Abstract
Man's evolution features two unique developments: growing a huge brain and upright gait. Their combination makes the pelvis the most defining skeletal element to read human evolution. Recent revival in joint preserving hip surgery have brought to attention morphological variations of the human hip that appear similar to hips of extant mammals. In man, such variations can produce hip osteoarthrosis through motion. We reviewed the evolution of the hip and pelvis with special interest in morphology that can lead to motion induced osteoarthrosis in man. The combination of giving birth to big brained babies and walking upright has produced marked differences between the sexes in pelvis and hip morphology, each having their characteristic mode of hip impingement and osteoarthrosis.
Glossary
Definitions of position as in a human pelvis
abduction: limb moves away from midline (laterally)
adduction: limb moves towards the midline (medially)
medial/internal (endo) rotation: foot/greater trochanter turns inward
lateral/external (exo) rotation: foot/greater trochanter turns outward
flexion: bending, lower limb moves ventrally
extension: lower limb moves dorsally
anterior/ventral: to the front or belly-side
posterior/dorsal: to the back or back-side
medially: near or towards the midline of the body
laterally: near the outside of the body, away from midline
cranial: near or towards the head
caudal: near or towards the tail
ventral: near or towards the belly-side (front) of the body
dorsal: near or towards the backside of the body
abductors: hip muscles (gluteus medius and minimus and tensor fascia latae) that move the lower limb in a lateral direction (away from the midline) and thus can also stabilize the pelvis over the femoral head when standing on one leg.
Acanthostega: Acanthostega was clearly a tetrapod, but a poor version for a land animal. Its legs were frail to support its weight, wrists were absent. Yet, it had digits (8! fingers and 7! toes). However, it also had internal gills—like fish. Perhaps Acanthostega never left the water, hence one of the fishapods, animals that combined tetrapod and fishlike characteristics in the period of approx. 380–340 mya.
acetabular fossa of fovea: central part of the acetabulum, not covered with cartilage, where the ligamentum teres originates. (see lateral (side) view of pelvis).
acetabular notch: non-articular area making up the inferior circumference of the acetabulum between the two horns of the lunate surface.
acetabulum: the deepening or socket in the pelvis that holds the proximal (upper) end of the femur (thigh bone), in mammals the femoral head.
adductors: muscles that move the femur towards the body axis in the coronal plane (Man).
allometry: relatively increased growth, i.e. increasing more than proportionally with increasing body weight.
amniote eggs: eggs with a membrane that prevent them from drying out when not in water.
amphibians: (class Amphibia), e.g. frogs, toads and salamanders, are cold-blooded animals that transform (metamorphose) from a juvenile, water-breathing form to an adult, air-breathing form. Usually, amphibians have four limbs. Unlike other land animals (amniotes), amphibians lay eggs in water, as their fish ancestors did.
anterior gluteals: gluteus minimus and medius muscles in primates (quadrupeds).
anteversion: forward tilt,
femoral anteversion or the angle between the neck of the femur and the back of the two femoral condyles (dashed line).
An anteversion angle of 0 degrees indicates the axis of the neck and the condylar axis are in the same plane. A negative angle, called retroversion, means the head of the femur is rotated posterior to the condylar axis. In normal growth and development the human anteversion angle is about 30 degrees at birth and decreases to 15 degrees by skeletal maturity. A large anteversion angle or a retroversion angle creates abnormal hip motion, increases the potential for dislocation of the hip, and may contribute to the development of osteoarthritis in the hip.
acetabular anteversion: forward tilt of acetabulum in pelvis (angle between thin dash lines).
anthropoidea: monkeys and apes
A-P axis: see Pelvic positions
aspherical: out of round
Australopithecines: the most likely ancestors of the genus for Homo, of approx. 4–2.5 mya.
bipedal: on two feet
bipedal gait: upright walking
body planes: see figure
Bonobo: Pan paniscus (pygmy chimpanzee), close relative of the chimpanzee, but separate species since approx. 2 my.
Carboniferous: 360–300 mya, a geologic period of glaciation, low sea level and mountain building.
carnivore: meat eater.
caudally: in the direction of the tail
CE (centre-edge) angle: Angle between vertical line through centre of femoral head and line from centre of femoral head to edge of the acetabulum.
chimpanzee: Pan troglodytes.
Coelacanth: (Latimeria chalumnae) order of fish related to lungfishes and tetrapods, first appearing in the fossil record in the Devonian, 410 mya, believed extinct since the end of the Cretaceous (65 mya), but found off the east coast of South Africa in 1938, near the Chalumna River. Now also known in Madagascar, the Comoros, and East African coast. The second extant species, L. menadoensis, was described from Sulawesi, Indonesia in 1999. A coelacanth can be up to 2 m and 80 kgs, usually living at depths of 75–200 m. They are opportunistic night feeders, hunting squid and other fish.
continental drift (Plate tectonics): Alfred Wegener hypothesized in 1912 that continental landmasses move about on the globe, but the Dutch cartographer Abraham Ortelius in 1596 had already considered the Americas “torn away from Africa and Europe” Only in the 1960s, sufficient geological evidence of that movement was found in the mechanism of seafloor spreading.
coronal plane: see body planes
cortical bone: the dense and hard bone at the outside of a bone. The spongeous bone with a a honeycomb like structure inside a bone is called trabecular bone.
cranially: towards the head
cranio-caudal: in the long axis of the body (from head to tail)
cranium: skull
crocodiles: large aquatic reptiles that live throughout the tropics in Africa, Asia, the Americas and Australia.
crossover sign acetabulum: when the anterior (green line) and posterior (red line) wall of the acetabulum intersect on an pelvis AP radiograph. This is one feature of acetabular retroversion, i.e. a position of the acetabulum facing backward instead of forward (anteversion). See radiographs.
cursorial: long distance walking and running
dermal bones: bone formed in the skin without a cartilaginous precursor (for example cleithrum and anocleithrum in fish)
Devonian period: 415–75 mya
DNA hybridization evidence: the ranges for the datings of divergence nodes are Old World monkeys, 25–34 mya; gibbons, 16.4–23 mya; orangutan, 12.2–17 mya; gorilla, 7.7–11 mya; chimpanzees–human, 5.5–7.7 mya (Sibley).
dorsal/dorsally: posterior, towards the back (as opposed to ventral)
encephalization: the amount of brain volume or mass an animal has exceeding that what may be expected related to the animal's body mass, i.e. brain size relative to body size.
endochondral bones: are formed from a cartilaginous precursor by endochondral ossification: see ossification.
explosive radiation: rapid emergence of new species
extant: still living today
extinct: no longer living
extension: retro or backward flexion, moving toward the back.
Eusthenopteron (Eusthenopteron foordi): Genus of fossil lobe finned fish of about 385 mya. Eusthenopteron has endochondral bones in its pectoral and pelvic fins with the pattern seen in mammals and other tetrapods today of on proximal (humerus, femur) and two distal bones (radius/ulna and tibia/fibla). This, and it's extensive documentation by paleoichthyologist Erik Jarvik has brought Eusthenopteron a somewhat iconic status as the link between fish and tetrapods. The first specimen was described in 1881 from, Miguasha, Quebec. Some 2,000 Eusthenopteron specimens have been found there.
femoral condyles: semicircular bones on in and outside (medial and lateral) of the femur, which articulate on the plateau of the tibia
femoral neck: bone between femoral head and femoral shaft
femur: thigh bone, upper leg
fishapods: mosaic animals that combined tetrapod and fishlike characteristics in the period of approx. 380–340 mya. They are sometimes called “transitional” or intermediate forms between fish and tetrapods, but neither these animals nor evolution had any idea what they were in transit to, or what they were intermediates between. Examples: Panderichtys, Acanthostega, Ventastega, and Tiktaalik.
flexion: forward movement of a limb.
fossil: the preserved remains or traces of animals, plants, and other organisms from the remote past. Fossils are usually formed when mineral-filled water penetrates the remains of a buried creature. Over the ages, minerals are laid down in the body, for which bone with its porous structure is particularly suited, changing it to a virtual “stone bone” over time. But even soft tissues have in rare cases been preserved, for example the invertebrates of half a billion years ago in the Burgess Shale in Canada.
fossil “gap”: the absence of “intermediate forms between two taxa. Given the time span and the odds for the required geologic processes, it would be highly surprising if there was no fossil gap. Estimates are that about 90% of all species, will never be known to us as fossils (Dawkins, Citation2004).
genetic switches: the first example of a genetic switch unravelled is the lactose switch (by French biologists Jacob and Monod, Nobelprize 1965). E. coli bacteria normally digests glucose, but switch to other sugars such as lactose when glucose is unavailable. Lactose is broken down by the enzyme beta-galactosidase which normally is virtually absent in E. coli that digest glucose. If, however, glucose runs out, and only lactose is present, the bacteria starts making beta-galactosidase at a nearly thousand fold rate. What happens is that a genetic switch is turned on by the presence of lactose which then starts beta-galactosidase production by the beta-galactosidase gene. The switch itself is a molecule that sits at the beta-galactosidase gene, blocking its activity. But this molecule also has a binding site for lactose, and when lactose binds, the switch falls off and the gene starts making beta-galactosidase.
geologic time scale: . The 4.55 billion-year geologic time scale is subdivided into different time episodes of varying lengths. All of Earth history is divided into two great expanses of time. The Precambrian from when Earth first formed to about 570 mya. The Phanerozoic Eon began 570 mya and continues today. The time scale is occasionally refined, for example by improved dating of rocks using radiometric methods.
gibbons: small Asian apes, fine acrobates, proficient brachiators (moving about using arm swinging). They also walk upright over branches or on the ground.
hominids: members of the family Hominidae (or “great apes”), including the extinct and extant humans, chimpanzees, gorillas, and orangutans.
hominoids: (superfamily Hominoidea) the great apes such as human, gorilla, chimpanzee, orangutan and lesser apes (gibbons). Living hominoids are human, chimpanzee, bonobo, gorilla, orangutan and nine species of gibbon.
hominins: humans and our ancestors, but not other apes.
human: Homo sapiens. The word is sometimes also used to refer to any extinct member of the genus Homo (hominins).
horizontal plane: plane parallel to the ground
hypertrophy: growth
impinge: make an impact, encroach (intrude or advance beyond due limits)
impingement bump: outcrop of bone / cartilage, in humans mostly seen at the anterosuperior (femoral) head-neck junction
independent development of a trait: means that the trait arose anew in a species that in its family tree was surrounded by species that did not have this trait.
invertebrates: animals without vertebral collum (spine) such as insects or worms.
joint preserving hip surgery: surgery that attempts to increase the lifespan of a joint, in other words that tries to delay or obviate the implantation of an artificial joint.
kinematics: the study of motion without regard to force or mass.
kinetics studies: what motions of bodies are produced under the action of particular forces.
knuckle-walking: quadrupedal walking where the front limb rests on knuckles.
K/T boundary: separation between the age of dinosaurs (Cretaceous 65–145 mya) and the age of mammals (Tertiary). Geologist can find a worldwide layer of Iridium (a rare metal in the Earth's crust, but common in meteorites) in the geologic strata at 65 mya. K is the traditional geological abbreviation for Cretaceous, the C already being in use for Carboniferous epoch (360–300 mya).
K/T mass extinction event: presumably a large meteorite impact 65 mya near the Yucatan peninsula that caused extinction of all Dinosaurs (except birds) and approx. half of all other species.
labrum: cartilage ring on the acetabular rim (periphery) that works as a fluid seal, containing the femoral head and the joint's fluid.
lateral: located on the outside
ligamentum teres: ligament between femoral head and acetabulum (often absent in orangutan)
lordosis: extension of the lumbar spine (“backward bending”)
lumbar spine: part of the vertebral collum between the ribs and the sacrum
lunate surface: the horse–shoe shaped cartilage surface within the acetabulum
lungfish: freshwater fish, retaining “primitive” characteristics such as ability to breathe air, lobed fins and a well-developed internal skeleton. Today, they live in Africa, South America, Australia.
medially: near or towards the midline of the body
medio-lateral: in a sideways direction
Menschik's kinematic laws: the knee moves in its flexion–extension guided by its cruciate ligaments according to a four bar linkage model.
molecular clock (evolutionary clock): a technique in molecular evolution to relate the time since two species diverged to the number of molecular differences measured between the species' DNA sequences or proteins. The first notion of a “molecular clock” is attributed to Emile Zuckerkandl and Linus Pauling who, in 1962, saw that the number of amino acid differences in hemoglobin between lineages scales roughly with divergence times, as seen in fossil evidence.
my: million years
mya: million years ago
neck-shaft angle (also caput-collum-diaphysis or CCD angle): angle subtended between axis of the femur and of the femoral neck.
neoteny: birth of a fetus before development/ growth has finished. In neoteny, the physiological (or somatic) development of an animal or organism is slowed or delayed
Old World monkeys: a group of primates, from the superfamily Cercopithecoidea. Old World monkeys live in Africa and Asia today, but are also known from the fossil record in Europe. The most familiar species of Old World monkeys are baboons, mandrills and macaques.
opposable hallux: a great toe that can grab as a thumb by moving opposite to the other toes
orangutan: Pongo pygmaeus
ossification: bone formation, Fetal bone formation happens by two processes: intramembranous and endochondral ossification. Intramembranous ossification mainly occurs during formation of the flat bones of the skull; the bone is formed from mesenchymal tissue. In intramembranous ossification an ossification center develops which calcifies and matures and develops a periosteum. Endochondral ossification, on the other hand, occurs in long bones such as the femur. The bone is formed from cartilage beginning in “primary ossification centers”. They mostly appear during fetal development, and form the diaphyses of long bones, short bones and some parts of irregular bones. Secondary ossification happens after birth, and forms the epiphyses of long bones and the extremities of irregular and flat bones, such as the pubic rami. The diaphysis and both epiphyses of a long bone are separated by a growthplate of cartilage (physis). When a child reaches skeletal maturity (18 to 25 years of age), all of the cartilage is replaced by bone, fusing the diaphysis and both epiphyses together (epiphyseal closure), but this is markedly delayed in the human pubic bones, allowing longer growth and greater flexibility at the pubic symphysis.
paleontology: study of the morphology of extinct animals
Pan troglodytes: chimpanzee
Pan paniscus: Bonobo
Panderichthys: a fishapod with many features of the transitional between lobe-fin fishes (e.g., Eusthenopteron) and early tetrapods. It is 90–130 cm long with a large flattened tetrapod-like head that is narrow at the snout and wide in the back.
Pangaea: supercontinent joining all landmasses during the Paleozoic and Mesozoic eras about 250 mya. The name was coined by Alfred Wegener (1920), originator of the continental drift theory.
pectoral: breast
Pelycosaurians: earliest mammal-like reptiles, living in the Carboniferous period. Best known example is Dimetrodon. Pelycosaurs had sprawling gait.
Permian: geologic period from 300–250 mya that ended, with the most extensive extinction event recorded in paleontology, probably by impact of a comet larger than in the K/T event. In the Permian-Triassic event 90% to 95% of marine species and 70% of all land organisms became extinct.
phylogeny: the study of evolutionary relatedness among groups of organisms.
Pongo pygmaeus: orangutan
point of ground contact: point where the body mass is transferred to the ground (as determined by force plates in gait analysis), i.e. position of this point in the foot changes while walking.
popliteal fossa: dorsal (flexion) side of the knee joint
postcranial (skeleton): skeleton “beyond the cranium”, i.e. everything but the skull.
Primates: apes, monkeys (e.g. baboons) and lemurs.
quadrupeds: those walking on all fours
retroversion: backward tilt of acetabulum or femoral neck. See anteversion and crossover sign
reptiles: air breathing, cold-blooded, scaly animals of the class Reptilia which includes snakes, lizards, crocodiles, turtles, tortoises.
rodents: an order of mammals characterised by two continuously-growing incisors in the upper and lower jaws which must be kept short by gnawing.
Forty percent of mammal species are rodents, and they are found in vast numbers on all continents other than Antarctica. Common rodents include mice, rats, squirrels, chipmunks, gophers, porcupines, beavers, hamsters, gerbils, guinea pigs, degus, chinchillas, and groundhogs. Rodents use their sharp incisors to gnaw wood, break into food, and bite predators. Most eat seeds or plants, though some have more varied diets. Some species have historically been pests, eating human seed stores and spreading disease.
rotational birth: rotation of the fetal head to pass the birth canal
sacro-lumbar spine: part of the spine between tail/sacrum and thorax (ribs)
sacrum: bone connecting the two pelvic bones (ilium) dorsally
sagittal: see body planes
scoliosis: S-shaped curve in the spine in the frontal plane
sexual dimorphism: systematic difference in form between individuals of different sex in the same species. Examples are size, color, but also body parts used in courtship displays or fights, such as ornamental feathers, horns, antlers or tusks.
sexual selection: Darwin proposed that certain traits can be explained by competition between members of a species. He defined sexual selection as the effects of the “struggle between the individuals of one sex, generally the males, for the possession of the other sex”. Biologists today distinguish between “male to male combat”, “mate choice” (usually females who choose) and sexual conflict. Traits selected for by male combat are called secondary sexual characteristics (e.g. horns, antlers), traits selected by mate choice are called “ornaments”.
species: see taxonomy. Perhaps 99% of all species that ever existed have gone extinct (Sepkoski).
spherical: round
spondylolisthesis: when one vertebral body shifts anteriorly (ventrally) on the lower (caudal) vertebral body due to a defect or fracture in the vertebral arch on the dorsum of the vertebrae.
sprawling gait: see postures, gait with flexed limbs perpendicular to the body axis, requiring a constant “push-up” to keep the body from the ground.
superior femoral neck: see figure
superorder: see taxonomy
taxon (plural: taxa) is a name designating an organism or a group of organisms. Carl Linnaeus, Swedish physician, botanst, zoologist, formulated a systematic hierarchy of taxa reflecting evolutionary relationships in his Systema Naturae, during his stay in the Netherlands in 1735.
taxonomy: taxis = order, the classification of animals according to similarity. See Table
tetrapods: animals with four limbs, digits, ribs, neck.
tilt or inclination: is the angle between the face of the acetabulum and the transverse plane, or the angle between the acetabular axis and the longitudinal axis. The transverse plane can be defined as a line joining the left and right spinous processes.
Tiktaalik: a Sarcopterygean found in the Canadian Arctic, lived 380 mya, see Fishapods
trait: any single feature or quantifiable measurement of an organism (a characteristic or property of an animal).
Linnean taxonomy of Man
transverse plane: the plane perpendicular to the main (longitudinal) body axis (see body planes).
transverse process: bony spine on vertebrae, attachment site for ligaments and muscles. Compared to monkeys, apes developed a much more dorsal position of the transverse process, which determines the size the erector spinae muscle can attain. In humans, this dorsal position is still present.
The transverse process in monkey and man. Drawing from Lovejoy Citation2005.
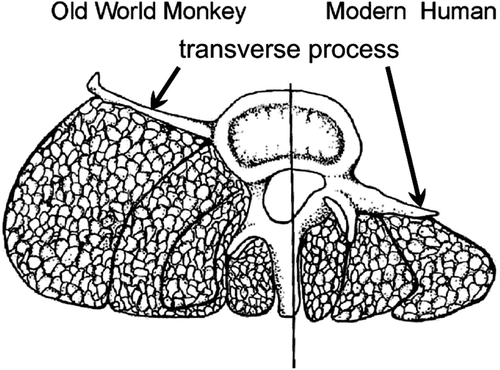
Trendelenburg gait: swaying gait where the pelvis tilts downward over the loaded hip due to weakness of the abductor mechanism.
trichromatic vision: colour vision based on the presence of three types of colour sensitive cells (“cones”): red, green and blue.
triradiate: consisting of three parts (in the acetabulum: the ilium, ischium and pubis)
ungulates: hoofed animals such as horses, cows and deer
ventral: anterior, towards the abdomen (as opposed to dorsal)
vertebral collum: spine
vertebrates: animals with a vertebral collum (spine)—fish, reptiles, amphibians, birds, and mammals.
Evolution of the hip and pelvis
Introduction
The human hip is a conceptually simple ball and socket joint, but part of a complex anatomic unit with the pelvis and sacro-lumbar spine. This unit is highly variable between different species of animals. In contrast, the knee exhibits a similar building plan in many animal species. The knees of birds, crocodiles, cows and man all share similar morphology. They have cruciate ligaments, menisci and two different radii of curvature for the tibiofemoral and patellofemoral joints (Dye Citation2003), and thus follow Menschik's kinematic laws (Müller Citation1983). Below the knee, enormous variation is seen in the morphology of paws, hoofs, feet, flippers etc. Above the knee, and perhaps surprisingly, hip morphology also differs fundamentally in various animals that share similar knees.
Morphologic variations of the hip that cause impingement (abutment, jamming or impact) between the femoral neck and acetabulum have long been associated with pain and arthritic changes (Smith-Petersen 1936, reprinted 2009). More recently, the mechanism of femoroacetabular impingement has been further clarified, and can now be summarized as osteoarthrosis through motion (Ganz et al. Citation2008, Harris Citation1986, Stulberg Citation1975). Some of the morphologic traits of human femoroacetabular impingement are normal morphology in extant mammals, for example the aspherical femoral heads of ungulates such as horses and deer. But when seen in the human hip, such morphology can induce osteoarthrosis through motion, not only in sports but also in daily activities.
The aim of this study is to present a framework of evolution of the hip and pelvis and relate this to the human hip joint with special interest for development of morphology that can lead to motion induced osteoarthrosis in man.
The pelvis is not attached to the spine in Fish
Fish have no neck—the pectoral (breast) fins are attached to the pectoral ring, which is attached to the cranium (skull) through “dermal bones” (). Near the tail, the paired pelvic fins are attached to the pelvic ring, which, however, is not attached to the spine, it is a “free” element (). Development of land life hinged on the development of these paired fins into limbs that, eventually, could bear the animal's weight against gravity. However, it now appears that limbs first developed in water, not on land.
Figure 1. Ray- versus lobe-finned fish: a lobe-finned fish (bottom) has endochondral bones with the primordial building plan of one proximal and two distal bones. The lobe-fin is narrower at its base, facilitating rotation around its long axis to increase efficiency of swimming (adapted from Clack Citation2002, with permission).
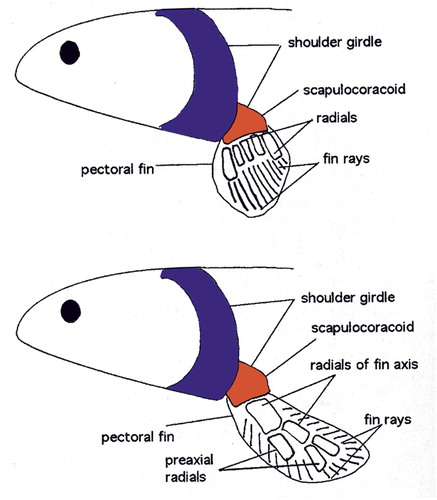
Figure 2. Eusthenopteron (top), a fossil lobe-finned fish of approx. 380 mya is seen as the beginning of the transition to land-life. The minute scapulocoracoid (red) is attached to the skull through the dermal bones cleithrum and anocleithrum (blue). The pelvic bone (yellow, green, orange) is not attached to the spine. Acanthostega (a fishapod, middle) can be seen as the other end of the transition to land-life, and the time elapsed between these two fossils is about 15 million years. Scapulocoracoid (red) has increased in size, and the pectoral girdle is no longer attached to the cranium. The pelvis has enlarged markedly and is now attached to the spine by ligaments and muscles. Varanus (bottom), is an extant tetrapod, a lizard. The clavicle (light blue) is not attached to the spine, allowing movement between the scapulocoracoid and the spine to increase stride length. The pelvis has a bony connection to the spine through the sacroiliac joints (figures not to scale, adapted from Clack Citation2002, with permission).
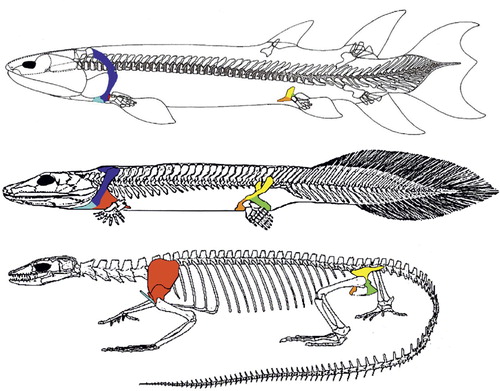
Land life started with Sarcopterygians (from Greek sarx – flesh, pteryx – fin) in the Devonian period (415–375 million years ago – mya). Sarcopterygians, or lobe-finned fish, are still around. Examples are lungfish and coelacanths. Lobe-finned fish such as the fossil Eusthenopteron (), were the first to have endochondral bones in their paired breast and pelvic fins with the pattern still seen in our limbs today: one proximal (femur and humerus) and two distal bones (tibia/fibula and radius/ulna, –). This setup narrows the base of the fin compared to that of ray-finned fish (), allowing the fin to rotate easier around its long axis, increasing efficiency of swimming. These fins are also sturdier, allowing these fish to push them along the bottom of shallow waters. Their pelvic fins had what can be interpreted as a proto-femur ().
Fishapods evolve limbs – in water
In recent years the “fossil gap” between fish and tetrapods (animals with four limbs, digits, ribs, neck) narrowed down with spectacular finds of intermediate forms dating from 380–360 mya. The most recent example is Tiktaalik, a Sarcopterygean that lived 375 mya in the Canadian Arctic (at that time situated near the equator with a subtropical climate – ). Tiktaalik looked like a fish and was a fish, for it had fins, scales, and gills (). However, it also had a neck, ribs, an upper and forearm, even a wrist. It's pelvic fins, less well preserved than the pectoral ones, appeared smaller and weaker (). Hence, Tiktaalik was a mosaic – a “fishapod” – demonstrating that fins and limbs are part of a continuum (Shubin et al. Citation2006, Daeschler et al. Citation2006).
Table 1. Geologic time scale, life and tectonics
Another “fishapod”, Acanthostega, found in 1987, had large gills and a fish-like tail. However, it also had limbs, but these look delicate, more flipper than leg-like, and seem unable to bear the animal's weight on land. Thus, although Acanthostega had limbs, it may never have left the water, indicating legs evolved in water, not on land (Clack Citation2002).
Molecular genetic studies recently underpinned that the difference between fins and limbs is made by subtle changes in a relatively small number of genetic switches (Davis et al. Citation2007). No extraordinary processes or genetic mechanisms are needed to explain the fin to limb transformation. This contrasts with “classical” evolutionary thinking that assumed severe draughts in the Devonian “forced the fish out of the water” in a “major evolutionary event”. Recent findings suggest tetrapods followed the lead of plants and invertebrates when the time was right. Only after land was colonized by plants and invertebrates, vertebrates could follow. However, once this transition was firmly grounded, a huge diversity of forms emerged during the Carboniferous (360–300 mya) and Permian (300–250 mya), some of which looked unlike anything living today. However, the Permian ended with an utterly catastrophic extinction event (250 mya). Whereas the K/T extinction event (K/T stands for Cretaceous/Tertiairy, ) of 65 mya has been popularized by the wipe-out of Dinosaurs (Alvarez Citation1998), the Permian extinction event was far more annihilating: 90% of marine species and 70% of all land organisms became extinct. In fact, such extinction events are regularly recurring events on a geological scale (Raup and Sepkoski Citation1986), although decreasing in severity due to optimization of fitness with evolutionary time ().
Tetrapods start walking with limbs perpendicular to the trunk
Tetrapods include amphibians, reptiles, dinosaurs, birds, and mammals. In tetrapods the pectoral ring no longer has bony attachments to the cranium, but attaches to the vertebral collum through muscles. In contrast, the pelvic ring is no longer a free element, but attached to the vertebral collum through the sacral ribs ( and ).
The best known early amphibian, Ichtyostega was discovered in 1932 in 365 million year old rocks in Greenland () (Jarvik Citation1996). Unlike Acanthostega, its spine may not have been very flexible laterally (sideways), but more so dorsoventrally (up and down). So it was probably not a very efficient swimmer, and walked with a stiff trunk, or shuffled around somewhat like a an inchworm, with two front and two hind limbs moving simultaneously (Ahlberg et al. Citation2005).
Whatever its locomotion, the hip joint in Ichtyostega was shaped roughly like a trough () and corresponding cam (), appearing far more “primitive” than the knee, which already had two distinct femoral condyles and a popliteal fossa (Jarvik Citation1996).
Figure 4. Triradiate form of pelvic bones in A. a lobe finned fossil Eusthenopteron, B. an early tetrapod, Ichtyostega, and C. an extant mammal, the mole (from Jarvik 1980).
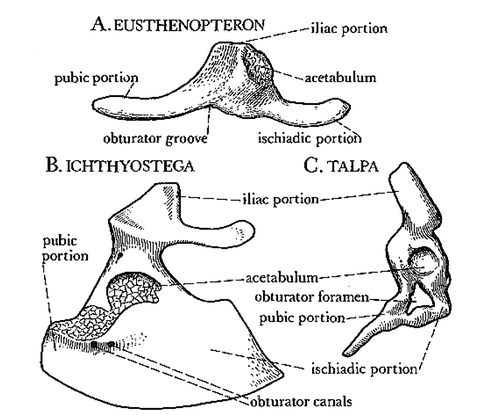
Figure 5. Tiktaalik roseae, a fishapod from the Canadian Arctic, which was equatorial at that time, 375 mya (reprinted by permission from Macmillan Publishers Ltd: Daeschler Citation2006).
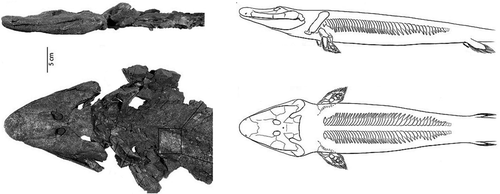
Figure 6. Extinction events such as the K/T extinction that wiped out the dinosaurs (except birds) are regularly recurring events on a geological scale (Raup and Sepkoski Citation1986). K/T stands for Cretaceous / Tertiary, marking the end of the Cretaceous Period (in geologic abbreviations the C was already taken for Carboniferous, hence K for “Kreidzeit”). The x-axis shows geological time, the letters mark the geological periods. The y-axis shows the percentage of marine genera that got extinct during these events. Percentage extinction of species is even higher because a genus contains many species making it much harder to extinguish a genus than a species. Note, though, decreasing severity of extinction events with time (adapted from Sepkoski 1996, Dawkins Citation2004).
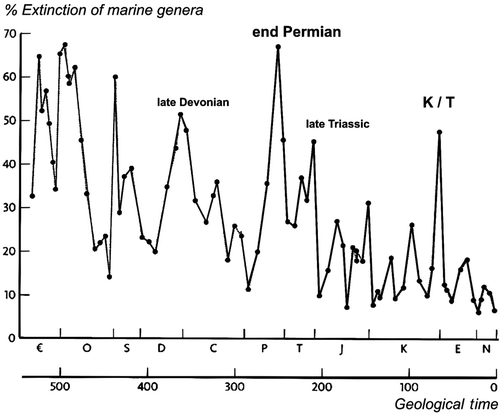
Figure 7. Ichtyostega. Note 7 toes on “foot” (from Clack Citation2002, with permission).
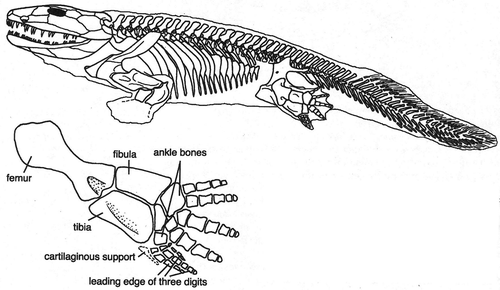
In later amphibians and early reptiles the limbs grow larger and stronger, but are still flexed and perpendicular to the long axis of the body, as the paired fins in fish. For a fish, the orientation of the fins relative to the body axis has no consequence for energy consumption. But walking on land is far more energy efficient when the limbs are under instead of perpendicular to the body. With perpendicular limbs, muscle action is constantly needed to prevent the trunk from sagging to the ground, like a permanent “push-up” (). With the limbs more extended and under the body, muscle action is needed for balance and locomotion, but not for lifting the trunk off the ground (Hutchinson and Gatesy Citation2000). Amphibians and early reptiles walked with a sprawling gait, with an almost horizontal movement of the humerus and femur, with the adductors carrying the bodyweight. Rotation around the long axis of the humerus and femur helped increase step length. Sideways flexibility of the spine creates a wave-like trunk movement (a vestige of its fish ancestors' propulsion), likewise increasing step length. But this trunk movement is not only energy-inefficient for walking, it also hinders breathing because the sideways flexing alternately compresses one lung, shunting stale air to the other lung, decreasing breathing efficiency (Carrier Citation1987). This is why amphibians can move about in only short bursts of activity, after which they have to stop and breathe. Several evolutionary developments subsequently bypassed this constraint. Walking upright, decoupling walking from breathing, is one. Development of a diaphragm or shifting the wavelike motion to the vertical plane (e.g. galloping quadrupeds) are other examples.
Another problem for a sprawler that arises with larger body size is the required muscle strength. The problem with upscaling body size is that strength of a muscle is proportionate to its area of cross section (πr2), whereas mass increases with volume, i.e. the cube (3rd power) of linear dimensions. Thus, an animal that grows to twice its linear dimensions will have four times the muscle strength, but its weight will be eight times the original weight. Further, muscle moment arms scale linear rather than squared, and particularly for the adductors used to lift the body off the ground in a sprawling posture: the trunk simply becomes too heavy for this posture type.
Reptiles shift their limbs under the trunk
Early reptiles evolved from amphibians approximately 350 mya. Reptiles, unlike amphibians, are no longer dependent on water to breed because their eggs have a membrane that prevents them from drying out when not in water (amniote eggs). In mammals these membranes became the amniotic sac that surrounds the foetus. Reptiles started walking in a more upright position. This semi-erect gait has the front and hind limbs () aligned more with the long axis of the trunk and thus closer to the body axis. The front limb was repositioned with the elbow facing backwards (caudally), the hind limb with the knee facing forwards (cranially). This shift can be seen today when a crocodile goes from walking in sprawling gait, to running with the hind limb more under its trunk in semi-erect gait. Perhaps this means a crocodile has about the maximum weight (800–1000 kg) compatible with sprawling gait). This position also shifts the task of stabilizing the body over the hind limb from the adductors to the abductors (Hutchinson and Gatesy Citation2000). The repositioning of the hind limb changed the hip joint: the proximal end of the femur evolved from the cam-like shape in amphibians to a more rounded cam or oval shape, with corresponding shape changes in the acetabulum (Tables and ).
Table 2. Pelvis types
Table 3. Proximal femur types
Dinosaurs start walking upright
In the reptilian superorder of dinosaurs, evolved from reptiles 230 mya, the transition to erect posture was completed. A vertical limb can carry much heavier loads than the same limb loaded horizontally, thus dinosaurs could grow huge sizes compared to amphibians. Vertical limb position and more round hip joint shapes also gave dinosaurs a greater stride length. Further, erect posture decoupled walking from breathing, increasing stamina as running no longer counteracted breathing. In erect posture, forces in the acetabulum were redirected, from medially in the sprawling and semi-erect postures, to dorsally in the fully erect posture (). Dinosaurs therefore had less need for a sturdy bony buttress in the medial (inner) wall of the acetabulum. In fact, one of the hallmarks of a dinosaur is an “open” acetabulum (). The inner wall may have consisted of cartilage, but this is not preserved in fossils.
Bipedalism becomes possible when the hind limb is positioned under the trunk, freeing the front limbs for other tasks such as feeding and fighting. All carnivorous dinosaurs and many others were bipedal, their balance assisted by a slender but long tail. Indeed, one of many “reversals” in evolution is that some quadrupedal dinosaurs evolved from bipedal ancestors (Hutchinson and Gatesy Citation2006).
Bipedal dinosaurs had a more cylindrical femoral head and several species had what looked a bit like a femoral neck. Consequently the outer (lateral) part of the neck began to look like a greater trochanter (). However, this lateral margin of the dinosaur's proximal femur had few if any muscle insertions and was probably covered with cartilage, presumably continuous with the femoral head. It was not comparable in relative size to the “true” greater trochanter seen in mammals, nor did it likely have a similar function: perhaps it was a site for a bursa over which femoral protractors (flexors, such as m. iliofemoralis and iliofibularis) passed, or only a continuation of the femoral head (Walker Citation1977). Given these considerations, the ossification of the proximal femur during growth of a dinosaur likely arose from one single, continuous epiphysis (“coalesced”, see below).
The shift to bipedalism can today be seen in some types of quadrupedal lizards, that, without specific anatomic adaptations, can go from quadrupedal gait to upright bipedal running.
Small mammals in a dinosaur world
Mammals began to evolve 300 mya from synapsid reptiles, so before dinosaurs evolved. Synapsids (“fused arch”) derive their name from the openings in their skull. Synapsids also have the first osteochondral connection between sacrum and ilium (Romer and Price Citation1940). An early example, one of the earliest ancestors of mammals, was the pelycosaur Dimetrodon (“two shapes of tooth”), which, by all appearances would have passed for a dinosaur-type animal () at over 3 m long and with the great “sail” on its back. Did its sail attract mates? Frighten off enemies? Control body temperature? We don't have a clue. We do know Dimetrodon had a proximal femur with a rounded cam, without femoral neck or greater trochanter, much more like Ichtyostega than a dinosaur (compare to and ). This makes sense because Dimetrodon lived 280 mya, long before dinosaurs began to evolve (230 mya) from reptiles. We are used to seeing mammals as the “evolutionary successors” of dinosaurs after their K/T extinction, but the mammal-like reptiles flourished before the era of dinosaurs.
Figure 8. Ichtyostega femur (from Jarvik Citation1996). Ichtyostega acetabulum is shown in .
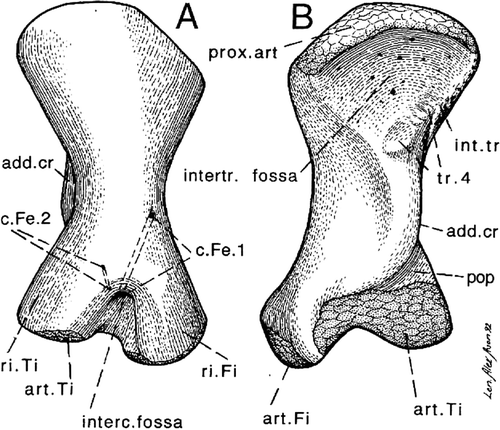
Figure 9. Posture types (adapted from Jenkins Citation1971).
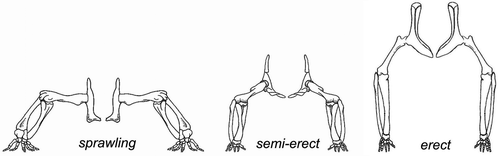
Figure 10. Saurischian (Antrodemus, left) and ornitischian (Thescelosaurus, right) dinosaur pelvis and femur. Both have an “open” acetabulum. Femora in posterior view, acetabula in lateral view (from Romer 1957).
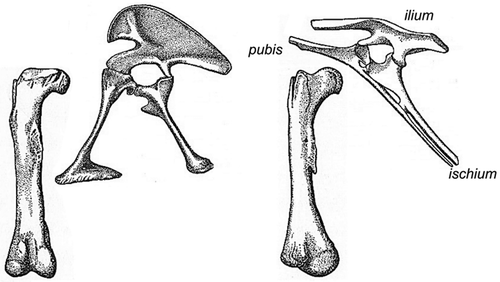
Figure 11. Dimetrodon was an early mammal-like reptile of 280 mya. Note it has a medial wall in its acetabulum, and a femur resembling that of Ichtyostega. Acetabulum in lateral view and femur posterior view (from Romer 1957). Reconstructions of skeleton and body abound on the internet, but no one knows what colour Dimetrodon was.
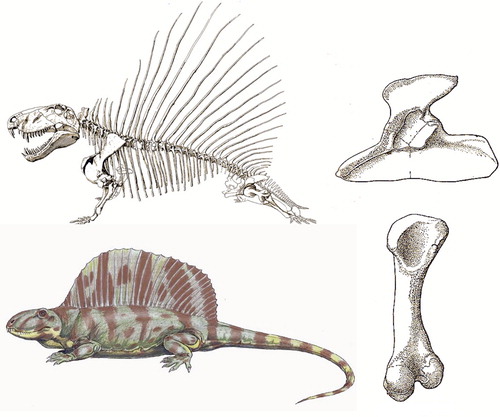
About 200 mya the first “true” mammals appear (Jenkins Citation1971), small hairy animals that can maintain a higher body temperature. They have sweat glands, and in females some of these glands specialized to produce milk to feed the young (). But soft tissues are rarely if ever preserved for palaeontologists. Instead, the signature feature that distinguishes mammals from reptiles and indeed all other vertebrates is the presence of two small bones in the middle ear. The evolution of the middle ear auditory ossicles from reptilian jawbones is a prime example of exaptation, where a structure finds a new purpose during evolution. It is supported by transitional forms (Kermack and Mussett Citation1981) and molecular-genetic evidence (Tucker and Watson Citation2004).
Figure 12. Thrinaxodon Liorhinus, a Cynodont (early mammal), which lived in the mid-Triassic (approx. 230 mya), fossils found in Africa and Antarctica, then joined in Pangaea (from Jenkins Citation1971).
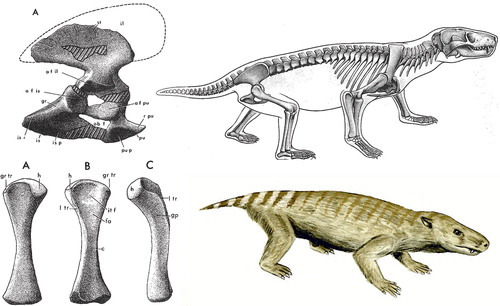
However, for the “new” mammals, life on earth in the ensuing 135 my was dominated by dinosaurs. Nearly all early mammals were nocturnal which led to degeneration of the colour discrimination earlier mammal-like reptiles likely had in their vision. Today, mammals as a group probably have the poorest colour discrimination of all vertebrates. An exception are apes and monkeys where, at least twice, colour (trichromatic) vision re-evolved independently in South America and Africa (Dawkins Citation2004).
Mammals in the dinosaur-world were always small. In fact, for more than two thirds of their entire existence, mammals were not larger than cats, and most were the size of rats and mice. Then, following the mass extinction event of 65 mya that ended the era of large dinosaurs, some mammal species survive, and within the evolutionary blink of an eye, 2–3 my, we see development of massive mammals and explosive radiation to a plethora of different species. Evolution to recent mammals was characterized by extinction of the large mammals, approximately 40,000 years ago – possible causes such as climate change or hunting by man are hotly debated among palaeontologists (Kemp Citation2004).
Two hip types in extant mammals
Large variation exists in hip morphology in extant mammals, but within this variation, two types of hip can be distinguished. These types can be characterized morphologically as a sturdy hip (coxa firma or coxa recta) or a mobile hip (coxa mobilis or coxa rotunda), based on differences in roundness of the head and the position of the head on the femoral neck. Roundness or sphericity of the femoral head can be quantified with the alpha angle for the anterior head-neck junction (Notzli et al. Citation2002) and its analogues such as the beta angle for the posterior head-neck junction ( and 31) (Toogood et al. Citation2009). Femoral head roundness is similar for both types for the portion that is within the acetabulum in habitual standing position. But at the junction of the femoral head and neck a coxa recta has a straight section whereas a coxa rotunda exhibits continued roundness (examples in , , , and ). Related to this roundness is the position or “set” of the head on the femoral neck. The parameter that best clarifies this aspect of hip morphology is offset: the space available between the head and the neck (), also a measure of the thickness of the neck relative to the head when expressed as a ratio. A coxa recta has low offset, specifically in the straight section of the head-neck junction (). A coxa rotunda has high offset, increasing range of impingement-free motion within the acetabulum ( and ). Head roundness and offset are thus inversely related: higher anterior offset correlates to a lower alpha angle, higher posterior offset correlates to lower beta angle (). Both sphericity and offset thus shape the “concavity” of the head-neck junction (Toogood et al. Citation2009), which determines the bony limit of femoral head motion in the acetabulum.
Figure 13. The femoral neck can be characterized by its length, width and the angle it forms with the shaft of the femur in 2 planes (anteversion and neck-shaft angle). Offset: the maximum space between femoral neck and a line drawn parallel to it along the femoral head. Green arrow posterior (dorsal) offset, yellow arrow anterior offset. Anterior/cranial is up, lateral is to the left. Lines drawn on cast of femur of Turkana Boy, a 15 year old Homo erectus that lived 1.5 mya ().
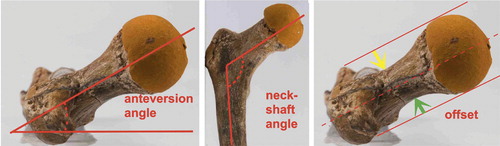
Examples
Most larger mammals have a coxa recta. This hip type is best exemplified by cursorials, mammals that do a lot of standing and running. Horses are a prime example. But also carnivores, like a wolf, and ground-dwelling monkeys such as mandrills or baboons have a coxa recta (although the femoral head is rounder in monkeys than in cursorials, ). Correspondingly, the body posture and locomotion of these monkeys is quite similar to canids (dogs, wolves, etc; ), and so is the relation of the femoral head to neck ().
Figure 14. Variation in coxa recta hip morphology in (left to right): an ungulate, the horse (Equus caballus); a carnivore, the wolf (Canis lupus); and a ground-dwelling monkey, the mandrill (Mandrillus sphinx). Top two rows show AP view of femur. Below, the third row shows the anteversion of the femoral neck (as seen in the long axis of the femur, anterior is up). Femoral head of Equus has (normal) large groove posteriorly at teres ligament insertion site. Bottom row shows (axial) view perpendicular to the femoral neck with reduced offset and straight section of posterior head-neck junction compared to anterior, where heads are round and offset much higher.
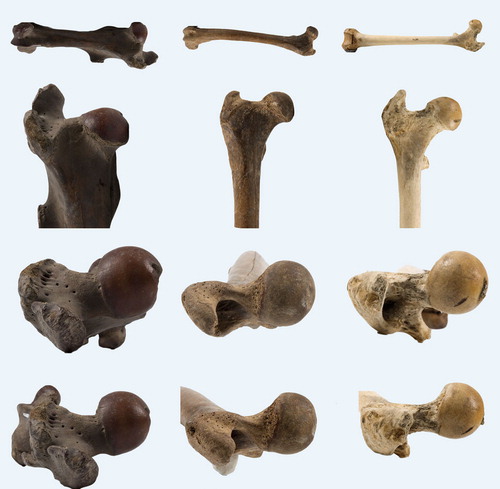
Figure 15. Skeletal (not cranial) similarities between dog (left) and ground dwelling monkey (mandrill, right).
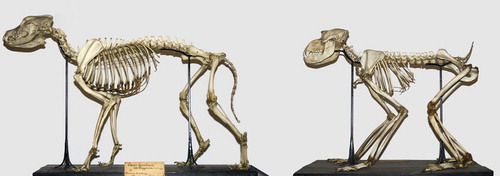
Figure 16. Beta angles in a monkey coxa recta (80°, mandrill, left) and an ape coxa rotunda (42°, chimpanzee, right). Also note differences in anterior and posterior head-neck offset and neck version between coxa recta and coxa rotunda. Mandrill has 10° anteversion, chimpanzee has 12° retroversion. View is perpendicular to the femoral neck, anterior is up, posterior is down.
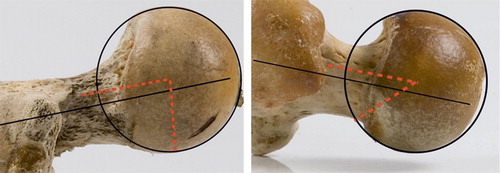
Apes (today: gorilla, chimpanzee, bonobo, orangutan) have a round femoral head, positioned more symmetrically on the femoral neck, with higher offset. But several marine species (e.g. seals) and many rodents also have a coxa rotunda ().
Figure 17. Variation in coxa rotunda hip morphology. From left to right: two apes, a chimpanzee and an orangutan; a semi-aquatic rodent, the beaver (Castor fiber), and a marine mammal, the seal (Phoca vitulina). Top two rows shows AP view of femur. Below, third row shows version of femoral neck (anterior is up). Note all have retroversion of the neck except Castor. Bottom row shows (axial) view perpendicular to the femoral neck with offset both anteriorly and posteriorly, and a round femoral head.
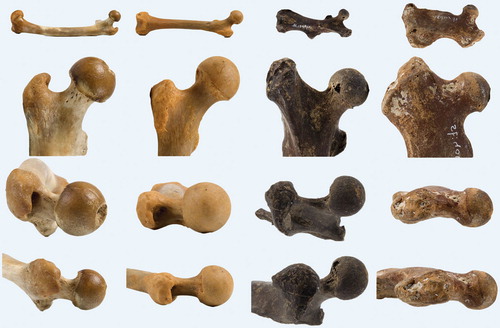
Development aspects
These two hip types are the outcome of developmental differences in the proximal femur. In early growth, all mammalian species have one epiphysis for both greater trochanter and femoral head. In the majority of mammal species this epiphysis remains one single entity (“coalesced”), resulting in a coxa recta hip type. But in several species, including apes, the epiphysis is separates in two parts during growth (“separate”), one for the femoral head, one for the greater trochanter () (Serrat et al. Citation2007).
Figure 18. Histological sections of proximal femur from a 7-day-old (left) and 28-day-old mouse (right). During early postnatal development, the entire proximal end of the femur consists of a single chondroepiphysis (top). Later in growth this epiphysis is separated in two, one for the femoral head and one for the greater trochanter (below). These two epiphyses remain separate throughout the rest of growth. Scale bar = 200 µm (from Serrat Citation2007).
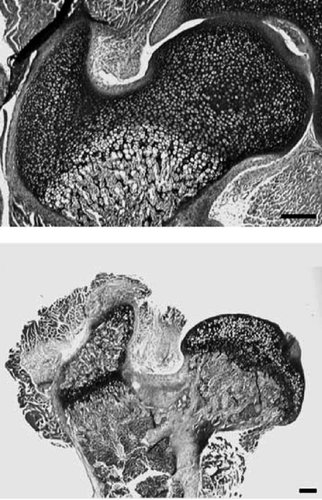
Interpretation
A straightforward phylogenetic explanation for these hip types is not available, in other words, one type is not older or more “primitive” than the other (Serrat et al. Citation2007). Body weight appears to play a role only at the extremes of the range, with the lightest mammals (rodents) having a coxa rotunda and separate ossification pattern, and the largest, the elephant, having a coalesced ossification pattern, with a round femoral head on a very thick and vertical femoral neck (neck-shaft angle approx. 160º).
When hip movements and loading are examined in more detail, it appears that mammals requiring a large range of hip motion, specifically rotation, have a coxa rotunda and a “separate” hip ossification pattern. For example, apes use a wide range of hip motion in climbing, including internal and external rotation for grasping with their opposable big toe. Aquatic mammals such as seals likely also benefit from hip rotation in the long axis of their flipper to increase swimming efficiency.
Mammals with more stereotyped, less varied hip motion have a coxa recta with coalesced ossification pattern, for example ungulates such as horses that use their hip almost exclusively in a limited flexion–extension range, with little, if any rotation. In a coxa recta the femoral head cartilage extends onto the neck, giving the femoral head a shape somewhat like a bicycle helmet, suggesting this cartilage is not covered by acetabular cartilage during normal function, but a large labrum covers most of the lateral extension of the head on the neck.
No quantitative studies have been done to compare the mechanical characteristics of the two hip types. Even so, the morphology of the head neck junction in a coxa recta can be interpreted to increase tensile strength. Low offset, or even better, a straight section, is found where the highest tensile stress is expected. For example, offset is low in the area (cranio-dorsally) where the highest rate of tensile loading can be expected in a galloping horse. Likewise, in a kangaroo, offset is completely absent and the head-neck junction straight, at the cranio-dorsal head-neck area, precisely where forces of landing of the jumping animal are transmitted ().
Figure 19. Kangaroo posture is characterized by deep flexion in hips and knees. Coxa recta hip morphology is pronounced with no offset whatsoever on the postero-superior head-neck junction, where tensile forces are largest when a kangaroo lands a jump, and joint contact area is maximized.
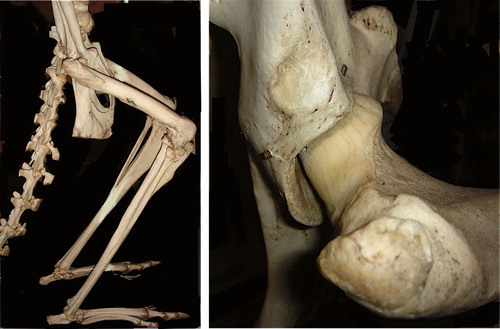
A coxa rotunda has high offset, increasing range of impingement-free motion within the acetabulum, at the expense of tensile strength of the femoral head-neck junction. In coxa rotunda the neck is also relatively longer (), further increasing range of motion of the hip.
Apes evolve a stiff back and mobile hips
Primates (apes, monkeys and lemurs), share a last common ancestor 65 mya, i.e. they were already a separate lineage of mammals at the K/T extinction. Monkeys (e.g. baboons) are quadrupeds with a tail and overall body architecture quite similar to canids (). Apes, as opposed to monkeys and the majority of all other mammals, have no tail- why is unknown.
The apes emerged about 25 mya, and as they grew to larger sizes their lumbar spine changed to a stiff “shortback” (Schultz Citation1950) () Their ilium “wings” (cranial part) lengthened, while the number of lumbar vertebrae decreased to three or four, bringing the ribcage very close to the ilium. This creates a stiff “unibody” with biomechanical advantages for the larger apes as they climb and swing through trees, also reducing the risk of spinal lesions after a fall. As with limb proportions in reptiles, size is an issue: in smaller and lighter primates a relatively long and more mobile lumbar spine is not a liability whereas the mobility of the lumbar spine increases their efficiency to jump (compare quadrupeds such as hares that use their flexible lumbar spine to increase stride length).
Figure 20. Evolution of long flexible lumbar spine in monkeys (left, macaque) to a stiffer construct in apes (gibbon), unibody in chimpanzee (Note 3 lumbar vertebrae) and back to longer and more flexible lumbar spine in man (scales normalized for comparison to same distance of 1st thoracic vertebra to ischium. Adapted from Schultz Citation1950, with permission).
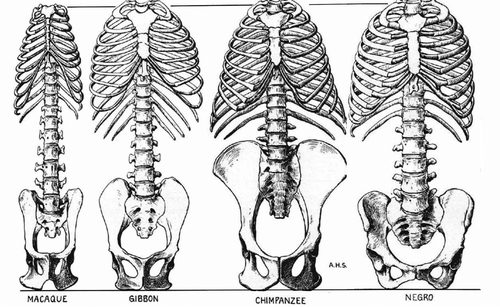
In contrast to the stiffening of the spine, the hip became more mobile in apes to facilitate tree climbing: a shallower acetabulum developed and femoral heads became more round than those in their quadrupedal ancestors (Jenkins Citation1977, Schultz Citation1969) ().
Early humans develop into an extraordinary primate indeed: an ape with an enormous brain, walking upright
Man's evolution from the apes features three unique developments: bipedal gait and encephalization (the third feature, elongation of life phases, is beyond current scope (Schultz Citation1950, Gibbons Citation2008)). The concept of encephalization was proposed by Dubois (who started the field of paleoanthropology with his discovery of “Java man”, and ), when he documented brain size in relation to body size (Dubois Citation1897). Although many species can use bipedal gait, Man is the only primate that made bipedal gait its exclusive form of transport. Furthermore, humans had their birth process re-engineered to accommodate the spectacular size increase of their brains. Both changes have had enormous impact on the pelvis, making this the most defining skeletal element to “read” human evolution.
Figure 21. Encephalization as a pattern shift in brain and skull growth: differential rate and duration of growth underlies marked differences in skull and jaw shape (adapted from Dawkins Citation2004, with permission).

Figure 22. Evolution of the Hominin femur from left to right: Lucy (Australopithecus afarensis, 3.2 my); Java Man (Homo erectus, 1.5 my, note traumatic defect on anterior femoral head and myositis ossificans-like lesion on medial diaphysis); Homo sapiens female; H. sapiens male. H. erectus and both H. sapiens femora are from Dubois collection. Lucy femur has no femoral condyles, anteversion of femoral neck is therefore uncertain. Note variable rotation on AP views (2 top rows) giving variable impression of femoral neck length. Also note coxa rotunda in female and coxa recta with high anteversion in male H. sapiens. Scales are approximate.
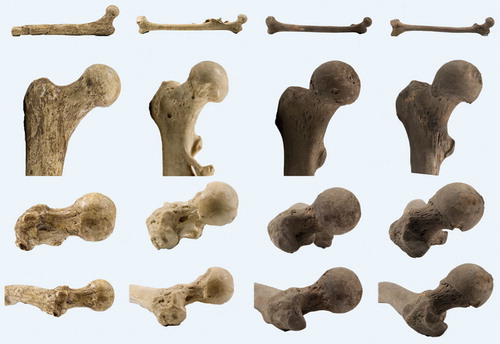
Early humans share a last common ancestor about 6 mya (range 5.5–7.7 mya, (Sibley and Ahlquist Citation1987)) with chimpanzees (and bonobos), that's approximately 250,000 generations ago. Many species and many “dead ends” characterize this evolution, most of which took place in Africa. Homo sapiens is present in only about the last 3% of total time of the evolution of the apes (Kingdon Citation2003), and evolution of the pelvis and hip certainly predated Homo's origin (Carroll Citation2005).
In the last 15 years spectacular fossils were found that demonstrate the transition from ape to human (; see also Gibbons Citation2006). These are mostly called hominins (humans and our ancestors, but not other apes), or hominids, a term for “great apes”, including the extinct and extant humans, chimpanzees, gorillas, and orangutans. Heated debate usually follows the claims based on a new fossil's morphology. The two most recent were the proximal femur of Orrorin tugenensis (Kenya, 2000) and the skull of Toumai or Sahelanthropus tchadensis (Tchad, 2002). Orrorin, dated at six million years, is claimed as a bipedal hominid (), primarily based on the ossification pattern of the femoral neck (Galik et al. Citation2004). Toumai, 6–7 million years old (Brunet et al. Citation2002), is thought to have walked upright because of the position of the foramen magnum in the skull and the insertion site for the neck muscles at the back of the skull. The dating of both fossils is not contested, and debate now focuses on whether they were indeed bipedal, because if both were, Orrorin lived close to, and Toumai possibly even before the split with chimpanzees. This would mean either a very short time for bipedality to evolve, or repeated evolution of bipedality at more than one time, or that our common ancestors with chimpanzees were at one time bipedal and then reverted to all fours (Dawkins Citation2004).
Figure 23. Femur of Orrorin tugenensis (from Galik Citation2004, reprinted with permission from AAAS).
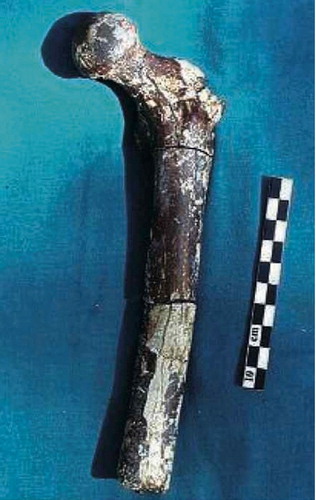
Table 4. Human evolution from Toumai to Homo (for review see Gibbons, Citation2006).
Why did early humans develop these peculiar traits?
Evolution has no plan or goal, it just happens. A strict “why” is therefore irrelevant, but what were the evolutionary drivers for encephalization and bipedality? Quite a few theories have been proposed. As to the relation between encephalization and bipedality, it is now clear bipedality came first: “Lucy” (see below) was bipedal, but her brain was not much larger than a chimpanzee's (). But neither was tool use the “cause” since bipedality predates estimates of first tool use by several millions of years (Lovejoy Citation1981).
Some of the better known hypotheses to explain walking upright are: freeing the hands to carry food or infants over large distances (Lovejoy Citation1988), walking upright on branches (Thorpe et al. Citation2007, Preuschoft Citation2004), squat feeding (Kingdon Citation2003), increasing the visual horizon, providing an advantage for feeding in savannah-woodlands and better escape from predators (Videan and McGrew Citation2002), and feeding in a water environment – the aquatic or wading ape (Morgan Citation1997).
However, any explanation for bipedality, and even more so for encephalization, will have to deal with one important point: why are humans the only apes with these traits? In other words, if there is a clear evolutionary benefit in growing a huge brain and walking upright, why did not more apes, primates or even mammals do it?
Bipedality appears the easier trait to explain, because walking upright is a normal activity for other apes (e.g. as they walk across branches (Thorpe et al. Citation2007), and its further evolution in Homo could simply be a quantitative shift in existing behaviour (Dawkins Citation2004). For chimpanzees however, walking or running on two legs is not faster or more energy efficient than on four (Taylor and Rowntree Citation1973). Looking at energy cost, the evolutionary benefit of a huge brain is even more questionable: a huge brain is also a huge energy expenditure (approx. 25% of total in an adult human, up to 60% of total in an infant). Thus, another type of evolutionary driver must have played a role in what appears to be the non-utilitarian initial development of both traits, particularly encephalization. This driver may be sexual selection. Sexual selection has the dual effect of enhancing a given trait in male offspring and inheriting the preference for this trait in female offspring (Darwin Citation1871). Working in lockstep in both partners, sexual selection can produce rapid spurts of evolution in arbitrary directions, creating bizarre extremes as the peacock's tail and the “gardens” of birds of paradise (Dawkins Citation1996).
Miller argued that up to 50 percent of human genes are expressed through or in the brain. For an (early) human, it then makes sense to judge the reproductive health of a prospective mate by his or her brain, or more accurately, by judging brain products such as hunting, but also speech, play and art. Clearly, these were not consciously articulated goals of individual early humans, but evolutionary mechanisms that increase the reproductive success of a species. In this view, the human mind is open to sexual selection and rapid and far-reaching evolutionary change (Miller Citation2000). And that is what happened: brain size of early humans roughly doubled from 450 to 900 ml in the period from 2 mya to 0.7 mya (; ). Body size also increased, but an even bigger relative increase in brain size occurred from 0.5 to 0.1 mya, say from late Homo erectus to early Homo sapiens (). This is one of the most remarkable changes in the evolution of any mammal and a prime demonstration of a runaway effect (Johanson and Elgar Citation2001) or “software-hardware-co-evolution” (Dawkins Citation1996). Compared to similar sized mammals, chimpanzees have approximately twice their brain size, but a modern human six times this size (Jerison Citation1973). Such momentous size increase was no longer compatible with “ape-style” birth. Both “intra-” and “extra-pelvic” adaptations evolved. Delay of most of brain growth until after birth was probably most effective (neoteny, see below).
How did these peculiar human traits develop?
What evolutionary developments made walking upright and enormous brains possible? The key to understanding form is development, the process from egg to animal. Spectacular progress in developmental biology in the last two decades has substantially clarified our understanding of how bodies are built, showing that virtually endless variation in form is developed with an identical toolbox in insects, vertebrates and other animals (Shubin Citation2008). Morphological change is no longer seen as a consequence of a change in a gene, but a change in the regulation of genes: the turning on and off of genes at specific times and places during embryogenesis and growth (Wolpert Citation2006). This is evolutionary-developmental biology, Evo-Devo in short (Carroll Citation2005). Most important are genes that affect number, shape or size of a structure. Stunning similarity is present in these toolbox “master” genes (homeobox or hox genes) between animal groups that diverged in evolution over 600 mya. The profound morphological changes in pelvis and hip from chimpanzee to human thus result from “pattern shifts” in the regulation of the genes involved, the genes themselves are virtually identical (Lovejoy et al. Citation2002).
This contrasts to thinking for over a century that development of bone and joints was primarily determined by mechanical loads. Among many mechanical parameters, loading history and, specifically, bone strain have the strongest influence on osteoblast activity (Carter and Wong Citation1988, Shefelbine and Carter Citation2004), the more popular expedient of this interaction known as Wolff's law (Prendergast and Huiskes Citation1995). However, recent advances in developmental biology show loading history is not the only, nor even the primary determinant of resulting bone and joint morphology (for review see (Wolpert Citation2006, Lovejoy et al. Citation2002). An integrated system of sequentially expressed gene arrays determines bone and joint morphology; mechanical parameters mainly provide threshold values for these gene actions.
The 5 nuts and bolts of bipedalism
The development of habitual bipedal gait hinged upon changes in the lumbosacral spine and pelvis- far more than changes in the hip joint. But pelvic changes in turn influenced the hip, leading to pronounced differences between male and female human hips. Encephalization not only co-evolved with key pelvic shape changes, but also depended on adaptations outside the pelvis such as neoteny. These developments are summarized in 5 paragraphs below, but clearly are intertwined ( and ).
1. Lumbar lordosis increases efficiency of bipedal gait
When a chimpanzee walks upright, the pelvis is more vertical, but the hip maintains about the same position in the pelvis, i.e., is not extended () (Jenkins Citation1972). This is not caused by a lack of possible extension of the hip joint, but by a stiff lumbar spine that lacks lordosis (extension or retroflexion). The chimpanzee walks with flexion of both hips and knees to compensate the forward bent position of the spine that keeps the centre of mass of the upper body forward (ventral) to the sacrum. This “bent-hip, bent-knee gait” brings this centre of mass closer to the point of ground contact of the feet, but at the price of considerable work for hip, knee and back muscles (Carey and Crompton Citation2005).
Figure 24. Chimpanzee has a Trendelenburg gait due to lack of efficient abductors and walks with flexed hips and knees because its stiff spine remains in forward flexion (from Jenkins Citation1972, reprinted with permission from AAAS).
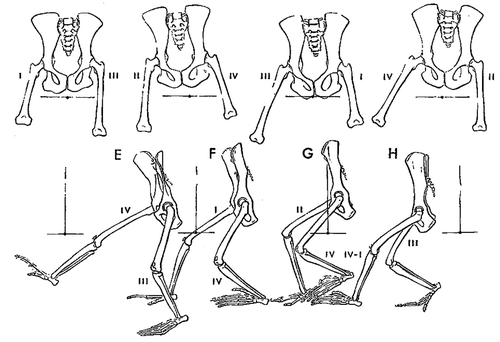
When a human walks upright, hips and knees can be in much more extended positions because lumbar lordosis aligns the centre of mass of the upper body with the sacrum and the point of ground contact. Lordosis was made possible when considerable reduction in height of the ilium “freed-up” the lumbar spine (), which itself lengthened when the number of lumbar vertebrae increased to five or six. A larger distance developed between lower ribs and iliac crest, creating a waist in the human trunk (as opposed to the barrel shaped ape trunk). The sacrum broadened markedly and tilted forward (horizontally). Changes in the shape and position of the facet joints further increased lumbar spine mobility (Lovejoy Citation2005). Lumbar lordosis thus benefits energy-efficient upright walking. In humans, standing erect requires only 7% more energy than lying down. Dogs use considerably more energy standing than lying, presumably because they have their hind legs flexed (Abitbol Citation1988).
The downside of this “mobilization” of the lumbar spine may be a relatively insufficient erector spinae muscle. The potential for hypertrophy is likely hampered by the dorsal position of the transverse processes that developed with the “shortback”. Scoliosis and spondylolisthesis are virtually absent in the great apes. Perhaps these spinal malformations in humans are associated with the length and mobility of the lumbar spine and a relative erector spinae muscle insufficiency (Lovejoy Citation2005).
2. The pelvis changes from long, narrow and flat to short, wide and curved.
Indeed, just about everything changed in pelvic shape going from ape to human, but at about 3 mya, the hominid pelvis had acquired its general form seen in humans today (). This included functioning abductors and hip kinetics that allowed permanent bipedal gait (Lovejoy Citation2005). Thereafter, the most important pelvic changes were driven by further ballooning of the early human brain.
Figure 25. Hominid pelves, from left to right: chimpanzee, Lucy (Australopithecus afarensis, 3.2 my), Homo erectus (1.5 my), Homo sapiens. Note that chimpanzee pelvis has larger AP than ML diameter, then evolves to wider pelvis in Lucy, and from H. erectus pelvis is again increasing in AP diameter by differential growth of the pubic bones. Red asterisk marks ischial spine in Homo pelvis (adapted from Simpson 2008, with permission from AAAS). Scale of chimpanzee pelvis is approximate.
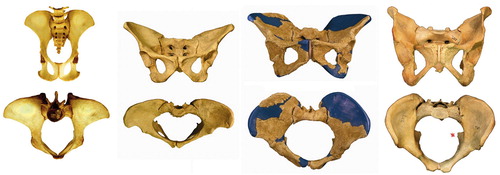
The world-renowned specimen from this era is “Lucy”, or Australopithecus afarensis, a female from East Africa 3.2 mya, that stood around 1.m10 tall (), males stood around 1.30 m). Lucy walked upright, but had a brain size of approx. 450 ml, not much bigger than a chimpanzee's (Johanson and Elgar Citation2001).
Figure 26. Lucy (Australopithecus afarensis) skeleton. Pelvis was reconstructed, see (from Johanson Citation2001, © / courtesy National Museum of Ethiopia).
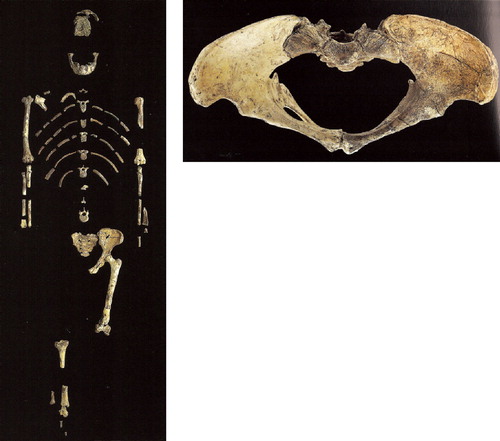
The human pelvis is much more three-dimensional than the long and flat ape pelvis. The ilium wings curve forward, (), repositioning the origin of the anterior gluteal muscles (medius and minimus) and tensor fasciae latae, changing their function from extensors in quadrupedal walking to hip abductors in bipedal walking. Effectiveness of these new abductors is reflected in bony architecture of the femoral neck: (early) humans have little cortical bone in the superior femoral neck whereas chimpanzees (and other apes) have a circumferential ring of cortical bone (). This is because chimpanzees do not have a functional abductor apparatus as such- they walk with a swaying torso (Trendelenburg gait (). The human anterior gluteals (the new abductors) exert compressive stresses on the superior neck negating the tension stresses in this area, resulting in less cortical bone in the superior femoral neck (CitationKalmey and Lovejoy 2002, Ohman et al. Citation1997). Australopithecines like “Lucy” already had this ossification pattern in their femoral neck, indicating they walked upright with well-functioning hip abductors (Lovejoy et al. Citation2002, Ohman et al. Citation1997). Identical findings were claimed after CT scanning the femoral neck of the 6 my old Orrorin (Galik et al. Citation2004), but controversy ensued promptly about the quality of the preparation and scans, and validity of the claims (Ohman et al. Citation2005).
Figure 27. Bone distribution in the femoral neck. From left to right: Chimpanzee, Australopithecus afarensis (AL-128), H. sapiens (from Lovejoy Citation2005, and Ohman Citation1997, with permission).
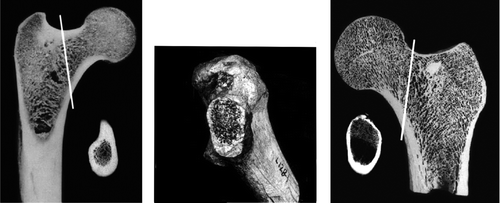
A vertical pelvic wall becomes a horizontal pelvic floor
The transition to upright posture meant that the vertical pelvic wall of quadrupedal mammals became the horizontal pelvic floor in early humans (and the horizontal abdominal floor became a vertical abdominal wall). In humans, the pelvic floor evolved to an essential control system for continence and prevention of prolaps (Abitbol Citation1988). Indeed, the pelvic wall is less adequate in other hominids, for example extended upright positions in chimpanzees and gorillas frequently produce incontinence. Evolution of the pelvic floor is related to the ischial spines (see below), which, in humans, are the attachment site not only for the strong sacrospinous ligament, but also for the tendinous arch of the pelvic fascia.
3. Childbirth
Likely a stronger (and certainly a more direct driver) for evolution than bipedal gait is childbirth. In the last 0.7–0.5 my, spectacular further increase in brain size led to pelvic adaptations, or vice versa. That is, only after enlargement of the birth canal (or “true pelvis”) was further increase in brain size possible. Initial changes in brain size up to ca. 900 ml were accompanied with increase in overall body size (). Up to approximately 0.7–0.5 mya, the true pelvis had roughly the same shape in inlet and outlet, an oval with its largest diameter in M-L direction (M-L = medio-lateral (side to side) () (Tague and Lovejoy Citation1986).
In apes, the fetal head passes in the same orientation through pelvic inlet, – midplane and – outlet (), and because the ape true pelvis has larger A-P than M-L dimensions (), the foetus aligns with its head in this A-P direction (). Although the head size of Lucy's baby was still similar to a chimpanzee's, A-P narrowing of the pelvic inlet and midplane (Figures 25, 28, and 29) caused by the repositioned sacrum, introduced the need for sideways (M-L) position of the head to pass through. The pelvic outlet () was likely passed with the head in the same direction.
Figure 28. Pelvic planes (from Tague Citation1986, with permission).
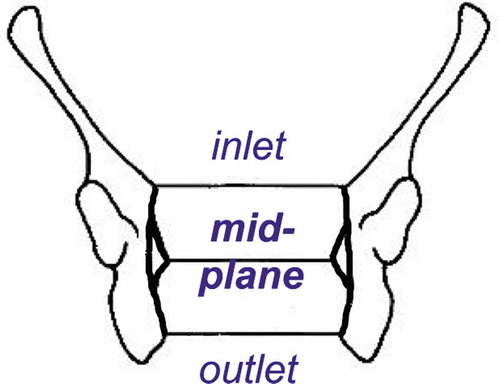
Figure 29. Rotational birth in Homo (from Tague Citation1986, with permission).
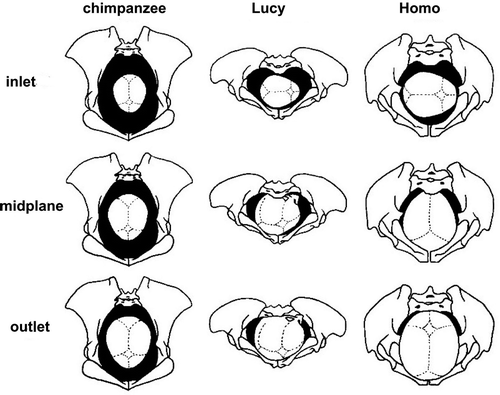
Further increase in brain size required “intra-pelvic” adaptations especially at the pelvic midplane and outlet (widening of the sacrum had already enlarged the pelvic inlet in M-L direction).
The problem of the pelvic midplane is its position between the hip joints. Further M-L widening of the pelvic midplane and outlet would place the hip joints still further apart, increasing the moment arm of body weight, requiring increased abductor work in compensation. To retain an energy efficient bipedal gait, the abductor moment arm, which is the femoral neck, would have to be lengthened. But hominids such as Lucy had already stretched the possibilities for this option, in fact they had a wider pelvis and longer femoral necks, relatively, than any other primate, including current humans (, , and (Lovejoy Citation2005).
A very special pubic symphysis evolves
The solution for enlarging the pelvic midplane and outlet was found in the other, A-P, direction, by forward lengthening the pubic rami and moving sideways of the ischia (). This creates the obtuse subpubic angle seen in the human female pelvis. The ossification pattern of the pubic rami is truly remarkable in modern humans as secondary ossification centres do not fuse before the end of the childbearing age in the third decade. In African apes, pubic secondary ossification centres fuse at the end of adolescent growth along with, for example, the iliac crest growth plate (apophysis). This delay in secondary ossification allows human pubic rami to continue lengthening after growth has long stopped in the remaining skeleton (Lovejoy Citation2005), and prevents premature fusion of the symphysis (as can happen in other hominids). The pelvic outlet was now enlarged (), but in a plane 90º to the largest diameter of the pelvic inlet. Accordingly, the foetal head had to rotate 90º in passing from midplane to outlet, i.e. rotational birth () (Tague and Lovejoy Citation1986).
Ischial spines: obstructing the pelvic midplane?
In the pelvic midplane, the ischial spines appear an awkward feature in pelvic development. In quadrupedal monkeys and other tailed mammals, the ischial spines are the insertion site for tail muscles, are of insignificant size, and located laterally and dorsally near the sacrum. Perhaps surprisingly, in early humans with their ballooning brains, the ischial spines not only grew in size, but did so in a far more obstructing position than in their ape ancestors: centrally in the pelvic midplane (). In humans, the ischial spines are the most critical feature for passage of the foetal head (Abitbol Citation1988). “Lucy” had an intermediate position in this evolution with an ischial spine sized and positioned between humans and chimpanzees (). The human ischial spines develop in the years 6–12 and fully ossify in adulthood. They may have prime importance in development and strength of the pelvic diaphragm (Abitbol Citation1988). For orthopaedic surgeons, the ischial spine is a sign of acetabular retroversion when seen on an A-P pelvic radiograph (Kalberer et al. Citation2008). However, size of the ischial spines is also determined by the presence of an inconstant separate ossification centre (Zimmer Citation1968), highly variable in humans and not related to gender and race, which has not been found in any other mammal (Abitbol Citation1988).
Adaptations outside the pelvis
As human brain size continued to grow, other, “extra-pelvic” adaptations also evolved. One was neoteny: birth of the foetus with an immature brain. Chimpanzees are born with about half their adult brain weight, humans with a quarter. To compensate, the human baby maintains its rapid (foetal) rate of brain growth in its first year, doubling its brain size (Johanson and Elgar Citation2001). By four years, the chimpanzee's brain is nearly finished, whereas the human brain grows until early adolescence, and undergoes substantial restructuring until at least age 15 (Thompson et al. Citation2000).
Another “extra-pelvic” adaptation was assisted birth. Apes do not have rotational birth, the foetus passes relatively easy through the birth canal, typically facing towards the ape mother on exit. With rotational birth, the foetus faces away from the human mother, which means she can have difficulty to clear the airway or remove the umbilical cord from the baby's neck. Extracting the baby in this position can produce hyperextension (neck) injuries. In this situation, an early human female likely had a better chance of survival for her baby and herself when she accepted or sought the help of other females, i.e. assisted birth. Walking upright and a ballooning brain thus transformed birth from an individual to a social enterprise for early humans (Trevathan Citation1996).
4. Upright walking repositioned the hip joint through both pelvic tilt and hip extension.
Comparing with the hip joint of other hominids, the most striking change in early humans is the extension in the hip through both tilting the pelvis more vertical (upright) and extending the hip joint itself (). This “double extension” places the hip joint near the limit of its extension in humans. Chimpanzee bent hip – bent knee gait has about the same position of the femur in the acetabulum as when knuckle-walking, which is in its mid-range (). But the human hip, when standing upright, has about 120º of active flexion, but only about 20º of active extension (Roach and Miles Citation1991). The double extension creates an “anterior uncovering” of the femoral head as seen on a lateral radiograph and CT scans (Weiner, Kelley Citation1993). This position () necessitates a large offset posteriorly and/or smaller posterior acetabular wall to prevent impingement of the femoral neck on the posterior acetabular wall, i.e. to prevent the neck from impacting on the posterior acetabular wall and/or the head from levering out of the acetabulum. This concurs with the observation that the posterior head-neck junction in humans nearly always has good concavity as a result of a low beta angle (a round head posteriorly), or large posterior offset, or both ( and ).
Figure 30. The Double Extension (adapted from Aiello Citation2002, with permission).
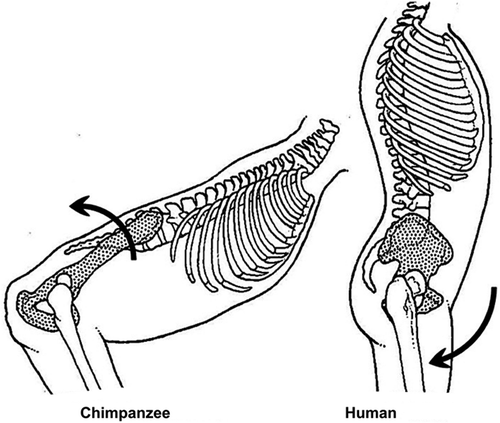
Figure 31. Identical radiographs of the “normal” human hip show, on the left, anterior “uncovering” of femoral head (red crescent). On the right: larger concavity posterior than anterior, in this case due to both low beta angle (beta angle a–c–d = 30°, vs. alpha angle b–c–d = 48°) and increased posterior offset (yellow arrow vs. anterior offset, red arrow). Cross table lateral radiograph (perpendicular to the femoral neck), anterior is up, posterior is down.
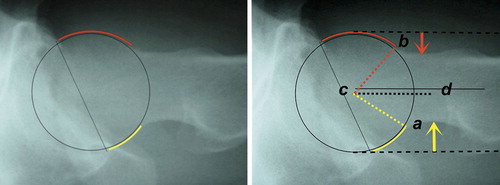
In addition to the double extension, anteversion has increased from chimpanzee to human, both in the femur and acetabulum.
5. Anteversion increases in both acetabulum and femur
Acetabulum: no comparative quantitative data exist on anteversion and inclination of the acetabulum of apes compared to humans (Schultz Citation1969, Jenkins Citation1972, Aiello and Dean Citation2002, Bergé Citation1993, Martin and Saller Citation1959), but chimpanzees have a “laterally facing acetabulum” (Jenkins Citation1972), and humans an “anterior facing” acetabulum (it has higher anteversion). This increase in acetabular anteversion from chimpanzee to human would only increase the need for sufficient posterior head-neck concavity in the human, to prevent impingement of the femoral neck on the posterior acetabular wall when the hip is extended and or rotated laterally. Conversely, retroversion can increase the tolerance of the hip for impingement in extension and lateral rotation. But acetabular retroversion (defined as a crossover sign of the acetabulum anterior and posterior wall) is found in only 6% of the human general population (whereas 20% of human hips with osteoarthrosis have retroversion (Ezoe et al. Citation2006, Giori and Trousdale Citation2003). If retroversion is a torsion of the lower hemipelvis (Kalberer et al. Citation2008), this would cause the ischial spines to narrow the pelvic midplane. Evolution would then likely favour development of anteversion in females, or at least avoid retroversion. Indeed, mean anteversion is 3–5 degrees higher in females than males as measured in the pelvic midplane (Maruyama et al. Citation2001, Stem et al. Citation2006, Murtha et al. Citation2008).
Femoral anteversion is highly variable within and between species (Martin and Saller Citation1959, Tayton Citation2007). Quadrupeds walk with flexed hips and in this position anteversion effectively reduces bending moments on the femoral neck(Tayton Citation2007). Humans walk with extended hips, and in this position the neck-shaft angle reduces the bending moments on the femoral neck. Both anteversion and neck-shaft angle are components of a 3D neck-shaft angle, likely the result of a developmental pattern, influenced by the forces on the proximal femur (Shefelbine and Carter Citation2004). CT measurements in humans without hip problems show a mean value of 24º ± 17° anteversion (range 1°–48° (Strecker 1997)).
Femoral retroversion may be rare in humans, but not so in the apes: in gorillas it is found in 17% (mean 7º anteversion), chimpanzees 29% (, ) (mean 5º, range -11 to +18º) and orangutans 80% (mean 4º, range -13º to +10º) (Martin and Saller Citation1959). The orangutan is remarkable in having the largest range of hip motion of any ape, possibly any mammal. Unique features of the orangutan hip are a high neck-shaft angle, a narrow neck with high head-neck offset, a commonly absent ligamentum teres, a shallow acetabulum and a small or nearly absent acetabular notch (the acetabulum is almost completely filled with cartilage (Schultz Citation1969). This enables the orangutan to scratch its back comfortably, with its foot (), an act of abduction-external rotation impossible for man, even for accomplished yogis ().
The human female and male pelvis and hip differ markedly in shape
The outcome of the 5 nuts and bolts outlined above is a pelvis with profound morphologic differences between the human sexes. In turn, these pelvic differences produce different hip types ( and ). These differences in hip joint morphology are not a female/male dichotomy, but different rates of prevalence of hip types within a continuum (Asfaw Citation1985). Nevertheless, within this continuum, we find in females a higher rate of coxa profunda, in males a coxa recta.
A coxa profunda has a “deep” acetabulum, which means the bottom (fovea) is at or medial to the ilio-ischial line (, ). A coxa profunda has a round femoral head with normal or high head-neck offset () and a high lateral centre-edge angle, it is a deep hip.
Figure 34. Coxa profunda in a 56 year old female: deep position of acetabulum at the ilio-ischial line requires a round head. Head/neck ratio = 1.5
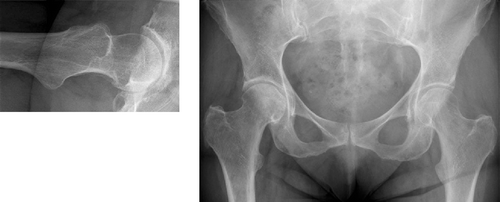
Table 5. differences between coxa profunda and coxa recta
A coxa recta has a straight (aspherical) segment on its antero-superior femoral head, whose cartilage extends onto a thick femoral neck, forming a cam, or pistol grip deformity or impingement bump, decreasing head-neck offset (, ).
Figure 35. Coxa recta in 35 year old male. Straight portion of femoral is seen on both AP pelvis and axial view (left). Note early degenerative changes. Head/neck ratio = 1.3 Orangutan (1.8) Gorilla (1.5) Chimpanzee (1.5) Homo (1.35)
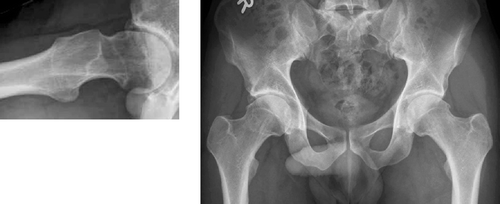
Figure 36. Radiographs of Hominid hips with head-neck ratios (head largest diameter divided by smallest neck width). The human hip has the thickest neck, decreasing offset and impingement-free motion. Also note thicker cortical bone in the superior femoral neck of the apes (from Lovejoy Citation2005, with permission). AP view, not to scale.
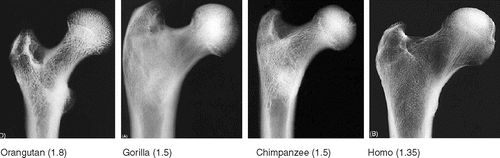
The clinical relevance is that both hip types can lead to osteoarthrosis through femoro-acetabular impingement (FAI) (Ecker Citation2007, Ganz Citation2008). Which hip will develop progressive osteoarthrosis, requiring arthroplasty, and which hip may remain more or less stable radiographically over the years, is currently studied (Bardakos and Villar Citation2009).
Prevalence of hip types has historically been studied using only AP pelvic radiographs. Perhaps the coxa recta is the “tilt deformity” of earlier studies, whose prevalence was reported around 10% in controls. In osteoarthrosis, prevalence of tilt deformity was found about 5 times higher in men than women, at 40–45% (Murray Citation1965, Solomon Citation1976). This is comparable to recent studies that documented cam type deformity (coxa recta) in 17% of men and 4% of women in a cohort of over 3000 unselected Danish persons (Gosvig et al. Citation2008). In addition, in over 1000 young men (Swiss army recruits), decreased internal hip rotation in flexion was related to decreased offset in the antero-superior head-neck junction on MRI scans. Prevalence, hip morphology types and implications of these MRI findings in young men are under examination in this ongoing study (Jüni Citation2009).
In arthroplasty patients, coxa recta was seen in around 40% (Gosvig et al. Citation2007, Ecker et al. 007). For coxa profunda, fewer data are yet available. In persons with mild hip osteoarthrosis it has been reported in 14% (Ecker et al. Citation2007), whereas no data are known from unselected populations. But historical prevalence rates for coxa profunda from several patient populations range between 5 and 20% (Ruelle and Dubois Citation1962).
The majority of features that characterize the female pelvis tend to maximize the size of the birth canal. Thus, widening of the true pelvis places the acetabulae further apart, increasing the lever arm of the body weight on the hips. Lengthening of the femoral neck can compensate this, lowering required abductor work in gait. But there is a limit to how long the femoral neck can be, for example, neck length is related to fracture risk (Faulkner et al. Citation2006). An alternative mechanism that obviates extensive femoral neck length while retaining energy efficient bipedal gait is to place the acetabulum deeper in the pelvis: a coxa profunda. The femoral head in a typical coxa profunda is by definition spherical and with good head-neck offset. In fact, a coxa profunda requires a round head: an aspherical head will not fit in the deep acetabulum in the first place, distorting development of the hip at an early stage.
However, in a coxa profunda the femoral neck can impact on the acetabular rim and labrum, with extension – lateral rotation, abduction or flexion-internal rotation movements (Smith-Petersen Citation2009). The acetabular rim and labrum damage result in osteoarthrosis (Leunig et al. Citation2003), typically seen in women in their fifties and sixties (Ganz et al. Citation2008).
For the male pelvis, obstetric drivers are non-existent; the loading history plays a larger role in determining development and ultimate morphology of the male hip. Once tree climbing was no longer important, evolution and development likely favoured sturdy over mobile hips. Walking upright full-time requires a stronger lower limb and hip than a mixed type of gait. Long distance walking or running do not require a large range of hip motion, but do generate loads in the hip joint of 3 up to 8 times body weight (Bergmann et al. Citation1993), and this in a hominid that roughly doubled in weight (). Although Man may not be a fast sprinter, he is an efficient long distance runner, likely a crucial skill in early hominids for hunting and scavenging (Bramble and Lieberman Citation2004). Consequently, the human hip, particularly in males, developed features typical of the coxa recta seen in cursorials: an aspherical femoral head with asymmetrical head-neck offset on a thick and short femoral neck (, , and ). Only now, in the human coxa recta, following the approximately 50 degrees repositioning of the hip in the double extension, the pars recta is situated antero-superiorly, instead of postero-superiorly as in cursorials.
However, a coxa recta can have femoro-acetabular impingement in flexion – internal rotation movements. What happens is that the pars recta of the head-neck junction is jammed into the acetabulum, producing an outside-in delamination of the acetabular cartilage right behind the labrum (cam impingement, (Ganz et al. Citation2008). Consequently, the clinical hallmark of a coxa recta is decreased internal rotation in flexion, becoming painful with the years as degenerative changes may develop.
Are hip types developmental or a purely mechanical response?
Do the stereotypes, coxa profunda and coxa recta, result from genetic regulatory processes during development and further growth, or are they a primarily mechanical response to loading: Evo-Devo or Wolff's law?
The evolution of the hip and pelvis outlined above substantiates development over adaptation. In other words, a coxa recta results from developmental processes determined by pattern shifts in gene regulation. A specific example is the genetic regulation of growth plate activity. In the growth plate of the femoral head, duration and intensity of (regional) activity shapes the neck-shaft angle, head-neck junction and the femoral head. Mechanical influences modulate this process (Shefelbine and Carter Citation2004), but a coxa recta likely is not the direct result of the amount or type of loading of the femur and hip joint. If this were the case, adolescents with strenuous sports activity would invariably end up with similar hip types, i.e. a coxa recta. Also, differences between male and female hips that have had comparable loading histories (or sports careers) would then be expected to be far less prominent.
Thus, evolutionary–developmental aspects of the hip appear to be the primary determinant in development of femoro-acetabular impingement. Consistently, prospective MRI studies now suggest a coxa recta develops in adolescents at the final stages of growth, mainly by remodelling activity of the antero-superior femoral head epiphysis (unpublished observations). As a consequence, correction of the morphology that produces osteoarthrosis in these hips makes sense when growth has completed. Recurrence of such morphology then is not expected, nor observed at midterm follow-up (Beck et al. Citation2004).
Nevertheless, amount and type of joint loading, for example in sports, may play a role in development of FAI, not only in onset and severity of symptoms, but also in modulating the morphologic development of the head-neck junction at the end of growth. Likely, sports activities with repetitive flexion-internal rotation movements, especially with a high rate of loading, will (literally) have the most impact.
Overview
In reviewing evolution of the hip and pelvis we found extensive changes occurred many times in the epochs preceding emergence of the apes. In the last 300 my, these changes were certainly more profound, and more frequent, than those seen in the knee. In the very recent evolution of ape to human, the peculiar combination of a fundamental change in gait type and spectacular increase in brain size can be followed closely in the profound changes in the pelvis and lumbosacral spine. These changes enabled a double extension of pelvis and hip to produce an efficient bipedal gait. In turn, pelvic changes influenced the development of two stereotype hips for males and females, coxa recta and coxa profunda, both associated with development of osteoarthrosis through femoroacetabular impingement. These stereotypes represent a major portion of the hip arthroplasty workload for orthopaedic surgeons. But clearly, considerable variation in pelvis and hip types exists and will remain, exemplified by extraordinary “outliers” as illustrated in .
Discussion
Although the 19th century palaeontologist Dollo stated “An organism is unable to return, even partially, to a previous stage already realized in the ranks of its ancestors” (Gould 1970), we found several such “reversals” in evolution of the hip and pelvis. The most illustrative is perhaps the lumbar spine, where a mammal “long” spine evolved to a “short” ape spine, which then reverted to a “long” human lumbar spine ().
The development of the male coxa recta also may appear a reversal to an “earlier” more cursorial hip type, and development of its aspherical head would appear a relapse, because aspherical heads are associated with osteoarthrosis and spherical heads less so (Hoaglund and Low Citation1980, Kim Citation1989). However, it is unlikely osteoarthrosis ever was an important evolutionary driver in the development of the hip and pelvis. Osteoarthrosis has been observed in Neanderthal fossils (Trinkaus 1985), but generally, hip joint degeneration is a disease of older age. In humans, a coxa profunda female hip usually produces osteoarthrosis in the 6th decade of life – it is not a disease of mothers, but of grandmothers. In the coxa recta male hip, problems may start earlier, in the 4th or 5th decade, but in evolutionary terms, this implies a male with offspring that already may be a grandfather. Selection for a hip type likely was more influenced by factors as fast or long running, or maximum power, than ability to withstand loading without degeneration for over 40 years.
The “evolutionary reversals” appear a waste of time, generations and energy, as evolution could have followed a more “direct” path to a given trait. But evolution doesn't plan, think or want anything, it can only build or adapt bodies with the materials of the past, the DNA of the parents.
Acknowledgement
The authors thank Mr. Ad Hupkes, photographer, for his valuable help with preparing illustrations of the Naturalis specimens.
References
- Abitbol MM. Effect of posture and locomotion on energy expenditure. Am J Phys Anthropol 1988; 77(2)191–9
- Abitbol MM. Evolution of the ischial spine and of the pelvic floor in the Hominoidea. Am J Phys Anthropol 1988; 75(1)53–67
- Ahlberg PE, Clack JA, Blom H. The axial skeleton of the Devonian tetrapod Ichthyostega. Nature 2005; 437(7055)137–40
- Aiello L, Dean C. An Introduction to Human Evolutionary Anatomy. Elsevier Academic Press, New York 2002
- Alvarez W.T. Rex and the Crater of Doom. Penguin Press Science, London 1998
- Asfaw B. Proximal femur articulation in Pliocene hominids. Am J Phys Anthropol 1985; 68(4)535–8
- Bardakos NV, Villar RN. Predictors of progression of osteoarthritis in femoroacetabular impingement: a radiological study with a minimum of ten years follow-up. J Bone Joint Surg Br 2009; 91(2)162–9
- Beck M, Leunig M, Parvizi J, Boutier V, Wyss D, Ganz R. Anterior femoroacetabular impingement: part II. Midterm results of surgical treatment. Clin Orthop Relat Res 2004, 418: 67–73
- Bergé C. L'evolution de la hanche et du pelvis des Hominidés. CNRS, Paris 1993
- Bergmann G, Graichen F, Rohlmann A. Hip joint loading during walking and running, measured in two patients. J Biomech 1993; 26(8)969–90
- Bramble DM, Lieberman DE. Endurance running and the evolution of Homo. Nature 2004; 432(7015)345–52
- Brunet M, Guy F, Pilbeam D, Mackaye HT, Likius A, Ahounta D, et al. A new hominid from the Upper Miocene of Chad, Central Africa. Nature 2002; 418(6894)145–51
- Carey TS, Crompton RH. The metabolic costs of ‘bent-hip, bent-knee’ walking in humans. J Hum Evol 2005; 48(1)25–44
- Carrier DR. The evolution of locomotor stamina in tetrapods: circumventing a mechanical constraint. Paleobiology 1987; 13: 326–41
- Carroll SB. Endless Forms, Most Beautifull. The new science of Evo-Devo. W. W. Norton & Company, New York 2005
- Carter DR, Wong M. The role of mechanical loading histories in the development of diarthrodial joints. J Orthop Res 1988; 6(6)804–16
- Clack JA. Gaining Ground: The Origin and Evolution of Tetrapods. Indiana University Press, Bloomington 2002
- Daeschler EB, Shubin NH, Jenkins FA, Jr. A Devonian tetrapod-like fish and the evolution of the tetrapod body plan. Nature 2006; 440(7085)757–63
- Darwin C. The Descent of Man, and Selection in Relation to Sex. 1st ed. John Murray, London 1871
- Davis MC, Dahn RD, Shubin NH. An autopodial-like pattern of Hox expression in the fins of a basal actinopterygian fish. Nature 2007; 447(7143)473–6
- Dawkins R. Climbing Mount Improbable. Viking, London 1996
- Dawkins R. The Ancestor's tale. A pilgrimage to the dawn of evolution. Weidenfeld & Nicholson, London 2004
- Dubois E. Sur le rapport de l'encéphale avec la grandeur du corps chez les Mammifères. Bull Soc Anthropol Paris. 1897; 8: 337–74
- Dye SF. Functional morphologic features of the human knee: an evolutionary perspective. Clin Orthop Relat Res 2003, 410: 19–24
- Ecker TM, Tannast M, Puls M, Siebenrock KA, Murphy SB. Pathomorphologic alterations predict presence or absence of hip osteoarthrosis. Clin Orthop Relat Res 2007, 465: 46–52
- Ezoe M, Naito M, Inoue T. The prevalence of acetabular retroversion among various disorders of the hip. J Bone Joint Surg Am 2006; 88(2)372–9
- Faulkner KG, Wacker WK, Barden HS, Simonelli C, Burke PK, Ragi S, et al. Femur strength index predicts hip fracture independent of bone density and hip axis length. Osteoporos Int 2006; 17(4)593–9
- Galik K, Senut B, Pickford M, Gommery D, Treil J, Kuperavage AJ, et al. External and internal morphology of the BAR 1002'00 Orrorin tugenensis femur. Science 2004; 305(5689)1450–3
- Ganz R, Leunig M, Leunig-Ganz K, Harris WH. The etiology of osteoarthritis of the hip: an integrated mechanical concept. Clin Orthop Relat Res 2008, 466: 264–72
- Gibbons A. The First Human. The race to discover our earliest ancestors. Anchor Books, New York 2006
- Gibbons A. Anthropology. The birth of childhood. Science 2008; 322(5904)1040–3
- Giori NJ, Trousdale RT. Acetabular retroversion is associated with osteoarthritis of the hip. Clin Orthop Relat Res 2003, 417: 263–9
- Gosvig KK, Jacobsen S, Palm H, Sonne-Holm S, Magnusson E. A new radiological index for assessing asphericity of the femoral head in cam impingement. J Bone Joint Surg Br 2007; 89(10)1309–16
- Gosvig KK, Jacobsen S, Sonne-Holm S, Gebuhr P. The prevalence of cam-type deformity of the hip joint: a survey of 4151 subjects of the Copenhagen Osteoarthritis Study. Acta Radiol 2008; 49(4)436–41
- Harris WH. Etiology of osteoarthritis of the hip. Clin Orthop Relat Res 1986, 213: 20–33
- Hoaglund FT, Low WD. Anatomy of the femoral neck and head, with comparative data from Caucasians and Hong Kong Chinese. Clin Orthop Relat Res 1980, 152: 10–6
- Hutchinson JR, Gatesy SM. Adductors, abductors, and the evolution of archosaut locomotion. Paleobiology 2000; 26: 734–51
- Hutchinson JR, Gatesy SM. Dinosaur locomotion: beyond the bones. Nature 2006; 440(7082)292–4
- Jarvik E. The Devonian Tetrapod Ichtyostega. Fossils and Strata. 1996; 40(April)1–213
- Jenkins FA, Jr. Chimpanzee bipedalism: cineradiographic analysis and implications for the evolution of gait. Science 1972; 178(63)877–9
- Jenkins FA. Carnivore hip structure and locomotion. J Zool Lond 1977; 181: 351–70
- Jenkins FA. The Postcranial Skeleton of African Cynodonts. Bull Peabody Mus Nat Hist 1971; 36: 157–216
- Jerison HJ. Evolution of the Brain and Intelligence. Academic Press, New York 1973
- Johanson D, Elgar B. From Lucy to Language. Cassell, London 2001
- Jüni P. Personal communication: Hip impingement in Swiss army recruits. Bern. 2009
- Kalberer F, Sierra RJ, Madan SS, Ganz R, Leunig M. Ischial spine projection into the pelvis : a new sign for acetabular retroversion. Clin Orthop Relat Res 2008, 466: 677–83
- Kalmey JK, Lovejoy CO. Collagen fiber orientation in the femoral necks of apes and humans: do their histological structures reflect differences in locomotor loading?. Bone Aug, 2002; 31(2)327–32
- Kemp TS. The Origin and Evolution of Mammals. Oxford Biology, Oxford 2004
- Kermack KA, Mussett F, Rigney HW. The skull of Morganucodon. Zoological Journal of the Linnean Society. 1981; 71(1)1–158
- Kim YH. Relationship between the sphericity of femoral head-acetabulum and the low incidence of primary osteoarthritis of the hip joint in Koreans. Yonsei Med J 1989; 30(3)280–7
- Kingdon J. Lowly origins: where, when and why our ancestors first stood up. Princeton University Press, Prinecton / /Oxford 2003
- Leunig M, Beck M, Woo A, Dora C, Kerboull M, Ganz R. Acetabular rim degeneration: a constant finding in the aged hip. Clin Orthop Relat Res 2003, 413: 201–7
- Lovejoy CO, Meindl RS, Ohman JC, Heiple KG, White TD. The Maka femur and its bearing on the antiquity of human walking: applying contemporary concepts of morphogenesis to the human fossil record. Am J Phys Anthropol 2002; 119(2)97–133
- Lovejoy CO. Evolution of human walking. Sci Am 1988; 259(5)118–25
- Lovejoy CO. The natural history of human gait and posture. Part 1. Spine and pelvis. Gait Posture 2005; 21(1)95–112
- Lovejoy CO. The natural history of human gait and posture. Part 2. Hip and thigh. Gait Posture 2005; 21(1)113–24
- Lovejoy CO. The origin of man. Science 1981; 211(4480)341–50
- Martin R, Saller K. Lehrbuch der Anthropologie. Band II. Gustav Fischer Verlag, Stuttgart 1959
- Maruyama M, Feinberg JR, Capello WN, D'Antonio JA. The Frank Stinchfield Award: Morphologic features of the acetabulum and femur: anteversion angle and implant positioning. Clin Orthop Relat Res 2001, 393: 52–65
- Miller G. The Mating Mind: how sexual choice shaped the evolution of human nature. Heinemann, London 2000
- Morgan E. The aquatic ape hypothesis. Souvenir Press, London 1997
- Murphy SB, Ganz R, Muller ME. The prognosis in untreated dysplasia of the hip. A study of radiographic factors that predict the outcome. J Bone Joint Surg Am 1995; 77(7)985–9
- Murray RO. The aetiology of primary osteoarthritis of the hip. Br J Radiol 1965; 38(455)810–24
- Murtha PE, Hafez MA, Jaramaz B, DiGioia AM. Variations in acetabular anatomy with reference to total hip replacement. J Bone Joint Surg Br 2008; 90(3)308–13
- Müller W. Das Knie. Form, Funktion und ligamentäre Wiederherstellungschirurgie. Springer Verlag, Berlin 1983
- Notzli HP, Wyss TF, Stoecklin CH, Schmid MR, Treiber K, Hodler J. The contour of the femoral head-neck junction as a predictor for the risk of anterior impingement. J Bone Joint Surg Br 2002; 84(4)556–60
- Ohman JC, Krochta TJ, Lovejoy CO, Mensforth RP, Latimer B. Cortical bone distribution in the femoral neck of hominoids: implications for the locomotion of Australopithecus afarensis. Am J Phys Anthropol 1997; 104(1)117–31
- Ohman JC, Lovejoy CO, White TD, Eckhardt RB, Galik K, Kuperavage AJ. Questions About Orrorin Femur. Science 2005; 307(5711)845b
- Prendergast PJ, Huiskes R. The biomechanics of Wolff's law: recent advances. Ir J Med Sci 1995; 164(2)152–4
- Preuschoft H. Mechanisms for the acquisition of habitual bipedality: are there biomechanical reasons for the acquisition of upright bipedal posture?. J Anat 2004; 204(5)363–84
- Raup DM, Sepkoski JJ, Jr. Periodic extinction of families and genera. Science 1986; 231: 833–6
- Roach KE, Miles TP. Normal hip and knee active range of motion: the relationship to age. Phys Ther 1991; 71(9)656–65
- Romer AS, Price LI. Review of the Pelycosauria. Geological Society of America Special Paper 1940; 28: 1–538
- Ruelle M, Dubois JL. La malformation protrusive et sa complication arthrosique. I.- Symptomatologie radiologique et clinique. - éthiopathogénie. [The protrusive malformation and its arthrosic complication. I. Radiological and clinical symptoms. Etiopathogenesis.]. Rev Rhum Mal Osteoartic 1962; 29: 476–89
- Schultz AH. Observations on the acetabulum of primates. Folia Primatol 1969; 11: 181–99
- Schultz AH. The physical distinctions of Man. Proc Am Philos Soc 1950; 94(5)428–99
- Serrat MA, Reno PL, McCollum MA, Meindl RS, Lovejoy CO. Variation in mammalian proximal femoral development: comparative analysis of two distinct ossification patterns. J Anat 2007; 210(3)249–58
- Shefelbine SJ, Carter DR. Mechanobiological predictions of growth front morphology in developmental hip dysplasia. J Orthop Res 2004; 22(2)346–52
- Shubin NH, Daeschler EB, Jenkins FA, Jr. The pectoral fin of Tiktaalik roseae and the origin of the tetrapod limb. Nature 2006; 440(7085)764–71
- Shubin NH. Your Inner Fish: a Journey into the 3.5-Billion-Year History of the Human Body. Pantheon, New York 2008
- Sibley CG, Ahlquist JE. DNA hybridization evidence of hominoid phylogeny: results from an expanded data set. J Mol Evol 1987; 26(1-2)99–121
- Smith-Petersen MN. The classic: Treatment of malum coxae senilis, old slipped upper femoral epiphysis, intrapelvic protrusion of the acetabulum, and coxa plana by means of acetabuloplasty. 1936. Clin Orthop Relat Res 2009, 467: 608–15
- Solomon L. Patterns of osteoarthritis of the hip. J Bone Joint Surg Br 1976; 58(2)176–83
- Stem ES, O'Connor MI, Kransdorf MJ, Crook J. Computed tomography analysis of acetabular anteversion and abduction. Skeletal Radiol 2006; 35(6)385–9
- Stulberg SD, Cordell L.D., Harris W.H. Unrecognized Childhood Hip Disease: A Major Cause of Idiopathic Osteoarthrosis of the Hip. The Hip Proceedings of the Third Meeting of The Hip Society. (Ed Amstutz HC). C.V. Mosby Company, Saint Louis 1975; 212–28
- Tague RG, Lovejoy CO. The Obstetric pelvis of A.L. 288-1 (Lucy). J Hum Evol 1986; 15: 237–55
- Taylor CR, Rowntree VJ. Running on two or on four legs: which consumes more energy?. Science 1973; 179(69)186–7
- Tayton E. Femoral anteversion: a necessary angle or an evolutionary vestige?. J Bone Joint Surg Br 2007; 89(10)1283–8
- Thompson PM, Giedd JN, Woods R, MacDonald D, Evans AC, Toga AW. Growth patterns in the developing brain detected by using continuum mechanical tensor maps. Nature 2000; 404(6774)190–3
- Thorpe SK, Holder RL, Crompton RH. Origin of human bipedalism as an adaptation for locomotion on flexible branches. Science 2007; 316(5829)1328–31
- Toogood PA, Skalak A, Cooperman DR. Proximal femoral anatomy in the normal human population. Clin Orthop Relat Res 2009, 467: 876–85
- Trevathan WR. The evolution of bipedalism and assisted birth. Medical Anthropol Quarterly 1996; 10(2)287–90
- Tucker AS, Watson RP, Lettice LA, Yamada G, Hill RE. Bapx1 regulates patterning in the middle ear: altered regulatory role in the transition from the proximal jaw during vertebrate evolution. Development 2004; 131(6)1235–45
- Walker AD. Evolution of the pelvis in birds and dinosaurs. Problems in vertebrate evolution. 1977; 319–58
- Weiner LS, Kelley MA, Ulin RI, Wallach D. Development of the acetabulum and hip: computed tomography analysis of the axial plane. J Pediatr Orthop 1993; 13(4)421–5
- Wiberg G. The anatomy and roentgenographic appearance of a normal hip joint. Acta Chir Scand Suppl 1939; 83: 7–38
- Videan EN, McGrew WC. Bipedality in chimpanzee (Pan troglodytes) and bonobo (Pan paniscus): testing hypotheses on the evolution of bipedalism. Am J Phys Anthropol 2002; 118(2)184–90
- Wolpert L. Principles of Development. Oxford University Press, Oxford 2006
- Zimmer EA. Borderlands of the Normal and Early Pathologic in Skeletal Roentgenology. Grune and Stratton, New York 1968