the cost of making it difficult to generalize the results to patients without reference to additional clinical evidence. On the other hand, patient studies of hip range of motion typically suffer from large variations due to many confounding variables such as differences not only in anatomy but also in soft tissue characteristics, age, underlying disease, and many others, and therefore require large numbers of subjects before statistically significant differences can be detected.
Finally, our study was subject to technical confounding variables such as implantation technique. While this is unavoidable to some extent with this type of model, we sought to minimize internal variables by using several simple parameters: implanting the acetabular component in the plane of the pelvic rim, minimizing potentially biased techniques, implanting the femoral component to restore offset, maintaining the center of femoral head at the level of the tip of the greater trochanter, and ensuring a smooth transition from resurfacing head to femoral neck. We note that at the time of surgery, acetabular components may be “ideally” oriented to minimize impingement. For the purposes of this study, one reproducible position was based on the acetabular brim to avoid confounding variables.
Historically, hip resurfacing has underperformed relative to conventional total hip arthroplasty, largely due to aseptic loosening. Our findings suggest that component loosening may be caused by—or be at least partially attributable to—a relatively smaller arc of motion and a predominance of neck-on-cup impingement. This is particularly concerning given that a large proportion of the patient populsation undergoing hip resurfacing are younger and more active, thus potentially exacerbating the impingement profile of these devices.
This study was supported by the Los Angeles Orthopaedic Hospital Foundation. The authors thank Jaya Maewal for her technical contributions.
No competing interests declared.
BCB: in vitro model implantation, experiment design, and writing of manuscript. SNS: experiment design and literature research. EE: editing of manuscript, experimental design, and literature research.
- Alberton G M, High W A, Morrey B F. Dislocation after revision total hip arthroplasty: an analysis of risk factors and treatment options. J Bone Joint Surg (Am) 2004; 12(5)314–21
- Amstutz H C, Editor. Metal on metal hip prostheses: past performances and future directions. Raven Publishers, Philadelphia, 1996: S2
- Amstutz H C, Lodwig R M, Schurman D J, Hodgson A G. Range of motion studies for total hip replacements. A comparative study with a new experimental apparatus. Clin Orthop 1975, 111: 124–30
- Amstutz H C, Grigoris P, Dorey F J. Evolution and future of surface replacement of the hip. J Orthop Sci 1998; 3: 169–86
- Bader R, Scholtz R, Steinhauser E, et al. The influence of head and neck geometry on stability of total hip replacement. Acta Orthopaedica 2004; 75(4)415–21
- Ball S T, Le Duff M J, Amstutz H C. Early results of conversion of a failed femoral component in hip resurfacing arthroplasty. J Bone Joint Surg (Am) 2007; 89(4)735–41
- Barrack R L. Dislocation after total hip arthroplasty: implant design and orientation. Review. J Am Acad Orthop Surg 2003; 11(2)89–99
- Chandler D R, Glousman R, Hull D, McGuire P J, Kim I S, Clarke I C, Sarmiento A. Prosthetic hip range of motion impingement. The effects of head and neck geometry. Clin Orthop 1982; 166: 284–91
- Cristofolini L, Viceconti M, Cappello A, Toni A. Mechanical validation of whole bone composite femur models. Review. J Biomech 1996; 29(4)525–35
- Harty J A, Devitt B, Harty L C, Molloy M, McGuinness A. Dual energy X–ray absorptiometry analysis of peri-pros-thetic stress shielding in the Birmingham resurfacing hipreplacement. Arch Orthop Trauma Surg 2005; 125(10)693–5, Epub, Oct 20
- Howie D W, Campbell D, McGee M, et al. Wagner resurfacing hip arthroplasty. The results of one hundred consecutive arthroplasties after 8 to 10 years. J Bone Joint Surg (Am) 1990; 72: 708–14
- Perron M, Malouin F, Moffet H, McFadyen B J. Three-dimensional gait analysis in women with a total hip arthroplasty. Clin Biomech (Bristol, Avon) 2000; 15(7)504–15
- Rieker C B, Kottig P, Schon R, . Clinical wear performance of metal-on-metal hip arthroplasties. Alternative bearing surfaces in total joint replacements, J Jacobs, T L Craig, et al. ASTM, Conshohocken 1998; 144
- Vogt L, Brettmann K, Pfeifer K, Banzer W. Walking patterns of hip arthroplasty patients: some observations on the medio-lateral excursions of the trunk. Disabil Rehabil 2003; 25(7)309–17
- Watelain E, Dujardin F, Babier F, Dubois D, Allard P. Pelvic and lower limb compensatory actions of subjects in an early stage of hip osteoarthritis. Arch Phys Med Rehabil 2001; 82(12)1705–11