Abstract
In our study, a novel hepatocyte-targeting norcantharidin (NCTD) derivative lactosyl-norcantharidin (Lac-NCTD) was synthesised using ethanediamine as a truss arm, and lactosyl-norcantharidin nanoparticles (Lac-NCTD-NPs) were obtained by an ionic cross-linkage process with an entrapment efficiency of (80.29 ± 0.56)%. The release of Lac-NCTD-NPs in vitro was then investigated by means of a dialysis method, and its sustained effect was evident. The in vitro anti-tumour activities of Lac-NCTD and Lac-NCTD-NPs were studied by their cytotoxic effects on HepG2, SMMC-7721 and SGC-7901 cells. The results showed that the IC50 values of Lac-NCTD and Lac-NCTD-NPs cytotoxicity against HepG2 and SMMC-7721 cells were lower compared to NCTD, and the anti-tumour effects could be remarkably inhibited by galactosylated fetal bovine serum (Gal-FBS) as a competitor of the asialoglycoprotein receptor. The cytotoxic effects against SGC-7901 cells were relatively high, even higher than NCTD, and Gal-FBS had no influence on them at all. The amount of Lac-NCTD accumulated in SMMC-7721 cells was assayed by high-performance liquid chromatography, which indicated that Lac-NCTD may be able to permeate the cell membrane in its unchanged form. The anti-tumour activities of Lac-NCTD and Lac-NCTD-NPs in vivo were evaluated in mice bearing an H22 tumour. The results suggested that tumour growth was effectively inhibited by all treatments, including NCTD, Lac-NCTD and Lac-NCTD-NPs, with Lac-NCTD-NPs being the most effective, followed by Lac-NCTD. As a result, Lac-NCTD-NPs may be regarded as liver-targeting agents, which combine both active and passive targeting.
1. Introduction
Norcantharidin (NCTD) is synthesised from cantharidin, an active constituent obtained from the dried body of the Chinese blister beetle (Mylabris) used as a Chinese traditional medicine (CTM), traced back to over 2000 years Citation1,Citation2. The powerful activity of NCTD against hepatoblastoma cells has been demonstrated in vitro and in vivo, but its therapeutic applications are limited because of severe side effects, including the significant drawback of irritating the urinary organs in previous clinical trials Citation3,Citation4. In order to minimise its toxicity, several alternative carriers such as microspheres, microemulsions, liposomes and nanoparticles have been designed to improve the liver-targeting properties of NCTD Citation5. However, increasing the entrapment efficiency of the drug in formulations is a challenge, because of the powerful solubility of norcantharidin acid forms in water Citation6,Citation7.
The asialoglycoprotein receptor (ASGP-R), situated on the hepatocyte membrane, is able to recognise galactose or N-acetylgalactosamine residues of desialylated glycoproteins Citation8,Citation9. Its main function is to maintain serum glycoprotein homeostasis by the recognition, binding and endocytosis of asialoglycoproteins (ASGPs), that is, desialylated glycoproteins with terminal galactose or GalNAc residues. After internalisation via clathrin-coated pits and their fusion with endosomes, the ASGPs are released in the acidic environment of the endosome and transported to lysosomes for degradation, while the receptor is recycled back to the cell surface Citation10. For these properties, ASGP-R has been exploited as a liver-specific targeting marker for gene and drug vehicles Citation11–13. Galactose was also used as a ligand of hepatocyte-specific targeted agents, including various formulations and prodrugs Citation14–17.
The formulation of nanoparticles is known to be an efficient approach to improving the oral bioavailability of some poorly bioavailable drugs and protecting incorporated drug molecules from gastrointestinal tract degradation as well as gut wall metabolism. Simultaneously, drugs entrapped by nanoparticles are protected from enzymes metabolism during blood transport. On the other hand, the nanoparticles are subject to passive target preparation and transport through the Peyer's paths in the gastrointestinal tract. It has been reported that nanoparticles injected intravenously are taken up by the liver after only a few minutes due to the opsonisation process. The characteristic of passive targeting of nanoparticles has been confirmed to be related to size in previous studies Citation18. When the diameter of the nanoparticle is less than 200 nm, it can be captured by the reticuloendothelial system, especially by Kupffer cells in the liver.
To obtain higher selectivity and enhance the uptake of target cells, a novel nanoparticle was prepared that integrates both active targeting, by ligand binding to the ASGP-R, and its own passive targeting function Citation19. Considering the water solubility of the drug, it was decided to modify NCTD with a lactobiose-bearing galactose group, rather than the nanoparticle carrier. The emphasis of this study was on the combination of active and passive targeting for the selected substance, NCTD.
2. Materials and method
2.1. Materials
NCTD was purchased from Surui medicine chemical Co. Ltd (Suzhou, China). Chitosan (CS), with the deacetylation of 90.9% and the molecular weight of 8–10 kDa, was supplied by Xingcheng Biochemical Co. Ltd (Nantong, China). Sodium tripolyphosphate (TPP) was obtained from National Drug Group (Shanghai, China). 3-(4,5-dimethylthiazd-2-yl)-2,5-diphenyltentrazolium bromide (MTT), fetal bovine serum (FBS) and RPMI-1640 medium were purchased from Bipec Biopharma Corporation (Nanjin, China). Galactosylated fetal bovine serum (Gal-FBS) was purchased from Sigma Chemical Company (Shanghai, China). Other chemicals and solvents were of analytical grades.
2.2. Synthesis of lactosyl-norcantharidin
The lactonolactone was synthesised as described previously Citation20,Citation21. Briefly, a solution of lactobionic acid sodium was passed through a cation-exchange resin column (732) to convert it to free lactobionic acid. The eluted free acid was vacuum dried. Lactobionic acid (25 g, 69.77 mmol) was dissolved in 130 mL of 2-methoxyethanol. The solution was heated and stirred, and then the solution was diluted with 65 mL of toluene, and concentrated. When the temperature of distilled solution rose up to the boiling point of 2-methoxyethanol, addition of 130 mL 2-methoxyethanol and 65 mL of toluene, followed by concentration, was repeated two times. Finally, after cooling the solution, crystalline lactonolactone was collected by filtration, and washed with cool methanol, yield 11.88 g (35.05 mmol, 50.23%), mp:198–199°C; Max (kBr)/cm−1 : 1716.
NCTD-mono-amides was synthesised, referring to the procedure reported by Mantovani G with slightly modified Citation22. Briefly, the NCTD (2.00 g, 12.0 mmol) was suspended in methanol (50 mL) and the mixture cooled to 0°C A solution of ethanolamine (0.8 mL, 12.0 mmol) in 20 mL of methanol was added dropwise (10 min), and the resulting solution was stirred for 5 min at 0°C, then 6 h at ambient temperature, and finally standed for 24 h. The sedimentum was removed by filtrate and the filter liquor was purified by passing through silica gel column (acetic ether:methanol = 4 : 1, Rf ≈ 0.5) to give 1.86 g (8.9 mmol; 73.89%) NCTD-mono-amides.
The lactonolactone was dissolved in 20 mL methanol, heated and stirred, and then 0.841 g (4 mmol) NCTD-mono-amides was added. The mixture solution was heated and stirred at 80°C for 4 h; the white sedimentum was collected by filtration, washed with cool methanol, and then vacuum dried to yield 1.934 g (3.7 mmol; 92.54%) Lacto-NCTD. Mp:191–192°C; TLC:Rf ≈ 0.7 (ether : methanol = 1 : 1) and Rf ≈ 0.6 (n-butanol : acetic acid : water = 2 : 1 : 1).
The chemical structure was confirmed by IR, 1H-NMR, 13C-NMR, and EMS-IR (v/cm−1) methods: 3352.2 (broad peak, –OH), 1696 (singlet, –C=O); 1H-NMR (400 MHz, DMSO), δ(ppm):1.619 (4H, s, 1-H); 2.982 (2H, q, J = 7.2, 3-H); 3.146 (1H, t, J = 6.8, 5-H); 3.236 (1H, t, J = 6.4, 5-H); 3.321 (4H, m, 12-H, 15-H, 10-2H); 3.399 (2H, t, J = 4.4, 4-H); 3.491 (2H, t, J = 5.6, 16-H); 3.579 (2H, m, 13-H, 14-H); 3.672 (2H, m, 8-H, 9-H); 3.961 (1H, s, 7-H); 4.055 (1H, d, J = 4.4, 6-H); 4.255 (1H, d, J = 6.4, 11-H); and 4.663 (2H, s, 2-H); 13C-NMR (DMSO), δ(ppm): 33.638 (1-C); 83.934 (2-C); 55.124 (3-C); 182.903 (a-C); 43.382 (4-C); 41.678 (5-C); 178.185 (b-C); 77.461 (6-C); 75.934 (7-C); 88.434 (8-C); 73.938 (9-C); 66.232 (10-C); 110.115 (11-C); 78.825 (12-C); 76.938 (13-C); 76.920 (14-C); 81.264 (15-C);and 67.937 (16-C); EMS:550.10.
2.3. Preparation of lactosyl-norcantharidin nanoparticles
Lactosyl-norcantharidin nanoparticles (Lac-NCTD-NPs) were prepared according to the procedure first reported by Calvo et al. Citation23, which is based on the ionic gel of CS with TPP anions. Briefly, 0.1 g CS was dissolved in 50 mL acetic acid aqueous solution and then 0.1 g lactosyl-norcantharidin (Lac-NCTD) was added under magnetic stirring (500 rpm) at 40°C. When almost dissolved, 20 mL of TPP (1.2 mg mL−1) solution was added to the CS solution drop by drop, resulting in cross-linkage. Finally, an opalescent suspension (Lac-NCTD-NPs) was obtained.
2.4. Evaluation of Lac-NCTD-NPs
A freshly prepared suspension of Lac-NCTD-NPs was filtered through a 0.45 µm filter (Shanghai Xinya Cleaning Materials Co., Chian) to remove insoluble polymer residues. Then, the drug entrapment efficiency and loading capacity of Lac-NCTD-NPs were determined as previously described Citation24. Briefly, 5 mL of filtered suspension was ultracentrifuged (250,000 × g for 30 min at 10°C) in an Optima MAX Centrifuge (Berkman Co. Ltd., USA). The supernatant was sampled, and the concentration of Lac-NCTD in the filtered suspension and in the supernatant were estimated using a reversed-phase high-performance liquid chromatography (HPLC) method.
The HPLC system consisted of two pumps (LC-10AT VP, Shimadzu, Japan) and a UV-Vis detector (SPD-M10A VP, Shimadzu, Japan) set at 220 nm. The chromatographic column used was a Hypersil ODS2 (5 µm in 4.6 × 250 mm, Elite, Dalian, China) thermostated at 25°C. The mobile phase consisted of acetonitrile/water (10 : 90, adjusted to pH 3.2 by adding phosphoric acid) and the flow rate was 0.8 mL min−1. The entrapment efficiency (EE%) and loading capacity (LC%) of Lac-NCTD in the nanoparticles were calculated as follows:
The analysis of particle size distribution was performed by light scattering (Malvern Instruments Ltd., UK). The nanoparticle colloids were diluted 10-fold with ultrapure water, with the wavelength and temperature set at 670 nm and 25°C, respectively.
The morphological characteristics of the nanoparticles were studied by transmission electron microscopy (TEM; H600, Hitachi, Japan). Lac-NCTD-NPs were dropped onto the copper screen and stained with a 2% phosphotungstic acid solution before analysis.
2.5. In vitro release studies
The release of Lac-NCTD from nanoparticles in vitro was performed using a dialysis method. A 5 mL suspension of Lac-NCTD-NPs, or Lac-NCTD with concentration of 2 mg mL−1, was placed into a dialysis bag (MW cutoff 8000–14,000) sealed at both ends with clips. The bag was then placed into a beaker filled with 50 mL of the medium containing 0.1 mol L−1HCl, physiologic saline, PBS (pH = 6.0), PBS (pH = 7.4), and a cell culture medium of 10% FBS (RPMI-1640 10% FBS). The suspensions were incubated in a shaker at 37°C for 48 h, at a speed of 100 rpm. Aliquots were drawn from the beaker and replaced with equal volumes of the medium at different intervals. The amount of Lac-NCTD in the sample solutions was determined by HPLC.
2.6. Preparation of cells and animals
The human hepatoblastoma cell lines HepG2 and SMMC-7721, and a gastric cancer cell line SGC-7901 (obtained from the Teaching and Research Section of Pharmacology, Soochow University) were maintained in the RPMI-1640 medium, supplemented with 10% FBS (V/V) heat-inactivated (56°C, 30 min), penicillin (100 U mL−1) and streptomycin (100 U mL−1) in a humidified incubator (5% CO2), at 37°C.
Male C57BL/6 mice (6–8 weeks old) ranging from 18 to 22 g were supplied by the Laboratory Animals Center, Soochow University. All animal experiments adhered to the principles of care and use of laboratory animals.
Mice bearing the H22 hepatoblastoma cell line (supplied by Fudan University, China) were established as follows: The H22 cells were reanimated and centrifuged, and the supernatant was then discarded. Finally, the cells were r-suspended in physiologic saline (PhyS) and injected s.c. into the mice for serial subcultivation.
2.7. In vitro anti-tumour activity assay
The in vitro anti-tumour activities of Lac-NCTD and Lac-NCTD-NPs were determined by their cytotoxicity on HepG2, SMMC-7721 and SGC-7901 cells with the MTT assay. The cells in their logarithmic growth were seeded at a density of 104 cells well−1 onto 96-well plates and exchanged for fresh medium, after being incubated for 24 h. The sterile samples (NCTD, Lac-NCTD and Lac-NCTD-NPs at a given concentration) were then treated, and the cells were further incubated for 12, 24, 48 and 72 h. To each well, 10 µL of PBS solution containing 5 mg mL−1 MTT was added, and then 100 µL containing 1% SDS after incubation for 4 h. When the formazan crystal was completely dissolved, the optical density (OD) was determined at 570 nm with a microplate reader (Model ELx808, BioTek Intruments). The viability of the treated cells was defined as: OD (570 nm) value of treated cells/OD (570 nm) of non-treated cells.
2.8. Inhibition effect of Gal-FBS
In order to investigate the liver cellular uptake mechanisms of Lac-NCTD and Lac-NCTD-NPs, various cancer cell lines, including HepG2, SMMC-7721 and SGC-7901 cells, were pretreated by the inhibitor Gal-FBS for 12 h. The levels of cytotoxicity of NCTD, Lac-NCTD and Lac-NCTD-NPs against the above cell lines were then determined by the MTT assay method.
2.9. Determination of Lac-NCTD accumulated in SMMC-7721 cells by HPLC
The amount of Lac-NCTD accumulated in the SMMC-7721 cells was determined by the HPLC method under the same chromatographic conditions, as described in Section 2.4. The linear range, recovery and precision of the method were investigated as follows: 10 mg Lac-NCTD was weighed and diluted with PBS at different multiples to obtain serial control aliquot solutions. The SMMC-7721 cells were cultured in a RPMI-1640 medium, harvested in their exponential phase, and then scraped off. After being washed with PBS twice, the cells were homogenated to produce a blank cell homogenate. Serial control aliquot solutions were added into the blank cell homogenate respectively, vortexed rapidly for 2 min and then ultracentrifuged (3200 × g) for 15 min. The supernatant was sampled, and the concentration of Lac-NCTD in it was determined by a reversed-phase HPLC method. The linear equation was constructed by performing a regression analysis of the peak area (y) versus the concentration of Lac-NCTD (x). Finally, the recovery and precision of the method were determined by adding control aliquots of high, medium and low concentrations into the blank cell homogenate.
Firstly, the stability of Lac-NCTD in the cell culture medium was studied. A suitable concentration of Lac-NCTD was dissolved in a RPMI-1640 medium, and then incubated in a humidified incubator (5% CO2), at 37°C for 6 days. The solution was ultracentrifuged (3200 × g) for 15 min and the supernatant was then assayed by HPLC.
Finally, the amount of Lac-NCTD accumulated in the SMMC-7721 cells was obtained according to the reported method Citation25. The SMMC-7721 cells were seeded at the density of 106cells/well onto 6-well plates and incubated for 24 h, then exchanged for fresh medium. The solution of Lac-NCTD was added to give the final concentration with 100 µg mL−1. The cells were further incubated for 12 h and scraped off after the medium was removed. After being washed with PBS twice, a known number cells in PBS were homogenated and ultracentrifuged for 15 min. The accumulated amount of Lac-NCTD in the SMMC-7721 cells in the supernatant was assayed by HPLC.
2.10. In vivo anti-tumour studies
After serial subcultivation of the H22 cells for 7 days, the mice with superior ascites tumours were selected and sacrificed by cervical dislocation under sterile conditions. The ascites was drawn and diluted with physiologic saline to modulate the cell density at l × 107 mL−1. The ascites tumour cells were injected s.c. into the right rear legs of mice at a dose of approx. 0.1 mL 10 g−1, and the mice were then divided at random into eight different experimental groups, including a control group (CG, physiologic saline) and medication administration team (MAT), including NCTD, Lac-NCTD high dose, Lac-NCTD medium dose, Lac-NCTD low dose, Lac-NCTD-NPs high dose, Lac-NCTD-NPs medium dose and Lac-NCTD-NPs low dose. On the second day after inoculation, the mice were given an i.p. injection of various samples daily for 8 days, and tumour size was measured every day with a caliper in two dimensions. Individual tumour volumes (V) were calculated by the formula: V = [length × (width)2]/2. The mice were weighed before being sacrificed the day after administration was terminated. The subcutaneous tumour, thymus, and spleen were carefully removed and weighed to obtain the indexes as follows: inhibition rate on tumour weight = [1 – (tumour weight of MAT/tumour weight of CG)] × 100%; organ coefficient = (organ weight/body weight) × l00%.
3. Results and discussion
3.1. Synthesis of Lac-NCTD
Ethanediamine was chosen as the truss arm to conjugate NCTD and lactonolactone to produce a novel compound, Lac-NCTD (), because of its two active terminal amine groups, which render the reaction well controlled and high yielding. Furthermore, the conversion reaction from lactobionic acid into lactones is a crucial step in obtaining Lac-NCTD with a higher yield, since the carboxyl group of lactobionic acid lacks reactivity to amines, even in the presence of catalysts such as carbodiimides Citation26. The purity of the Lac-NCTD was above 98.34% without further purification. However, in order to get the control aliquots, the crude product was effectively separated and purified with preparative liquid chromatography by adding acetic acid to adjust the pH of the aqueous phase. As a result, a hypso-yielded Lac-NCTD was attained, which could be prepared on a large scale, and also hypso-purified for use as control aliquots in the following experiments.
3.2. Evaluation of Lac-NCTD-NPs
The entrapment efficiency and loading capacity of Lac-NCTD-NPs were (80.29 ± 0.56)% and (9.58 ± 0.09)%, respectively. The entrapment efficiency was significantly higher than that of NCTD-NPs, which was only (54.64 ± 3.71)%. The much higher entrapment efficiency of Lac-NCTD-NPs may result from the large hydroxy group on the new chemical (Lac-NCTD) combined with the positive charge of CS due to intermolecular hydrogen bonds forming nanoparticles in which Lac-NCTD was adsorbed and encapsulated, while NCTD-NPs were formed only by encapsulation of NCTD in the nanoparticles. Moreover, the acid form of NCTD dissolved in water could easily be dispersed.
The mean particle size and polydispersity index (PI) of the prepared nanoparticles were about 100 nm and 0.168 nm, respectively, as shown in . It has been reported that the majority of fenestrated liver sinusoids are usually smaller than 200 nm in diameter Citation27. Additionally, tumour blood vessels present several abnormalities in comparison with physiologically normal vessels, often including a relatively high proportion of proliferation in vivo, an increased tortuosity, an aberrant basement membrane formation and enhanced permeability Citation28–30. Thus, the Lac-NCTD-NPs prepared can pass through the fenestrated sinusoids and accumulate in the tumour sites of the liver.
The morphological properties of the nanoparticles are shown in . Most of the nanoparticles appear uniform and round under TEM.
3.3. Drug release of Lac-NCTD-NPs in vitro
The results of drug release experiments in vitro are shown in . The release plot of Lac-NCTD from Lac-NCTD-NPs in various media showed no pronounced differences, and the effective sustained drug release lasted as long as 48 h. The release plot shows the representative character of nanoparticles as ‘sudden-slow-moving releasing’, while the native material has released almost 100% in the first 12 h. However, the drug release in the cell culture medium containing 10% FBS (RPMI-1640 10% FBS) was the slowest, not only for the nanoparticles but also for the native material solution, which may be slightly inhibited by the plasma proteins in the cell culture medium.
Figure 4.
In vitro release of Lac-NCTD from nanoparticles in various mediums compared to Lac-NCTD.








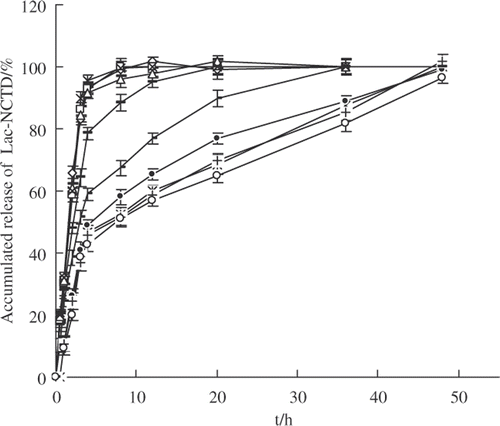
3.4. Cytotoxicity assay
The levels of cytotoxicity of Lac-NCTD on HepG2, SMMC-7721 and SGC-7901 cells are summarised in . The data indicate that the levels of cytotoxicity of Lac-NCTD on the three kinds of cells are all dose- and time-dependent. In addition, in order to compare the anti-tumour potency of Lac-NCTD, Lac-NCTD-NPs and NCTD, the IC50 values of each formulation were calculated in units of mmol L−1 (). The data suggest that the IC50 values of Lac-NCTD against HepG2 and SMMC-7721 cells are significantly lower than that of NCTD and Lac-NCTD-NPs when incubated for 24 h. When incubated for 48 h, the IC50 of Lac-NCTD-NPs was the lowest of all, followed by Lac-NCTD. In contrast, the IC50 values of Lac-NCTD against SGC-7901 cells were no lower than that of NCTD, and even higher as incubation time increased. As a result, Lac-NCTD had a much stronger therapeutic effect on HepG2 and SMMC-7721 cells than NCTD when applied at the same mole dosage in vitro. As for Lac-NCTD-NPs, their activity was significantly weaker than Lac-NCTD when incubated for 24 h, even weaker than NCTD, but when incubated for 48 h, they showed the strongest activity. The inhibiting effect of Gal-FBS, a competitor of ASGP-R, on the anti-tumour activity of Lac-NCTD was also investigated by assessing its influence on the IC50 of each formulation. The results () demonstrate that the activities of Lac-NCTD and Lac-NCTD-NPs on HepG2 and SMMC-7721 cells were significantly inhibited by Gal-FBS, but that of NCTD was not inhibited at all. However, the activities on SGC-7901 cells of all three formulations combined were not influenced by Gal-FBS.
Figure 5. Cytotoxic effects of Lacto-NCTD on various cell lines including (a) HepG2; (b) SMMC-7721; and (c) SGC-7901, at given time points: including 12 h


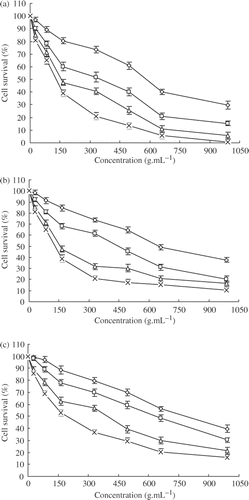
Figure 6. IC50 values of various formulations treated by two different mediums on various cell lines, including (a) HepG2; (b) SMMC-7721; and (c) SGC-7901; NCTD (RPMI-1640 10% FBS)




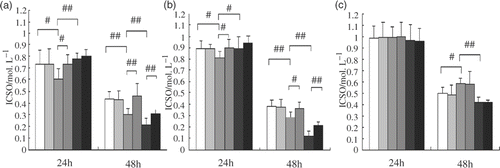
HepG2 and SMMC-7721 are both human hepatoblastoma cell lines that are known to express ASGP-R, while SGC-7901 is a gastric cancer cell line without ASGP-R Citation31,Citation32. The ASGP-R on the cell surface recognises the galactose residue of desialyated serum protein. The galactose residue is used for targeting drugs to liver parenchyma cells. Gal-FBS has been recognised as a specific ligand for ASGP-R with characteristics of high affinity for isolated liver cells and cultured hepatoblastoma cell lines. The effective functional oxo-bridge group in this study was still unchanged in the novel synthesised NCTD-derivate Citation33–35, so that its anti-tumour activity is not reduced at all. On the contrary, a higher potency has been shown, resulting from the galactose residue on the new compound, which aids Lac-NCTD recognition by ASGP-R on the surface of hepatoblastoma cell lines and enhances its rapid permeation into the cell membrane.
As for Lac-NCTD-NPs, their powerful in vitro anti-tumour activity may be induced by the innate characteristics of the nanoparticle, such as the cationic charge of CS, which is found to bind via electrostatic interactions to negatively charged phospholipid headgroups that are preferentially expressed on tumour cells Citation36. Additionally, the encapsulated Lac-NCTD was released slowly from the nanoparticles, preventing saturation of ASGP-R. The passive targeting properties of the nanoparticle should now be investigated in vivo.
3.5. Determination of Lac-NCTD accumulated in SMMC-7721 cells
As shown in , under the chromatographic conditions described in Section 2.4, the retention time of Lac-NCTD was about 5 min, the test of the active component was not confounded by impurities, and the method had a high specificity. The standard curve equation of Lac-NCTD was y = 5324 + 3468.9 x (r = 0.9991) at the linear concentration range from 10 to 1000 µg mL−1; the recovery and precision both fit the requirements of the analysis of the biological specimen.
Figure 7. Determination of Lac-NCTD accumulated in SMMC-7721 cells: (a) blank cell homogenate; (b) Lac-NCTD control aliquot reference substance; and (c) cell homogenate sample with Lac-NCTD; 1.Lac-NCTD.
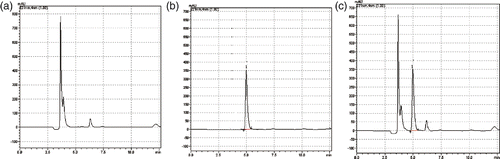
The stability of Lac-NCTD is shown in and its half-life were about 3.82 days. Although a little instability was shown in the RPMI-1640 medium in a humidified incubator (5% CO2) at 37°C, Lac-NCTD was still stable in the initial 12 h, during which we studied the accumulation of Lac-NCTD in SMMC-7721 cells, with almost 95% in its unchanged form. However, what type of products Lac-NCTD converts to needs further investigation.
Based on the result of the above study, the amount of Lac-NCTD accumulated in SMMC-7721 cells was 3.89 µg 106 cell−1 after treatment with 100 µg mL−1 Lac-NCTD for 12 h. The data presented here seem to indicate that Lac-NCTD partly permeated into the cell membrane in its unchanged form. But whether the unchanged form permeates the cell membranes through the ASGP-R pathway needs further research.
3.6. In vivo anti-tumour study
The tumour-inhibitory activities of Lac-NCTD and Lac-NCTD-NPs were evaluated in mice bearing H22 hepatoblastomas. The tumour growth curve () shows that all the formulations were effective in preventing tumour growth compared to the treatment with PhyS. Among the treatment groups, both Lac-NCTD and Lac-NCTD-NPs were more powerful against the tumours than NCTD, and Lac-NCTD-NPs were the most powerful of all Citation37,Citation38.
Figure 9. Tumour growth inhibition by various formulations containing the same mole of effective NCTD in H22-bearing mice (n = 10): PhyS



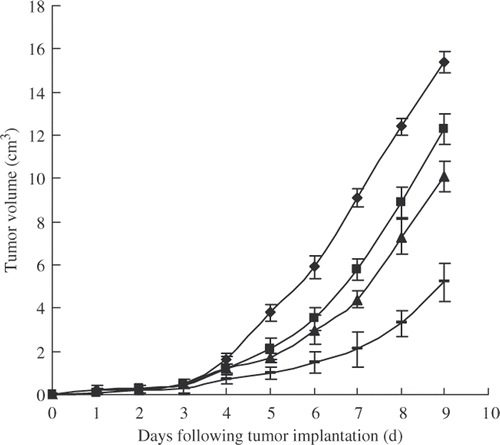
The inhibition rate on tumour weight in response to the above treatments was also determined. The results (data not shown) were in agreement with the tumour growth curve, which show that Lac-NCTD-loaded nanoparticles can produce a therapeutic improvement in liver tumours.
The organ coefficients of various formulations () were all higher than the control group (treated with PhyS), especially of Lac-NCTD-NPs, which was significantly higher than for the other groups. The results indicate that Lac-NCTD-NPs may be effective in preserving organs and enhancing immunity Citation39.
Table 1. Organ coefficients of various formulations in H22-bearing mice (n = 10).
The strong anti-tumour activity of Lac-NCTD-NPs, especially in vivo, may be explained by the fact that a far higher dose of free Lac-NCTD would need to be administered to reach the same drug concentration in the liver, because of its wider tissue distribution in vivo. It was suggested that the Lac-NCTD entrapped in nanoparticles administered in vivo may be able to passively accumulate in the liver due to their size (100 nm) without biodistribution to other tissues, such as the kidney, where NCTD tends to accumulate and cause renal toxicity. The nanoparticles that passed through the fenestrations in the hepatic sinusoid reached the hepatocytes, where the free Lac-NCTD could be released slowly from the nanoparticles to be recognised by ASGP-R on the surface of the hepatocytes by means of active targeting.
4. Conclusions
We have synthesised a novel liver-targeting NCTD derivative with a high yield. Additionally, the Lac-NCTD-NPs were prepared with a significantly high entrapment efficiency compared to NCTD-NPs, resulting from the new chemical constitution of Lac-NCTD. The results of investigations in vivo and in vitro suggest that the Lac-NCTD-NPs are an effective formulation which combine active and passive targeting. However, extensive investigation is still needed to assess the possible application of the new chemical and its nanoparticles in humans, and the mechanisms by which they target the liver.
Acknowledgements
This study was supported by National Key Technology R&D Program in the 11th Five year Plan of China (2006BAI09B00), the Innovation Fund of Medium or Small Science and Technology Enterprise by National Science and Technology Ministry (07C26223201333), Social Development Foundation of Jiangsu Province (BS2005022), ‘the Project of High New Technology Research’ from the department of Education of Jinagsu Province (JHB05-46) and grants from the health department of Jinagsu Province (H200630).
References
- Wang , GS . 1989 . Medical uses of Mylabris in ancient China and recent studies . J. Ethnopharmacol. , 26 ( 2 ) : 147 – 162 .
- Liu , X , Paul , WSH , Li , Q and Chan , LW . 2006 . Novel polymeric microspheres containing norcantharidin for chemoembolization . J. Control Release , 116 ( 1 ) : 35 – 41 .
- Liu , XH , Blazsek , I , Comisso , M , Legras , S , Marion , S , Quittet , P , Anjo , A , Wang , GS and Misset , JL . 1995 . Effects of norcantharidin, a protein phosphatase type-2A inhibitor, on the growth of normal and malignant haemopoietic cells . Eur. J. Cancer , 31A ( 6 ) : 953 – 963 .
- Wang , GS . 1983 . Hydrolyze of norcantharidin and subtracting methyla alleviate stimulation of renal system . Chin. Pharm. Bull. , 18 ( 7 ) : 18 – 20 .
- Wang , LX , He , HB , Tang , X , Shao , RY and Chen , DW . 2006 . A less irritant norcantharidin lipid microspheres: Formulation and drug distribution . Int. J. Pharm. , 323 ( 1–2 ) : 161 – 167 .
- Zhang , W , Gong , JH and Zhang , XN . 2008 . Multi-variable regression analysis applied to synchronously optimize preparation of nanoparticles . Chin. Pharm. J. , 43 ( 15 ) : 1162 – 1166 .
- Zhang , W , Liu , Y and Zhang , XN . 2008 . Spatial representable structure and release in vitro of low molecular weight (LMW) chitosan nanoparticles loading-norcantharidin . Chinese Traditional and Herbal Drugs , 39 ( 10 ) : 4 – 9 .
- Li , Y , Huang , G , Diakur , J and Wiebe , LI . 2008 . Targeted delivery of macromolecular drugs: Asialoglycoprotein receptor (ASGPR) expression by selected hepatoma cell lines used in antiviral drug development . Curr. Drug Deliv. , 5 ( 4 ) : 299 – 302 .
- Morell , AG , Irvine , RA , Sternlieb , I , Scheinberg , IH and Ashwell , G . 1968 . Physical and chemical studies on ceruloplasmin: V. Metabolic studies on sialic acid-free ceruloplasmin in vivo . J. Biol. Chem. , 243 ( 1 ) : 155 – 159 .
- Horev , O , Stokmaier , D , Schwardt , O , Cutting , B and Ernst , B . 2008 . Trivalent, Gal/GalNAc-containing ligands designed for the asialoglycoprotein receptor . Bioorg. Med. Chem. , 16 ( 9 ) : 5216 – 5231 .
- Spiess , M . 1990 . The asialoglycoprotein receptor: A model for endocytic transport receptors . Biochemistry , 29 ( 43 ) : 10009 – 10018 .
- Bettinger , T , Remy , JS and Erbacher , P . 1999 . Size reduction of galactosylated PEI/DNA complexes improves lectin-mediated gene transfer into hepatocytes . Bioconjug. Chem. , 10 ( 4 ) : 558 – 561 .
- Brigger , I , Dubernet , C and Couvreur , P . 2002 . Nanoparticles in cancer therapy and diagnosis . Adv. Drug Deliv. Rev. , 54 ( 5 ) : 631 – 651 .
- Niidome , T , Urakawa , M , Sato , H , Takahara , Y , Anai , T , Hatakayama , T , Wada , A , Hirayama , T and Aoyagi , H . 2000 . Gene transfer into hepatoma cells mediated by galactosemodified alpha-helical peptides . Biomaterials , 21 ( 17 ) : 1811 – 1819 .
- Hattori , Y , Kawakami , S , Yamashita , F and Hashida , M . 2000 . Controlled biodistribution of galactosylated liposomes and incorporated probucol in hepatocyte-selective drug targeting . J. Control. Release , 69 ( 3 ) : 369 – 377 .
- Kawakami , S , Wong , J , Sato , A , Hattori , Y , Yamashita , F and Hashida , M . 2000 . Biodistribution characteristics of mannosylated, fucosylated, and galactosylated liposomes in mice . Biochim. Biophys. Acta , 1524 ( 2–3 ) : 258 – 265 .
- Ruocco , LA , Viggiano , D , Viggiano , A , Abignente , E , Rimoli , MG , Melisi , D , Curcio , A , Nieddu , M , Boatto , G , Carboni , E , Gironi Carnevale , UA and Sadile , AG . 2008 . Galactosylated dopamine enters into the brain, blocks the mesocorticolimbic system and modulates activity and scanning time in Naples high excitability rats . Neuroscience , 152 ( 1 ) : 234 – 244 .
- Xu , ZH , Chen , L , Gu , WW , Gao , Y , Lin , LP , Zhang , ZW , Xi , Y and Li , YP . 2008 . The performance of docetaxel-loaded solid lipid nanoparticles targeted to hepatocellular carcinoma . Biomaterials , 30 ( 2 ) : 226 – 232 .
- Maitani , Y , Kawano , K , Yamada , K , Nagai , T and Takayama , K . 2001 . Efficiency of liposomes surface-modified with soybean-derived sterylglucoside as a liver targeting carrier in HepG2 cells . J. Control. Release , 75 ( 3 ) : 381 – 389 .
- Wang , SN , Deng , YH , Xu , H , Wu , HB , Qiu , YK and Chen , DW . 2006 . Synthesis of a novel galactosylated lipid and its application to the hepatocyte-selective targeting of liposomal doxorubicin . Eur. J. Pharm. Biopharm. , 62 ( 1 ) : 32 – 38 .
- Williams , TJ , Plessas , NR , Goldstein , IJ and Lönngren , J . 1979 . A new class of model glycolipids: Synthesis, characterization, and interaction with lectins . Arch. Biochem. Biophys. , 195 ( 1 ) : 145 – 151 .
- Mantovani , G , Lecolley , F , Tao , L , Haddleton , DM , Clerx , J , Cornelissen , JJ and Velonia , K . 2005 . Design and synthesis of N-maleimido-functionalized hydrophilic polymers via copper-mediated living radical polymerization: A suitable alternative to PEGylation chemistry . J. Am. Chem. Soc. , 127 ( 9 ) : 2966 – 2973 .
- Calvo , P , Remuñan-López , C , Vila-Jato , JL and Alonso , MJ . 1997 . Chitosan and chitosan/ethylene oxide-propylene oxide block copolymer NPs as novel carriers for proteins and vaccines . Pharm. Res. , 14 ( 10 ) : 1431 – 1436 .
- Yang , Z-Q , Xu , J , Pan , P and Zhang , X-N . 2008 . Preparation of an alternative freeze-dried PH-sensitive cyclosporine A nanoparticle formulation and its pharmacokinetic profile in rats . Pharmazie , 63 ( 6 ) : 26 – 31 .
- Teng , B-S , Lu , Y-H , Wang , Z-T , Tao , X-Y and Wei , D-Z . 2006 . In vitro anti-tumor activity of isorhamnetin isolated from Hippophae rhamnoides L against BEL-7402 cells . Pharm. Res. , 54 ( 3 ) : 186 – 194 .
- Kim , CK , Min , KH , Oh , YK , Park , KM and Kim , KM . 1996 . Preparation and lectin binding characteristics of N-stearyl lactobionamide liposomes . Intern. J. Pharm. , 128 ( 1–2 ) : 65 – 71 .
- Liang , HF , Yang , TF , Huang , CT , Chen , MC and Sung , HW . 2005 . Preparation of nanoparticles composed of poly(gamma-glutamic acid)-poly(lactide) block copolymers and evaluation of their uptake by HepG2 cells . J. Control. Release , 105 ( 30 ) : 213 – 225 .
- Wu , J and Zern , MA . 1996 . Modification of liposomes for liver targeting . J. Hepatol. , 24 ( 6 ) : 757 – 763 .
- Wu , J , Liu , L , Yen , R , Le , HT , Zern , MA , Nantz , MH and Marklund , SL . 2003 . Polycationic liposome-mediated extracellular superoxide dismutase gene delivery prevents acute liver injury in mice . Hepatology , 38 ( 1 ) : 240 – 241 .
- Wu , J and Zern , MA . 2004 . Tissue transglutaminase, a key enzyme involved in liver diseases . Hepatol. Res. , 29 ( 1 ) : 1 – 8 .
- Gao , S , Chen , J , Xu , X , Ding , Z , Yang , Y-H , Hua , Z and Zhang , J . 2003 . Galactosylated low molecular weight chitosan as DNA carrier for hepatocyte-targeting . Int. J. Pharm. , 255 ( 1–2 ) : 57 – 68 .
- Maitani , Y , Kawano , K , Yamada , K , Nagai , T and Takayama , K . 2001 . Efficiency of liposomes surface-modified with soybean-derived sterylglucoside as a liver targeting carrier in HepG2 cells . J. Control. Release , 75 ( 3 ) : 381 – 389 .
- Stewart , SG , Hill , TA , Gilbert , J , Ackland , SP , Sakoff , JA and McCluskey , A . 2007 . Synthesis and biological evaluation of norcantharidin analogues: Towards PP1 selectivity . Bioorg. Med. Chem. , 15 ( 23 ) : 7301 – 7310 .
- Hill , TA , Stewart , SG , Ackland , SP , Gilbert , J , Sauer , B , Sakoff , JA and McCluskey , A . 2007 . Norcantharimides, synthesis and anticancer activity: Synthesis of new norcantharidin analogues and their anticancer evaluation . Bioorg. Med. Chem. , 15 ( 18 ) : 6126 – 6134 .
- Hill , TA , Stewart , SG , Sauer , B , Gilbert , J , Ackland , SP , Sakoff , JA and McCluskey , A . 2007 . Heterocyclic substituted cantharidin and norcantharidin analogues—synthesis, protein phosphatase (1 and 2A) inhibition, and anti-cancer activity . Bioorg. Med. Chem. , 17 ( 12 ) : 3392 – 3397 .
- Byrne , JD , Betancourt , T and Brannon-Peppas , L . 2008 . Active targeting schemes for nanoparticle systems in cancer therapeutics . Adv. Drug Deliv. Rev. , 60 ( 15 ) : 1615 – 1626 .
- Shimura , N , Sogawa , Y , Kawakita , Y , Ikekita , M , Yamazaki , N and Kojima , S . 2002 . Radioiodination of glycoprotein-conjugated liposomes by using the Bolton-Hunter reagent and biodistribution in tumor-bearing mice . Nucl. Med. Biol. , 29 ( 4 ) : 491 – 496 .
- Xiong , XB , Huang , Y , Lu , WL , Zhang , X , Zhang , H , Nagai , TJ and Zhang , Q . 2005 . Enhanced intracellular delivery and improved antitumor efficacy of doxorubicin by sterically stabilized liposomes modified with a synthetic RGD mimetic . J. Control. Release , 107 ( 2–3 ) : 262 – 275 .
- Bodnar , M and Foryś , U . 2009 . A model of immune system with time-dependent immune reactivity . Nonlinear Anal. Theory Methods Appl. , 70 ( 2 ) : 1049 – 1058 .