Abstract
Background and purpose Efforts to prevent infection of arthroplasties, including the use of antibiotic-loaded bone cement, are not always successful. We investigated whether the incorporation of chitosan in gentamicin-loaded bone cement increases antibiotic release, and prevents bacterial adherence and biofilm formation by clinical isolates of Staphylococcus spp. In addition, we performed mechanical and degradation tests.
Methods Different amounts of chitosan were added to the powder of the gentamicin-loaded bone cement. Gentamicin release was determined using high-per-formance liquid chromatography mass spectrometry. Bacterial adherence and bacterial biofilm formation were determined using clinical isolates cultured from implants retrieved at revision hip surgery. The mechanical properties were determined as a function of degradation in accordance with ISO and ASTM standards for PMMA bone cement.
Results The addition of chitosan to bone cement loaded with gentamicin reduced gentamicin release and did not increase the efficacy of the bone cement in preventing bacterial colonization and biofilm formation. Moreover, the mechanical performance of cement containing chitosan was reduced after 28 days of degradation. The compressive and bending strengths were not in compliance with the minimum ISO and ASTM requirements.
Interpretation Clinically, incorporation of chitosan into gentamicin-loaded bone cement for use in joint replacement surgery has no antimicrobial benefit and the detrimental effect on mechanical properties may have an adverse effect on the longevity of the prosthetic joint.
Gentamicin-loaded bone cement is normally used for prosthesis fixation at the time of revision surgery, in an effort to remove bacterial infection and reduce the risk of infection of the newly implanted prosthesis (Passuti and Gouin Citation2003, Hendriks et al. Citation2004). Increasingly, gentamicin-loaded bone cement is also being used for prosthesis fixation during primary surgery in an attempt to prevent implant infection. However, the isolation of gentamicin-resistant bacteria from infected prosthetic hip joints has raised concerns about the habitual use of gentami-cin-loaded bone cement (Tunney et al. Citation1998b, Hope et al. Citation1989, Thornes et al. Citation2002). Furthermore, we have shown that the bacteria that cause these infections can form bacterial biofilms on the surface of gentamicin-loaded bone cements despite the release of gentamicin (Tunney et al. Citation2007).
There is thus a clear need for the development of alternative antimicrobial strategies for the prevention and treatment of prosthetic joint infections. In recent years, functional biomaterials research has been directed towards the development of new, improved drug delivery systems. For example, addition of fillers such as dextran (Kuechle et al. Citation1991), glycine (McLaren et al. Citation2004), xylitol (McLaren et al. Citation2006), and sucrose (McLaren et al. Citation2007) to polymethylmethacrylate (PMMA) bone cement has been shown to enhance porosity and improve the elution of antibiotics from antibiotic-loaded bone cement. Considerable attention has been paid to chitosan-based materials (Khor and Lim Citation2003). Chitosan is a co-polymer of glucosamine and Nacetylglucosamine deacetylated from the natural polymer chitin, and in its crystalline form it is nontoxic, biodegradable, and biocompatible. These properties have resulted in chitosan been used for tissue engineering scaffolds, cell microcapsules, and as a drug carrier for antibiotics (Khor and Lim Citation2003, Ge et al. Citation2004). Furthermore, chitosan has been shown to have intrinsic antibacterial activity when added to acrylic bone cement. Thus, it is quite possible that chitosan added to bone cement could act as a drug carrier for gentamicin, thereby increasing gentamicin release and preventing bacterial colonization and biofilm formation. One concern, however, is that incorporation of chitosan into the bone cement may result in a reduction in the mechanical performance of the cement due to its degradation. Accordingly, in this in vitro study we incorporated chitosan into unloaded and gen-tamicin-loaded bone cement and determined the effect this addition would have on bacterial biofilm formation, gentamicin release, mechanical properties, and degradability of the bone cement.
Material and methods
Material
The bone cements used were unloaded Palacos R containing no gentamicin and Palacos R+G, which included 0.5 g gentamicin per 40-g polymethylmethacrylate (PMMA) powder cement sachet (Heraeus-Kulzer, Germany). Chitosan (Primex Ingredients ASA, Norway) was refined twice by dissolving it in dilute acetic acid solution. The solution was filtered and the chitosan was precipitated with aqueous NaOH and then dried in a vacuum oven for 24±0.5 h at 40±0.5°C. The extent of deacetylation of the chitosan powder was approximately 85%. The molecular weight was 2,000–3,000 g/mol, according to gel permeation chromatography.
Preparation of bone cements
Different amounts (1, 3, and 5% w/w) of chitosan were added to the polymer powder (40 g) of the unloaded and gentamicin-loaded Palacos R bone cement. The chitosan was uniformly dispersed within the cement powder using a small-scale turbo blender (Speed Mixer Model PAC 150FVZ; Rondol Technology Ltd., Staffordshire, UK) at a speed of 1500±50 rpm for 30±1 s. After the chitosan was blended into the cement powder, both the polymer powder and the liquid monomer were refrigerated for 24±0.5 h at a temperature of 4±0.5°C. Following refrigeration, the bone cements were vacuum-mixed under ambient conditions using a commercially available vacuum system (Summit Medical Ltd., Gloucestershire, UK), under an evacuation pressure of 72 kPa, according to the manufacturer's instructions. Bone cement specimens for determination of gentamicin release and biofilm formation were prepared by injecting the cement dough onto a glass plate (200×200±1 mm) and placing a second glass plate of similar dimensions on top of the cement. To ensure a cement thickness of 0.5±0.05 mm, spacer bars were used to separate the glass plates. Following a minimum cure of 24 h, the cement specimen was cut into 1.0×1.0±0.1 cm2 sections and stored in the dark under sterile conditions at 22±0.5°C. Bone cement samples used for mechanical testing were prepared by injecting the mixed bone cement into PTFE molds, which were allowed to cure for a minimum of 24 h. The samples were then removed from the mold. Rough specimen edges were removed by sanding with 1,200-µm grit silicon carbide abrasive. The cement samples used for measuring the compressive properties were cylinders of 12±0.1 mm length and 6±0.1 mm diameter. The samples for determining the bending properties were 75±0.5 mm in length, 10±0.1 mm in width, and 3.3±0.1 mm in thickness. Unloaded Palacos R and Palacos R+G, which contained no chitosan, were used as controls in all experiments.
Cumulative gentamicin release rates
Cut sections were placed in 5 mL of sterile water and stored in the dark at 37±0.5°C. At designated time intervals (6, 24, 48, and 72 h), each section was removed, a 1-mL sample of the water was taken and stored at –20°C until analyzed, and the section was placed in 5 mL of fresh sterile water until the next time point. All samples were analyzed for gentamicin by high-performance liquid chromatography mass spectrometry (Waters 2795 Alliance HT LC system; Waters Ltd., Elstree, Hertfordshire, UK), with gentamicin release expressed relative to the surface area of the cement section (Keevil et al. Citation2003). For each cement, 12 cut sections were used (i.e. 3 cement sections at each of the 4 designated time intervals of 6, 24, 48, and 72 h).
Bacterial strains and biofilm formation
The 3 strains used were clinical isolates cultured as described previously from implants retrieved at revision of infected hip arthroplasty surgery (Tunney et al. Citation1998a). The isolates chosen were S. epidermidis (BK2), S. aureus (LFO2), and S. capitis (ED2D), which are typical of the bacterial species that have been implicated most commonly in TJR infections. The minimum inhibitory concentrations of gentamicin for these isolates growing planktonically have been determined to be<0.125 µg/mL (BK2 and ED2D) and 4 µg/mL (LFO2) (Espehaug et al. Citation1997). Biofilms were formed for each strain (in triplicate) on unloaded, gentami-cin-loaded, chitosan-loaded, and gentamicin/chi-tosan-loaded bone cement sections over specific time intervals (6, 24, 48, and 72 h) as described previously (Tunney et al. Citation2007, Dunne et al. Citation2007). The inoculum for each strain was prepared to an optical density at 550 nm corresponding to ∼1×107 colony forming units/mL, representative of an overt infection. At each time point, the bone cement sections were removed, washed, and bio-film formation quantified from total viable counts. The unloaded Palacos R cement containing 1–5% w/w chitosan specimens were included to assess the intrinsic antibacterial activity of the chitosan.
Physical, degradability and mechanical properties
The particle size and distribution of the chitosan, gentamicin, and unloaded (Palacos R) and genta-micin-loaded (Palacos R+G) powders were examined using a laser diffraction system (Sympatec GmbH, Clausthal-Zellerfeld, Germany).
To quantify the degradability of each sample, the water absorbency, weight loss, porosity, and mechanical properties were examined during a period of degradation.
To simulate the degradation process, the bone cement specimens were placed in an incubator at 37±0.5°C for 1, 7, 14, 21, and 28 days, with phosphate buffer solution (pH=7.4) containing 0.5 mg/mL lysozyme (Sigma-Aldrich, UK), which was not replaced.
The water absorbency (Equation 1), weight loss (Equation 2) and porosity (Equation 3) during the degradation period were determined using the following relationships:
where Wwis the weight of the water absorbed by the specimen, Wd is the weight of the dried specimen after degradation, Wi is the initial weight of the specimen, ρmax is the density of the original specimen, and ρmin is the density of the dried specimen after degradation.
The compressive and bending properties were determined in accordance with ISO and ASTM standards using a universal materials testing system (EZL 6000R, Lloyd Instruments Ltd., Fareham, UK). All compression tests were conducted at a crosshead speed of 20 mm/min and the bending tests at 5.0 mm/min, respectively (ASTM F 451 1999, ISO 5833 2002). The compressive strength was determined based on the ultimate load recorded at specimen failure. The bending strength and bending modulus were calculated based on the ultimate load and the difference between the deflections under the loads of 15 N and 50 N (ASTM F 451 1999, ISO 5833 2002). Altogether, 18 specimens of each bone cement mix were used for the determination of each property.
Micrographic analysis was conducted on a selection of the bending samples from each bone cement mix using a scanning electron microscope (JEOL JSM 6500F; JEOL (UK) Ltd., Herts, UK).
Statistics
Data collated for all experimental tests were evaluated for statistical significance using a one-way analysis of variance with a p-value of<0.05 denoting significance. Post hoc tests were conducted using the Student-Newman-Keuls and Duncan methods. All the tests were conducted using commercially available software (InStat 3.06; Graph-Pad Software, La Jolla, CA).
Results
Cumulative gentamicin release rates ()
For all the bone cements tested, gentamicin release was most rapid during the first 6 h and then continued at a much lower rate. The incorporation of 1–5% w/w chitosan in the bone cement resulted in a significant decrease in the total amount of gentamicin released over 72 h (p<0.001). After 72 h, the cumulative gentamicin release for Palacos R + G and Palacos R+G loaded with 1%, 3%, and 5% Chitosan was 18.0 (SD 0.05), 10.7 (0.8), 10.3 (4.5), and 12.5 (1.5) µg/cm2, respectively.
Figure 1. Mean cumulative gentamicin release (SD) of gen-tamicin-loaded Palacos R (Palacos R+G) bone cement containing different levels of chitosan. a indicates a p-value of less than 0.001 and b indicates a p-value of less than 0.05, demonstrating a statistically significant difference between Palacos R+G and Palacos R+G bone cement containing chitosan.
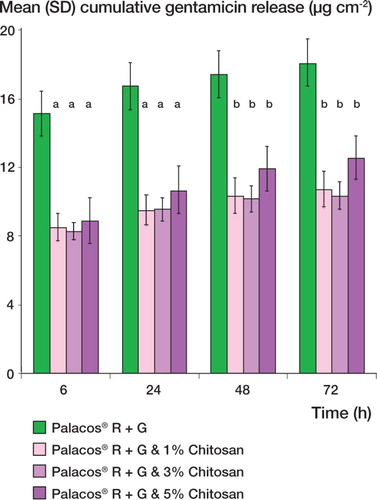
Bacterial biofilm formation ()
Incorporation of 1–5% w/w chitosan into unloaded Palacos R cement had no effect on bacterial colonization and biofilm formation (p>0.05), with bio-films being formed in similar numbers on unloaded and chitosan-loaded cement by the 3 strains tested at each time point. Furthermore, the addition of 1– 5% w/w chitosan to gentamicin-loaded (Palacos R + G) cement had an adverse effect on the efficacy of the bone cement in preventing bacterial colonization and biofilm formation. This adverse effect— only apparent at 6 h and for S. epidermidis and S. capitis strains—was shown by the fact that these strains adhered to the gentamicin-loaded cement containing chitosan but did not adhere to the gen-tamicin-loaded (Palacos R+G) cement without chitosan. For S. aureus, using gentamicin-loaded (Palacos R+G) cement did not prevent bacterial colonization and biofilm formation at any of the time points tested. Moreover, the negative effect of incorporating chitosan to gentamicin-loaded (Palacos R+G) cement was again apparent, demonstrated by the adherence of the S. aureus strain in greater or similar numbers to the gentamicin-loaded cement containing chitosan compared to the cement containing gentamicin only at all time points tested.
Figure 2. Mean (SD) colonization of unloaded (Palacos R) and gentamicin-loaded bone cement (Palacos R+G) containing different levels of chitosan, for 3 clinical prosthetic hip isolates. a indicates a p-value of less than 0.05, demonstrating a statistically significant difference between Palacos R+G and Palacos R + G bone cement containing chitosan less than 0.05, demonstrating a statistically significant difference between Palacos R+G and Palacos R+G bone cement containing chitosan.
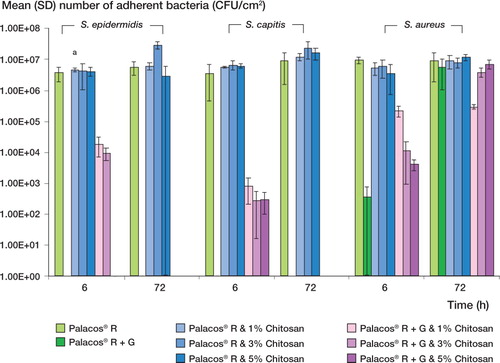
Physical, degradability and mechanical properties ( and )
The chitosan particles were angular in shape and had a significantly larger mean particle size and particle distribution (p<0.01) than the gentamicin and cement polymer powders, which where spherical in shape ().
Figure 3. The microstructure of as-molded and fractured surfaces of gentamicin-loaded (Palacos R+G) bone cement with 5% chitosan before and after 28 days of degradation. Note: arrows.
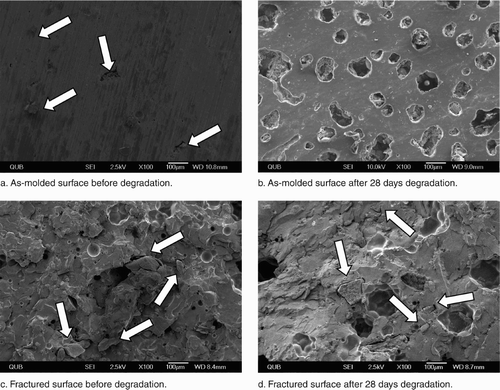
After a degradation period of 28 days, significant differences in the degradation profiles were observed between the control and the cements containing chitosan (p<0.001) (). In addition, significant differences in water absorbency, weight loss, and porosity were found not only between the control cements and each of the gentamicin-loaded (Palacos R+G) bone cements containing chitosan, but also between the gentamicin-loaded (Palacos R+G) bone cements containing chitosan (p<0.001).
Prior to degradation, no significant differences were observed in compressive strength (p=0.4) and bending strength (p=0.07) when comparing the control cements and the gentamicin-loaded (Palacos R+G) bone cements containing chitosan (). In contrast, a significant increase in bending modulus was seen when the control cements and the gentamicin-loaded (Palacos R+G) bone cements containing 3–5% w/w chitosan were compared (p<0.05).
Table 1. Mean (SD) particle size and particle distribution for chitosan, gentamicin, and unloaded (Palacos R) and gentamicin-loaded (Palacos R + G) bone cement powders
After the degradation period of 28 days, the compressive and bending strengths of the unloaded Palacos R cement and the gentamicin-loaded (Palacos R+G) cement were not significantly altered (p = 0.07 and p=0.08, respectively). In contrast, after the degradation period of 28 days, the compressive and bending strengths of the gentamicin-loaded (Palacos R+G) bone cements containing chitosan were significantly reduced (p=0.003). Furthermore, there was a significant reduction in bending modulus after 28 days of degradation (p=0.003).
Prior to degradation, SEM observations of the as-molded cement surfaces showed chitosan particles on the gentamicin-loaded (Palacos R+G) which increased as the level of chitosan increased. bone cement (, arrows), the frequency of After a degradation period of 28 days, the morphology of the bone cement changed significantly with pores forming on the surface. The pore sizes ranged from 30 to 100 µm when 5% chitosan was added to gentamicin-loaded (Palacos R+G) bone cement (. The control cements without chitosan did not show any changes in morphology over the 28-day degradation period. Panels c and d of show SEM images of the fractured surface of gentamicin-loaded (Palacos R+G) cement containing 5% chitosan, prior to and after 28 days of degradation, respectively. There was evidence of chitosan on the surface of the cement prior to degradation (. Following 28 days of degradation, the cement containing chitosan developed a highly porous structure ( whereas the gentamicin-loaded (Palacos R+G) bone cement did not show any such changes in microstructure.
Table 2. Mean changes (SD) in bone cements during the degradation period for unloaded (Palacos R) and gentamicin-loaded (Palacos R + G) bone cements containing different levels of chitosan
Table 3. Mean mechanical properties (SD) of the bone cements as a function of degradation for unloaded (Palacos R) and gentamicin-loaded (Palacos R + G) bone cements containing chitosan
Discussion
Chitosan has previously been reported to possess intrinsic antibacterial activity. For example, it has been shown that chitosan can reduce the infection rate in experimentally induced S. aureus osteomyelitis in rabbits (Risbud and Bhonde Citation2000). Furthermore, when added to hydroxyapatite and plaster of Paris to obtain a composite for sustained vancomycin or fosfomycn release, the composite material was found to be capable of inhibiting S. aureus in vitro for as long as 3 months (Burtanapanitkit et al. Citation2004). As chitosan is also used as a carrier for drug delivery, we hypothesized that chitosan added to bone cement could act as a drug-delivery carrier for gentamicin. Moreover, although the exact mechanism by which antibiotics are released from PMMA cement is unknown, it has been suggested that surface elution and diffusion are the main mechanisms of antibiotic release from the loaded material (Sterling et al. Citation2003, Bertazzoni et al. Citation2004). Thus, if the porosity of cement could be increased as a result of chitosan degradation giving a more efficacious route of antibiotic release, the degree of gentamicin release would be enhanced—thereby preventing bacterial colonization and biofilm formation on the surface of bone cement.
The results of our study, however, clearly show that the addition of chitosan to bone cement has a detrimental effect on gentamicin release; this was significantly reduced as a result of the incorporation of chitosan. The reduction in gentamicin release from chitosan-loaded cement could possibly be a result of the fact that the particle size of chitosan was significantly greater than that of gentamicin. Thus, chitosan retained on the surface of the cement was probably impeding the release of the smaller gentamicin particles from the cement. McLaren et al. (Citation2007) presented similar conclusions in a recent study investigating the influence of particle size of soluble filler (32% volume fraction) added to PMMA and its effects on permeability and elution rates. They concluded that smaller size of particles results in the development of smaller pores, greater pore interconnectivity, and a greater increase in effective surface area, resulting in a higher elution rate. Furthermore, it is possible that the chitosan does not degrade sufficiently within the first 72 h to facilitate the release of the high local gentamicin concentrations required to eliminate existing infection and prevent infection of the newly implanted device. Significantly, when overt infection was simulated using clinical isolates of Staphylococcus spp., this decrease in gentamicin release had an adverse effect on the ability of the bone cement to prevent bacterial adherence and biofilm formation.
Furthermore, the addition of 1–5% (w/w) chitosan to unloaded Palacos R bone cement did not prevent or reduce bacterial adherence and biofilm formation on the surface of the bone cement. One possible explanation for this lack of effect is that the porous microstructure that develops through the degradation of a bioactive material such as chitosan may provide additional niches for the attachment of bacteria, which subsequently form a bacterial biofilm. Interestingly, our results contrast with the results of a previous paper which reported that the incorporation of chitosan and chitosan nanoparticles resulted in a 1–2 log10 reduction in S. aureus and S. epidermidis adherence to bone cement that was not loaded with gentamicin (Shi et al. Citation2006). However, there a number of differences between the two studies that may account for the contrasting results. For example, Shi et al. incorporated chitosan and chitosan nanoparticles at a bone cement: Chitosan powder ratio of 15% (w/w), which is much higher than the 1–5% (w/w) ratio used in the present study. Furthermore, these authors used an attachment time of only 3 h, which would be sufficient to allow adherence but not formation of a bacterial biofilm. In contrast, we used a minimum attachment time of 6 h and examined biofilm formation at a number of additional time points, thereby simulating in vivo conditions more closely. All 3 strains of Staphylococcus spp. used in the present study were clinical isolates cultured from retrieved prosthetic hip implants, and were known to be biofilm formers. This is in contrast to the work of Shi et al. (Citation2006), who used S. epidermidis isolate ATCC 12228. This strain has been categorically shown to be incapable of forming biofilms in numerous previous studies (Garcia et al. 2000, Arciola et al. Citation2004, Citation2005) and it would, therefore, not be suitable for use in bacterial adherence assays.
During the degradation period, the porosity of the gentamicin-loaded (Palacos R+G) cement increased through the incorporation of chitosan. These results support the findings previously reported by Kim et al. (Citation2004), who showed that the porosity of PMMA cement containing bioactive materials increased by approximately 500% after a degradation period of 8 weeks (Kim et al. Citation2004). Ensing et al. (Citation2005) also observed a significant change in pore structure for bone cements containing gentamicin-loaded PMMA beads when immersed in PBS for 18 h, with pores in the range of 0.1 to 0.01 µm forming as the gentamicin-loaded PMMA beads in the cement dissolved. Our SEM results revealed that chitosan existed on the as-molded surface of chitosan loaded Palacos R+G specimens as the number and sizes of the pores significantly increased during the degradation period. We did not find this on the as-molded surfaces of the control cements.
The mechanical properties of the PMMA cement used in orthopedic surgery play an important role in determining the successful long-term stability of the prosthesis. In the compression and bending tests conducted prior to degradation, there was no significant difference in the compressive and bending strengths of the control cement and the gentamicin-loaded bone cements containing chitosan. However, the cement with 3–5% (w/w) chitosan had a significantly higher bending modulus (p<0.05). Furthermore, for all cements tested, the mechanical properties measured were in excess of the minimum values stipulated by the ISO and ASTM standards for acrylic bone cement (ASTM F 451 1999, ISO 5833 2002). The unloaded Palacos R and gentamicin-loaded (Palacos R+G) cements did not show any changes in compressive or bending strength after degradation. There was, however, a significant reduction in bending modulus for the unloaded (Palacos R) and gentamicin-loaded (Palacos R+G) cements after 28 days of degradation (p<0.05). This finding is consistent with the results of a previous study in which the authors postulated that the reduction in elastic modulus of unloaded (Palacos R) and gentamicin-loaded (Palacos R+G) cements was due to the plasticising effect of the aqueous medium (Dunne et al. Citation2006). In terms of compliance with the minimum ISO requirements, the gentamicin-loaded Palacos R cements containing chitosan powder did not maintain acceptable ISO or ASTM levels of compressive strength (70 MPa) or bending strength (50 MPa) on a repeatable basis after a degradation period of 7 days. The gentamicin loaded (Palacos R+G) cement with 5% chitosan did not retain a bending modulus in excess of the minimum requirement as quoted by the ISO standard (1,800 MPa) after a degradation period of 14 days. These results clearly indicate that the addition of chitosan to bone cement has an adverse effect on the mechanical properties, and could be detrimental to the longevity of the prosthetic joint.
The bone cement used in this study was prepared under vacuum, in accordance with current surgical practice. Vacuum mixing of antibiotic-loaded bone cements improves its mechanical properties by reducing the porosity (Kuechle et al. Citation1991, Klekamp et al. Citation1999), and while it has been shown to reduce the rate of cement failure under fatigue loading by up to tenfold (Klekamp et al. Citation1999) and to reduce the apparent porosity on radiographs by up to fivefold (Askew et al. Citation1990), it has also been shown to reduce antibiotic elution rates (Kuechle et al. Citation1991). Thus, it may be possible that when chitosan-based antibiotic-loaded bone cements are mixed under normal atmospheric pressure, they exhibit different pharmokinetics because of the increase in surface area and porosity.
To summarize, the findings of our in vitro study suggest that the incorporation of chitosan in unloaded Palacos R cement at concentrations of 1–5% (w/w) does not prevent bacterial colonization and biofilm formation by clinical prosthetic hip isolates. Similarly, the addition of chitosan powder to Palacos R+G cement significantly reduced gentamicin release and did not increase the efficacy of the cement in preventing bacterial colonization and biofilm formation. Furthermore, the mechanical performance of the bone cement containing chitosan was reduced after 28 days of degradation, with the compressive and bending strengths of the cement not being in compliance with the minimum requirements stipulated by the ISO or ASTM standards for PMMA bone cement. Thus, incorporation of chitosan into Palacos R+G bone cement for use in TJR surgery has no clinical antimicrobial benefit and the detrimental effect on mechanical properties may adversely affect the longevity of the prosthetic joint.
AB and JH were funded by a European Social Fund Grant and by the Department of Education and Learning (Northern Ireland), respectively. The supply of bacterial isolates by Shelia Patrick, School of Medicine and Dentistry, Queen's University Belfast, UK is gratefully acknowledged.
No competing interests declared.
ND, MT: joint principal investigators, performing data analysis and preparation of the manuscript. FB: data analysis. JH and CN: testing of physical, degradability, and mechanical properties. AB: measurement of gentamicin release and degree of biofilm formation. GW: production of chitosan.
References
- Arciola C, Campoccia D, Gamberini S, Rizzi S, Donati M, Baldassarri L, Montanaro L. Search for the insertion element IS256 within ica locus of Staphylococcus epidermidis clinical isolates collected from biomaterial-associated infections. Biomaterials. 2004; 25(18)4117–25
- Arciola C, Gamberini S, Campoccia D, Visai L, Speziale P, Baldassarri L, Montanaro L. A multiplex PCR method for the detection of all five individual genes of ica locus in Staphylococcus epidermidis. A survey of 400 clinical isolates from prosthesis-associated infections. J Biomed Mat Res A 2005; 75(2)408–13
- Askew M, Kufel M, Fleissner P, Grasdisar I, Salstrom S, Tan S. Effect of vacuum mixing on the mechanical properties of antibiotic-impregnated polymethyl methacrylate bone cement. J Biomed Mat Res 1990; 24(1)73–580
- ASTM F 451-99ae1: Standard Specification for Acrylic Bone Cement 1999
- Bertazzoni Minelli E, Benini A, Magnan B, Bartolozzi P. Release of gentamicin and vancomycin from temporary human hip spacers in two-stage revision of infected arthroplasty. J Antimicrob Chemother 2004; 53(1)329–34
- Burtanapanitkit B, Srinilta V, Ingiviga N, Oungbho K, Geater A, Ovatlarnporn C. The efficacy of hydroxyapatite composite as a biodegradable antibiotic delivery system. Clin Orthop. 2004; 424: 244–52
- Dunne N, Leonard D, Daly C, Buchanan F, Orr J. Small punch testing technique to characterise the mechanical properties of self-curing acrylic bone cement. Proceedings of the Institution of Mechanical Engineers. Journal of Engineering in Medicine Part H6 2006; 220(1)11–21
- Dunne N, Hill J, McAfee P, Todd K, Kirkpatrick R, Tunney M, Patrick S. In vitro study of the efficacy of acrylic bone cement loaded with supplementary amounts of gentamicin. Effect on mechanical properties, antibiotic release, and biofilm formation. Acta Orthop 2007; 78(6)774–85
- Ensing G, Hendriks J, Jongsma J, vanHorn J, van der Mei H, Busscher H. The influence of ultrasound on the release of gentamicin from antibiotic-loaded acrylic beads and bone cements. J Biomed Mat Res B 2005; 75(1)1–5
- Espehaug B, Engesaeter L, Vollset S, Havelin L, Langeland N. Antibiotic prophylaxis in total hip arthroplasty. Review of 10905 primary cemented total hip replacements reported to the Norwegian arthroplasty register 1987 to 1995. J Bone Joint Surg (Br) 1997; 79: 590–5
- Garcia-Saenaz M, Arias-Puente A, Fresnadillo-Martinez M, Matillia-Rodriguez A. In vitro adhesion of Staphylococcus epidermidis to intraocular lenses. J Cataract Refract Surg 2000; 26(11)1673–9
- Ge Z, Baguenard L, Wee A, Khor E. Hydroxyapatite-chitin materials as potential tissue engineered bone substitutes. Biomaterials 2004; 25(6)1049–58
- Hendriks J, van Horn H, van der Mei H, Busscher H. Backgrounds of antibiotic-loaded bone cement and prosthetic related infection. Biomaterials 2004; 25(1)545–56
- Hope P, Kristinsson K, Norman P, Elson R. Deep infection of cemented total hip arthroplasty caused by coagulase-negative staphylococci. J Bone Jt Surg (Br) 1989; 71: 851–5
- International Standard Organisation 5833: Implants for surgery. Acrylic resin cement 2002
- Keevil B, Lockhart S, Cooper D. Determination of tobramycin in serum using liquid chromatography–tandem mass spectrometry and comparison with a fluorescence polarisation assay. J Chromatography B 2003; 794: 329–35
- Khor E, Lim L. Implantable applications of chitin and chitosan. Biomaterials 2003; 24(1)2339–49
- Kim S, Kim Y, Yoon T, Park S, Cho I, Kim E, Kim I, Shin J. The characteristics of a hydroxyapatite-chitosan-PMMA bone cement. Biomaterials 2004; 25(1)5715–23
- Klekamp J, Dawson J, Haas D, DeBoer D, Christie M. The use of vancomycin and tobramycin in acrylic bone cement: biomechanical effects and elution kinetics for use in joint arthroplasty. J Arthroplasty 1999; 14(1)339–46
- Kuechle D, Landon G, Musher D, Noble P. Elution of vancomycin, daptomycin and amikacin from acrylic bone cement. Clin Orthop 1991; 264: 302–8
- McLaren A, Nelson C, McLaren S, DeClerk G. The effect of glycine filler on the elution rate of gentamicin from acrylic bone cement: A pilot study. Clin Orthop 2004; 427: 25–7
- McLaren A, Nelson C, McLaren S, Smeltzer M. Xylitol and glycine fillers increase permeability of PMMA to enhance elution of daptomycin. Clin Orthop 2006; 451(1)22–8
- McLaren A, McLaren S, McLemore R, Vernon B. Particle Size of fillers affects permeability of polymethylmethacrylate. Clin Orthop 2007; 461: 64–7
- Passuti N, Gouin F. Antibiotic-loaded bone cement in orthopaedic surgery. J Bone Spine 2003; 70(1)169–74
- Risbud M, Bhonde R. Polyacrylamide-chitosan hydrogels: in vitro biocompatibility and sustained antibiotic release studies. Drug Deliv 2000; 1(1)61–l7
- Shi Z, Neoh K, Kang E, Wang W. Antibacterial and mechanical properties of bone cement impregnated with chitosan nanoparticles. Biomaterials 2006; 27(1)2440–9
- Sterling G J, Crawford S, Potter J H, Koerbin G, Crawford R. The pharmacokinetics of simplex-tobramycin bone cement. J Bone Joint Surg (Br) 2003; 85: 646–9
- Thornes B, Murray P, Bouchier-Hayes D. Development of resistant strains of Staphylococcus epidermidis on genta-micin-loaded bone cement in vivo. J Bone Joint Surg (Br) 2002; 84(5)758–60
- Tunney M, Patrick S, Gorman S, Nixon J, Anderson N, Davis R, Hanna D, Ramage G. Improved detection of infection in hip replacements. A currently underestimated problem. J Bone Joint Surg (Br) 1998a; 80 568–72
- Tunney M, Ramage G, Patrick S, Murphy P, Gorman S. Antimicrobial susceptibility of bacteria isolated from orthopaedic implants following revision hip surgery. Antimicrob Agents Chemother. 1998b; 42(11)3002–5
- Tunney M, Dunne N, Einarsson G, McDowell A, Kerr A, Patrick S. Bacterial biofilm formation by bacteria isolated from retrieved failed prosthetic hip implants in an in vitro model of hip arthroplasty antibiotic prophylaxis. J Orthop Res 2007; 25(10)2–10