Abstract
Background Current specifications (standards) for preclinical testing of bone cements (ISO 5833: 2002, ASTM F451-99a) require simple mechanical testing after ageing for 24 h under dry conditions at 23°C. Some bone cements have fulfilled the requirements in the specifications, and yet had inferior clinical results. Clinically, bone cements are subjected to complex loading patterns in a moist or wet environment at 37°C. Thus, both the validity and the robustness of current standard testing protocols can be questioned.
Methods We examined the influence of temperature and storage medium on the properties of bone cement. We also compared the results of storage and testing under standard conditions of 23°C in dry air, with the results obtained at 37°C in water or plasma.
Results The dry specimens showed an increase in strength and elastic modulus with time, while the values of the wet ones decreased. There was no difference between specimens stored in water or in plasma. Ultimate compressive strength of dry specimens after 24 h was 1.16 times higher than that of the ones stored wet, increasing to 1.34 times after 1 month, and 1.46 times after 6 months (p<0.001 for all comparisons).
Interpretation Testing under dry conditions—as required in current standards—always gave higher values for mechanical properties than did storage and testing under more physiological conditions. The sensitivity of test values to different environments implies that testing conditions for bone cements should be scrutinized in order to develop more relevant testing protocols that reflect the in vivo environment more closely.
Bone cements are used routinely (Havelin et al. Citation1993, Malchau et al. Citation2002, Citation2005) for fixation of a variety of implants with different designs. The materials are polymeric materials usually based on methylmetacrylate (MMA) monomer and a prepolymer powder, mainly poly(methyl methacrylate) (PMMA). The arthroplasties will subject the cement to a variety of loading conditions. The selection of bone cements plays a critical role for the clinical outcome (Espehaug et al. Citation2002). The mechanical properties of bone cements are known to influence the survival of arthroplasties (Gruen et al. Citation1979), together with the surface preparation of the implant itself (Howell et al. Citation2004, Perez et al. Citation2006, Smailys et al. Citation2007).
Bone cements as materials are influenced by environmental factors such as moisture (Akashi et al. Citation1999) and temperature (Baleani et al. Citation2001), but they are also dependent on how the components of the cement have been prepared (Dunne and Orr Citation2001). Moreover, cements are technique-sensitive materials that require standardized handling in order to achieve reproducible results (Lewis and Austin Citation1994, Lewis et al. Citation2005, Nottrott et al. Citation2007).
With preclinical testing, the intention is to predict the clinical behavior of bone cements. Past experience has shown that bone cements that had adequate mechanical values according to existing specifications actually performed poorly in hip arthroplasties, as revealed by registries of implant survival (Thanner et al. Citation1995, Nilsen and Wiig Citation1996, Furnes et al. Citation1997, Carlsson Citation1998). Thus, it can be questioned whether standard preclinical testing is adequate for making a meaningful prediction about the clinical performance of bone cements. If not, then clinical trials may be required. Advanced preclinical in vitro testing such as fatigue testing has been described as a method for determination of the long-term survival of bone cements (Lewis Citation2003). There are still many areas of disagreement, which makes it necessary to standardize these test methods and conditions.
Current specifications for bone cements (Demian and McDermott Citation1998) are ISO 5833: 2002 (ISO 2002) and ASTM F451-99a (American Society for Testing and Materials 1999). According to these specifications, it is sufficient to perform simple static compressive mechanical tests after aging for 24 h under dry conditions at 23°C, which are also the recommended test conditions. Compressive strength should be at least 70 MPa. In addition, bending modulus and bending strength should be determined. Additionaly, the standards define doughing time, setting time, maximum curing temperature, packaging, and labeling.
We compared the mechanical properties of a bone cement stored up to 6 months under 3 sets of conditions: (1) standard conditions (storage and testing at 23°C in air), (2) in water (storage and testing at 37°C), and (3) in plasma (storage in plasma 37°C and testing at 37°C in water).
Material and methods
Cement and preparation
We used Palacos R cum gentamicin bone cement (Schering-Plough) (). The cement was pre-chilled to 4°C prior to mixing, and prepared at 23°C according to the manufacturer's instructions. The polymer powder was placed in a cartridge (Optivac S; ScandiMed Implant AB, Sjöbo, Sweden) into which the monomer was added. The components were mixed under vacuum conditions below 0.15 bar for about 40 s. At dough time, usually after 3.5 min approximately, the cement was injected into a Teflon mold (NIOM, Oslo, Norway) which was produced according to ISO 5833 (2002) (ISO 2002). The cement was allowed to cure for 15 min. The test specimens (6×12 mm) were removed from the mold and wet-ground (DAP-V; Struers, Copenhagen, Denmark) to ensure that the end-faces of the specimens were perpendicular to the long axis.
Chemical composition of Palacos cum Gentamicin bone cement (Schering-Plough). Information from the manufacturer
Samples were aged unloaded, in 3 different environments, until testing. Group A was aged in air at 23°C, group B in water at 37°C, and group C in fresh frozen plasma (Octaplas; Octapharma AS, Hurdal, Norway) at 37°C. Test specimens were mounted singly. In groups B and C, 4 mL of the respective fluid was used. In group B, the water was changed every second week. There were no visual signs of bacterial growth. In group C, plasma was changed every week; fungal growth was observed twice and sodium azide was then added to the plasma.
Test specimens were obtained from 3 different mixing procedures and were randomly assigned to the testing groups. All test specimens were from the same lot (batch) (Table).
Time protocol
Groups A and B were mechanically tested after 24 h, 1 month, and 6 months. 10 samples were tested at each time point in each group. The zero point was defined as the start of mixing of the components.
Group C was tested after 1 week and at 3 months; 9 samples were tested at each time point and compared to results at the same time points in a former study (Nottrott et al. Citation2007).
Mechanical testing
Cement specimens were tested in compression with a servo-hydraulic testing machine (Material Test System 810, model no. 318.10; MTS Systems Corp., Eden Prairie, MN) with a compression jig and strain transducer (custom-made by NIOM, Oslo, Norway).
The test set-up () made it possible to measure small strains in the specimens, being unaffected by overall mechanical compliance of the testing system.
Figure 1. Schematic diagram of the experimental configuration: (a) water at 37°C, (b) strain transducer, (c) test specimen.
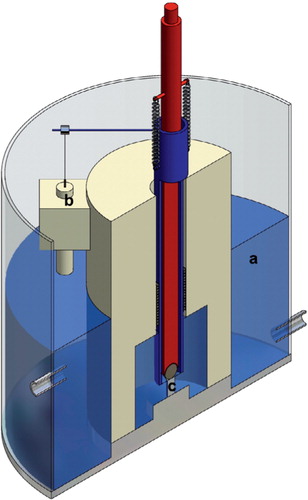
In group A, testing was performed in air at 23°C. In groups B and C, the jig was placed in a water-bath (custom-made by NIOM, Oslo, Norway) where the water temperature was kept constant at 37°C±0.5°C.
In all groups, a constant cross-head speed of 24 mm/min (ISO 2002) was employed until the cylinder fractured. Deformation and load were recorded continuously. Ultimate compressive strength, UCS (in MPa), maximum strain (in %) and E-modulus (E, in GPa), were calculated.
Statistics
We used a generalized linear model (GLM) to model an ANOVA to determine differences between the different groups, and also for the different time points in any particular group. The group differences were tested at each time point in the GLM using a categorical variable defined with an interaction of time. All p-values less than 0.05 were considered statistically significant. SPSS release 13.0 was used for the statistical analysis.
Results
Specimens in group A showed a continuous increase in ultimate compressive strength (UCS) while UCS decreased slightly in group B (). There were statistically significant differences in UCS in group A between 24 h and 1 month (p<0.001), and also between 1 month and 6 months (p=0.001). In group B, the differences in UCS between 1 week and 1, 3, and 6 months were statistically significant (p≤0.001). UCS in group A after 24 h was 1.16 times higher than in group B, increasing to 1.34 times after 1 month and to 1.46 times after 6 months. The respective differences between groups A and B at each time point of testing were always statistically significant (p<0.001).
Figure 2. Mean ultimate compressive strength (UCS) with 95% confidence intervals (CIs), on a logarithmic time scale.
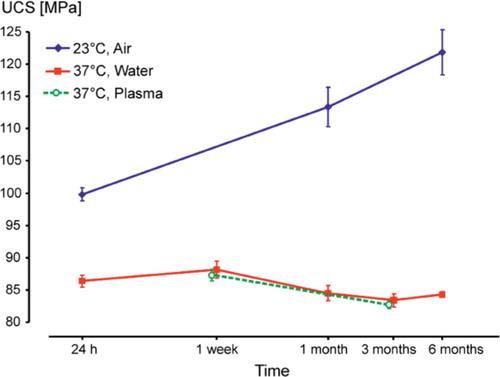
The same behavior was detected in elastic modulus (E), which increased continuously in group A whereas we observed a decrease in group B (). In group A, the differences in E between 24 h and 1 month, and between 24 h and 6 months were statistically significant (p<0.001), but those between 1 and 6 months were not (p=0.07). In group B, the differences in E between 1 week and 1, 3, and 6 months were statistically significant (p≤0.02). In group A, E after 24 h was 1.1 times higher than that in Group B, increasing to 1.31 times after 1 month and to 1.4 times after 6 months. Also here, the respective differences between groups A and B at each time point of testing were always statistically significant (p<0.001).
Maximum strain values increased in both groups but the differences were statistically significant only in group A between 24 h and 6 months (p<0.001). However, no statistically significancant differences were detected between the groups (p=0.7 at 24 h, p=0.5 at 1 month, and p=0.2 at 6 months) ().
Storage in plasma resulted in approximately identical values of ultimate compressive strength, modulus, and maximum strain to those for storage and testing in water at 37°C. The changes (decreases) in UCS () and E-modulus () were statistically significant (p<0.001), but this was not the case for changes in maximum strain values (p=0.5) ().
Discussion
We found a difference in the development of mechanical properties of a bone cement between storage in air (group A) and in water (group B), whereas there were no differences between storage in water (group B) and storage in plasma (group C). Moreover, we found that testing as recommended by the specifications (group A) always gave higher values than storage and testing under more physiological conditions (groups B and C).
The increase in strength and E-modulus in group A can be explained as being the result of ongoing polymerization and crosslinking, and the onset of physical ageing (Struik Citation1978, Hay Citation1995). For groups B and C, we assume that the slight decrease in strength and modulus between 1 week and 1 month was caused by water uptake in the cement (Ruyter and Svendsen Citation1980, Schmitt et al. Citation2004), which has a plasticizing effect (Deb et al. Citation1995, Akashi et al. Citation1999, Unemori et al. Citation2003). Thus, storage and testing at room temperature in the dry state, as recommended in specifications for bone cements, produces unrealistically high values compared with storage and testing under simulated body conditions.
Multiphase poly(methyl methacrylate) (PMMA) is sensitive to environmental temperature (Hailey et al. Citation1994, Arnold and Venditti Citation2001). This is supported by our finding that the values for 23°C were higher than those for 37°C, as also demonstrated earlier (Nottrott et al. Citation2007). The glass transition temperature (Tg) of PMMA is of importance: the Tis about 100°C in pure PMMA but in bone cement it is in the range of 60–70°C (Kuehn Citation2000, Kühn Citation2005), which is not far from the 37°C testing temperature. The low Tg of bone cements is caused by residual monomer of about 2–6% (Hailey et al. Citation1994, Kühn Citation2005). Residual monomer has a plasticizing effect on the cement, leading to reduced mechanical strength. Moreover, the effect of a difference of 14°C in testing temperature may be enhanced in the presence of water (Hailey et al. Citation1994, Arnold and Venditti Citation2001). Comparing the results of our present study with those of our former study (Nottrott et al. Citation2007), the effect of temperature on a bone cement in air appears to be less important.
After storage for 1 month in water at 37°C, the cement is nearly saturated with water and has reached stable values of strength and modulus, whereas there is a continuous increase in strength and E-modulus for cements stored under dry conditions. The change in maximum strain values, indicating brittleness, suggests that there are continous internal or external processes influencing the material.
The results of the present study confirm the recommendations given in our previous study of testing bone cements in a physiological environment after storage for at least 5 weeks (Nottrott et al. Citation2007). The use of plasma solutions seems unnecessary, as the values correspond closely with those obtained in water.
The current standards for bone cements—being reference documents for product evaluation and approval—do not mimic the conditions or environment in which the material is used clinically.
The current study investigated a single bone cement that is considered to be representative for PMMA-based materials, and which is commonly used (Havelin et al. Citation1993, Furnes et al. Citation2007, Kärr holm et al. 2007). We used a quasistatic compressive test, which represents a simplified test configuration. Still, differences were demonstrated, due to environmental factors and time span. Other test procedures may be relevant, e.g. tension, torsion, bending, and determination of viscoelastic properties and fatigue. Standardization of testing is necessary for meaningful comparison of test results (Lewis and Nyman Citation2000). The present study has addressed some basic properties of a well-established material. Considering that new materials often appear, the selection of test method could be crucial for preclinical approval.
In the absence of appropriate preclinical test methods for new bone cements, we still have to rely on data from randomized trials and registry studies, where the outcomes have a considerable lag time from product launch. Our results extend our understanding of the material behavior of bone cements, and may contribute to the development of more clinically relevant preclinical evaluation procedures.
Financial support from the Sophie Minde Foundation is gratefully acknowledged. The authors thank statistician Stein Atle Lie of the Department of Health, University Research, Bergen for his help in performing the statistical analysis.
No competing interests declared.
All authors contributed to the conception and design of the study and manuscript preparation.
In addition, individual authors contributed as follows. MN: data collection, data processing, statistical analysis, interpretation of results, and writing of the manuscript. AOM: statistical analysis, interpretation of results, and writing of the manuscript. IOM: data collection and data processing. NRG: data collection, data processing, interpretation of results, and writing of the manuscript. WRW: interpretation of results and writing of the manuscript.
- Akashi A, Matsuya Y, Unemori M, Akamine A. The relationship between water absorption characteristics and the mechanical strength of resin-modified glass-ionomer cements in long-term water storage. Biomaterials 1999; 20(17)1573–8
- American Society for Testing and Materials. Standard specification for acrylic bone cement (ASTM F451-99a). 1999
- Arnold J C, Venditti N P. Effects of environment on the creep properties of a poly(ethylmethacrylate) based bone cement. J Mater Sci Mater Med 2001; 12(8)707–17
- Baleani M, Cristofolini L, Toni A. Temperature and ageing condition effects on the characterization of acrylic bone cement. Proc Inst Mech Eng [H] 2001; 215(1)113–8
- Carlsson Å S. Comparison of different bone cements: An overview. Bimaterials in Surgery, G H I M Walenkamp. Georg Thieme Verlag, Stuttgart, New York 1998; 43–7
- Deb S, Braden M, Bonfield W. Water absorption characteristics of modified hydroxyapatite bone cements. Biomaterials 1995; 16(14)1095–100
- Demian H W, McDermott K. Regulatory perspective on characterization and testing of orthopedic bone cements. Biomaterials 1998; 19: 1607–18
- Dunne N J, Orr J F. Influence of mixing techniques on the physical properties of acrylic bone cement. Biomaterials 2001; 22(13)1819–26
- Espehaug B, Fumes O, Havelin L I, Engesaeter L B, Vollset S E. The type of cement and failure of total hip replacements. J Bone Joint Surg (Br) 2002; 84(6)832–8
- Furnes O, Lie S A, Havelin L I, Vollset S E, Engesaeter L B. Exeter and charnley arthroplasties with Boneloc or high viscosity cement. Comparison of 1,127 arthroplasties followed for 5 years in the Norwegian Arthroplasty Register. Acta Orthop Scand 1997; 68(6)515–20
- Furnes O, Havelin L I, Espehaug B, Steindal K, Sørås T E. The Norwegian Arthroplasty Register, REPORT 2007. Department of Orthopaedic Surgery, Haukeland University Hospital, BergenNorway 2007, Helse-Bergen HF, http://www.haukeland.no/nrl/
- Gruen T A, McNeice G M, Amstutz H C. “Modes of failure” of cemented stem-type femoral components: a radiographic analysis of loosening. Clin Orthop 1979, 141: 17–27
- Hailey J L, Turner I G, Miles A W, Price G. The Effect of Post-Curing Chemical Changes on the Mechanical Properties of Acrylic Bone Cement. Journal of Materials Science: Materials in Medicine 1994; 5(9–10)617–21
- Havelin L I, Espehaug B, Vollset S E, Engesaeter L B, Langeland N. The Norwegian arthroplasty register. A survey of 17,444 hip replacements 1987–1990. Acta Orthop Scand 1993; 64(3)245–51
- Hay J N. The physical ageing of amorphous and crystalline polymers. Pure & Appl Chem 1995; 67(11)1855–8
- Howell J R, Jr, Blunt L A, Doyle C, Hooper R M, Lee A J, Ling R S. In vivo surface wear mechanisms of femoral components of cemented total hip arthroplasties: the influence of wear mechanism on clinical outcome. J Arthroplasty 2004; 19(1)88–101
- ISO. International standard ISO 5833 Implants for surgery. Acrylic resin cements, 2002
- Kuehn K-D. Bone cements. Up-to-date comparison of physical and mechanical properties of commercial materials. Springer Verlag, Heidelberg 2000
- Kühn K-D. Properties of bone cement: What is bone cement. The well-cemented total hip arthroplasty. Theory and practice, S Breusch, H Malchau. Springer Medizin Verlag, Berlin 2005; 52–9
- Kärrholm J, Garellick G, Herberts P. Swedish Hip Arthroplasty Register, Annual Report 2006. Gothenburg, Sweden 2007, Department of Orthopaedics, Sahlgrenska University Hospital, www.jru.orthop.gu.se
- Lewis G. Fatigue testing and performance of acrylic bone-cement materials: state-of-the-art review. J Biomed Mater Res 2003; 66B(1)457–86
- Lewis G, Austin G E. Mechanical properties of vacuum-mixed acrylic bone cement. J Appl Biomater 1994; 5(4)307–14
- Lewis G, Nyman J S. Toward standardization of methods of determination of fracture properties of acrylic bone cement and statistical analysis of test results. J Biomed Mater Res 2000; 53(6)748–68
- Lewis G, Janna S, Bhattaram A. Influence of the method of blending an antibiotic powder with an acrylic bone cement powder on physical, mechanical, and thermal properties of the cured cement. Biomaterials 2005; 26(20)4317–25
- Malchau H, Herberts P, Eisler T, Garellick G, Söderman P. The Swedish Total Hip Replacement Register. J Bone Joint Surg (Am) (Suppl 2) 2002; 84: 2–20
- Malchau H, Garellick G, Eisler T, Kärrholm J, Herberts P. Presidential guest address: the Swedish Hip Registry: increasing the sensitivity by patient outcome data. Clin Orthop 2005; 441: 19–29
- Nilsen A R, Wiig M. Total hip arthroplasty with Boneloc(R). Loosening in 102/157 cases after 0.5-3 years. Acta Orthop Scand 1996; 67(1)57–9
- Nottrott M, Mølster A O, Gjerdet N R. Time dependent mechanical properties of bone cement. An in vitro study over one year. J Biomed Mater Res B Appl Biomater 2007; 83B(2)416–21
- Perez M A, Garcia-Aznar J M, Doblare M, Seral B, Seral F. A comparative FEA of the debonding process in different concepts of cemented hip implants. Med Eng Phys 2006; 28(6)525–33
- Ruyter I E, Svendsen S A. Flexural properties of denture base polymers. J Prosthet Dent 1980; 43(1)95–104
- Schmitt S, Krzypow D J, Rimmac C M. The effect of moisture absoption on the fatigue crack propagation resistance of acrylic bone cement. Biomed Tech 2004; 49(3)61–5
- Smailys A, Tarasevicius S, Kesteris U, Kalesinskas R J, Wingstrand H. Exeter total hip arthroplasty with matte or polished stems. Medicina (Kaunas) 2007; 43(3)215–20
- Struik L C E. Physical aging in amorphous polymers and other materials. Elsevier Scientific Publishing Company, Amsterdam 1978
- Thanner J, Freij-Larsson C, Kärrholm J, Malchau H, Wesslen B. Evaluation of Boneloc. Chemical and mechanical properties, and a randomized clinical study of 30 total hip arthrolasties. Acta Orthop Scand 1995; 66(3)207–14
- Unemori M, Matsuya Y, Matsuya S, Akashi A, Akamine A. Water absorption of poly(methyl methacrylate) containing 4-methacryloxyethyl trimellitic anhydride. Biomaterials 2003; 24(8)1381–7