Abstract
The nano-crystalline B3+ and F− co-doped titanium dioxide films were successfully prepared by the improved sol–gel process. The as-prepared specimens were characterised using X-ray diffraction (XRD), high-resolution field emission scanning electron microscopy (FE-SEM), the Brunauer–Emmett–Teller (BET) surface area, X-ray photo-electron spectroscopy, photoluminescence spectra and UV–Vis diffuse reflectance spectroscopy. The photo-catalytic activities of the films were evaluated by degradation of an organic dye in aqueous solution. The results of XRD, FE-SEM and BET analysis indicated that the TiO2 films were composed of nano-particles. B3+ and F− co-doping could obviously not only suppress the formation of brookite phase but also inhibit the transformation of anatase to rutile at high temperature. Diffuse reflectance measurements showed that co-doping could clearly extend the absorbance spectra of TiO2 into visible region. Compared with pure TiO2, B3+ doped or F− doped TiO2 film, the B3+ and F− co-doped TiO2 film exhibited excellent photo-catalytic activity. It is believed that the surface microstructure of the films and the doping methods of the two ions are responsible for improving the photo-catalytic activity.
1. Introduction
Photo-catalytic degradation of organic pollutants on oxide semiconductor surfaces is of vital interest with regard to both fundamental understanding and potential practical applications Citation1,Citation2. Among various oxide semiconductor photo-catalysts, titanium dioxide (TiO2) is considered a promising photo-catalyst because of its strong oxidising power, photo-stability, non-toxicity, chemical and biological inertness, as well as its low cost Citation3–6. However, the widespread technological use of TiO2 has been limited by its wide band gap (3.2 eV), because only under irradiation of light wavelength <387 nm can TiO2 realise its photo-catalytic activity, while natural solar spectrum contains less than 5%. Therefore, any shift in the optical response of TiO2 from the ultraviolet (UV) to the visible spectral range will have a profound positive effect on the photo-catalytic efficiency of this material Citation7.
It is well-known that the photo-catalytic activity of the TiO2 is due to the production of photo-generated electrons and corresponding positive holes under UV illumination. However, the species are unstable, and the recombination of electrons and holes can occur very quickly. In fact, the photo-catalytic efficiency depends on the competition between the surface charge carrier transfer rate and the electron–hole recombination rate. If the recombination rate is so fast, there is no photo-catalytic activity Citation8. To reduce the recombination of photo-generated electrons and holes, and to extend the light absorption of TiO2 into visible light region, many approaches have been carried out including various preparations, different carriers, surface modifications, etc. Among the methods, ion doping was proved to be simple and effective. It has been reported that doping of titania with metal ions, such as noble metal Citation9,Citation10, transition metal Citation11–13 and rare earth metal Citation14,Citation15 can increase photo-catalytic performance. However, metal ion doped photo-catalysts have been shown to suffer from thermal instability. The metal centres can act as electron traps, which reduce the photo-catalytic efficiency Citation16,Citation17. Since Asahi et al. Citation18 reported that N-doped TiO2 had shifted the absorption edge to wavelengths longer than 400 nm and enhance the photo-catalytic activities under visible light in 2001, many researches have focused on TiO2 doping with nonmetals, such as nitrogen Citation19, carbon Citation20, sulphur Citation21, fluorine Citation22, boron Citation23 and phosphor Citation24. Furthermore, there were also a few reports on titania photo-catalysts co-doped by two kinds of nonmetals Citation25. Recently, Ling et al. Citation26 prepared the B–N co-doped TiO2 photo-catalyst. They tested the photo-catalytic activity of materials by decomposing the phenol under visible light illumination and indicated that a remarkable reactivity improvement was observed. Xie and Zhao Citation27 prepared the F–N co-doped titania photo-catalysts, and photo-catalytic decomposition of methyl orange showed that the catalysts could work efficiently under visible light irradiation. These nonmetal ion doped catalysts may be more appropriate for extending the photo-catalytic activity of TiO2 into the visible region than other methods because their impurity states are near the valance band edge and their roles as recombination centres might be minimised, as compared to that of metal cation doping.
To avoid the use of powdered TiO2, which has to be separated from the water in a slurry system after photo-catalytic reaction, investigations on TiO2 photo-catalysis have been oriented towards the photo-catalyst immobilisation in the form of a thin film. Up to now many methods, including sol–gel technology, chemical vapour deposition, plasma spraying, anodisation, thermal oxidation of titanium metal, etc, can be used to prepare TiO2 films on the various substrates. In these processes, the sol–gel method has many advantages over other methods Citation28,Citation29. By the sol–gel method, no special apparatuses are required, uniform multi-component films can be easily formed, phase structure can be controlled and coatings of complex geometry can be allowed Citation30.
In this study, we adopted an improved sol–gel process to prepare B3+ and F− co-doped TiO2 films. To the best of our knowledge, there were few studies concentrating on B3+and F− co-doped TiO2 films for the photo-catalytic activity. This study may provide new insights and understanding on the mechanism of photo-activity enhancement by B3+ and F− co-doping.
2. Experimental
2.1. Materials and methods
Colloidal TiO2 was prepared by the sol–gel method. A total volume of 1 mL TiO(C4H9O)4 (AnalaR grade, China) was dissolved in 20 mL absolute ethanol (AnalaR grade, China) under vigorous stirring in a clean and dry vessel at room temperature. After 5 min, 20 mL of 0.2 M diluted nitric acid (AnalaR grade, China) was added at the rate of 1 drop/s and kept on stirring till the colloidal suspension was obtained, as seen in our previous work Citation31. TiO2 sol-films were coated to the surface of glass substrate (25 × 25 × 1 mm3) by a controllable dip-coating device in an ambient atmosphere. The speed withdrawal was controlled at 3 mm/s. After dip-coating for different times, the TiO2 sol- or gel-films of different thicknesses were obtained. A certain concentration of boric acid H3BO3 (AnalaR grade, China) and hydrofluoric acid HF (AnalaR grade, China) aqueous solution was doped into the surface layer of TiO2 gel-film, which is called external doping or surface layer doping. Then the TiO2 gel-films doped ions on glass substrate were calcined in air at the rate of 5°C/min up to 450°C and were left to stay in the furnace for 1 h for organic removal and to obtain crystalline TiO2. Usually, ions are directly doped into TiO2 sol precursor, namely ions are in the bulk material, which is called internal doping or bulk phase doping. Compared with the external doping, the drawback of the internal doping is lower photo-catalytic efficiency, because of the need of the ions to diffuse from the bulk into the surface of materials.
The thickness of TiO2 film can be approximately calculated by the formula: H = W 2 − W 1/ρ × l × d, in which H is the thickness of film, W 1 is the weight of glass substrate before coated film while W 2 after it, ρ is the density of TiO2 and l and d are the length and width of substrate, respectively. In our experiments, the thickness of TiO2 film was 1.52 µm ± 5%.
2.2. Catalyst characterisation
Surface morphology of the TiO2 film was characterised by high-resolution field emission scanning electron microscopy (FE-SEM). The X-ray diffraction (XRD) patterns obtained on a diffractometer (type DX-2500) using Cu-Kα radiation at a scan rate (2θ) of 0.05/s were used to determine the identity of crystalline phase and the crystallite size. The accelerating voltage and the applied current were 40 kV and 25 mA, respectively. The composition of the TiO2 film was investigated using X-ray photo-electron spectroscopy (XPS). The recombination of electron–hole in the samples was studied by photoluminescence (PL) emission spectra. The Brunauer–Emmett–Teller (BET) surface area of the TiO2 samples was analysed by nitrogen adsorption/desorption apparatus. The surface area was determined by the multipoint BET method using the adsorption data in the relative pressure (P/P 0) range 0–1.0, and the desorption isotherm was used to determine the pore size distribution using the Barret–Joyner–Halender (BJH) method. The nitrogen adsorption volume at the relative pressure (P/P 0) of 0.975 was used to determine the pore volume and average pore size.
2.3. Catalyst test
The photo-catalytic efficiency was evaluated by the degradation of a organic dye (ANR, acid naphthol red) in aqueous solutions under UV-lamp irradiation with a wavelength of 365 nm for 45 min. Ion doped or un-doped TiO2 films settled in 5 mL aqueous ANR with a concentration of 1.0 × 10−4 mol/dm3 in a weighing bottle. The averaged intensity of UV irradiance 6.5 mW/cm2 was obtained by measuring with a UV irradiance meter (Model UV-A). UV–Vis Spectrometer (TU-1901) was adopted to assess the photo-degradation activity of the film photo-catalysts. Their photo-catalytic degradation rate can be calculated by the formula: D = (A 0 − A)/A 0 × 100%, in which A 0 is the absorbency of the organic dye solution before illumination while A after it.
3. Results and discussion
3.1. Doping methods
Different doping methods of metal ions have an effect on the photo-catalytic activity of nano-crystalline TiO2 films. shows the degradation rate of ANR solutions using TiO2 film doped ions by different doping methods under the same conditions. From , it can be seen that the degradation rates of aqueous ANR using un-doping TiO2 film is 25.1%, compared with that of external doping B3+ TiO2 film, 36.2%; external doping F− TiO2 film, 42.4%; external co-doping B3+/F− TiO2 film, 58.6%; and internal co-doping B3+/F− TiO2 film, 45.3%. Obviously, we can draw the conclusion that the photo-catalytic activity of co-doping ions in TiO2 film is better than that of single doping ions, and the photo-catalytic activity of external doping ions in TiO2 film is better than that of internal doping ions. Two mechanisms were proposed to explain this fact. One is that the synergistic effect of B3+ and F− ion co-doping is favourable to the photo-reactions. B3+ ions act as a photo-generated electron trap due to their positive charge, while F− ions act as a photo-generated hole trap due to their negative charge, thus inhibiting the hole–electron recombination and improving photo-catalytic activity. The other mechanism is that the short-range effect of ion external doping can enhance the photo-catalytic activity of the TiO2 film.
Table 1. Effect of doping methods on photo-degradation rate of TiO2 film.
It is well-known that photo-generated electrons and holes are produced on the surface of TiO2 film under UV illumination. Therefore, the photo-catalytic efficiency depends on the competition between the surface charge carrier transfer rate and the electron–hole recombination rate. Nonmetal ion doping reduces the recombination of the species. However, different doping methods of the same nonmetal ions have different photo-catalytic efficiencies. For the case of external doping, doped ions are on the surface layer of TiO2 films, the distances from ions to electrons or holes are short, and so doped ions can effectively attract electrons or holes and enable electron–hole separation immediately. On the contrary, in the case of internal doping, some of doped ions are present in the bulk materials, so that the ions must diffuse from the bulk phase into the surface of TiO2 films. Because the photo-generated electrons or holes cannot be separated immediately, some of them recombine to generate heat, that is there is no photo-catalytic activity. Therefore, the photo-catalytic activity of internal doping of ions in TiO2 film is lower than that of external doping of ion in TiO2 film.
3.2. Catalyst characterisation
Surface morphology of pure TiO2 films and B3+ and F− co-doped TiO2 films calcined at 450°C in air for 1.0 h is shown in . It is clear that the film obtained by co-doping is composed of nano-particles or aggregates, indicating that B3+ and F− co-doping can effectively decrease the aggregation of the TiO2 nanometre particles, making the surface of the film uniform and smooth, and therefore keeping their special nano-characteristics.
shows the XRD spectra of the un-doped and co-doped ion TiO2 samples calcined at 450°C in air for 1.0 h. The samples exhibit a good crystallinity constituting a mixture of anatase (25.6°, 37.6° and 48.2°), rutile (27.5° and 36.2°) and brookite (30.9°), but anatase is the predominant crystal style. From , it can be seen that the intensities of rutile and brookite peaks became weaker and rutile peaks (27.5°, 36.2°) even disappeared after B3+ and F− co-doping, indicating that B3+ and F− co-doping can not only suppress the formation of brookite phase but also inhibit the transformation of anatase to rutile at high temperatures. According to Scherrer equation: D = 0.89 λ/βcos θ, where β is the half-height width of the diffraction peak of anatase, θ is the diffraction angle and λ is the X-ray wavelength corresponding to the Cu-Kα radiation, and we can calculate that the average size of pure TiO2 particles is 7.7 nm, compared with 6.1 nm of B3+ and F− co-doped TiO2. The smaller particles and the larger anatase content of the TiO2 samples obtained by B3+ and F− co-doping are favourable to the photo-catalytic degradation Citation32.
Specific surface area is a very important factor for photo-catalytic activity. N2 adsorption/desorption isotherms of pure TiO2 samples and B3+ and F− co-doped TiO2 samples calcined at 450°C in air for 1.0 h are shown in . From , we can know that the isotherms of two samples exhibit hysteresis loops at high relative pressures from 0.4 to 0.8. The sharp decline in desorption curve is indicative of mesoporosity, and the hysteresis loop demonstrates that there exists an ink bottle or a bottle-neck mesoporous structure, possibly caused by nonuniform pore size. It is evident that with the same relative pressure co-doped TiO2 samples have higher adsorption quantities as well as higher surface area, which can be proved by . In our experiment, specific surface area of B3+ and F− co-doped TiO2 sample is 125.69 m2/g, more than that of pure TiO2 sample 95.88 m2/g.
Table 2. Effect of B3+ and F− co-doping on BET surface areas and pore parameters of TiO2 samples.
The pore size distributions of the as-prepared samples are shown in , indicating that the diameter range of most pores locates from 3 to 4 nm, as seen from . From , it can be seen that the pores with size ranges 3–4 nm reach as high as 76.82% after B3+ and F− co-doped TiO2, compared with 68.12% of un-doped TiO2. The formation of mesostructure in the samples is attributed to the aggregation of TiO2 crystallites. Ion co-doping causing the decrease in the average pore size is mainly due to the fact that doping ions get inserted into the pore of mesoporous TiO2 resulting in smaller pore size Citation33. The smaller pores and the larger specific surface area of the TiO2 samples by B3+ and F− co-doping are favourable to the photo-catalytic degradation.
Table 3. Effect of B3+ and F− co-doping on BET pore size distribution of TiO2 samples.
XPS spectroscopy can be used to analyse the composition of the TiO2 film. The XPS spectra of the B3+ and F− co-doped TiO2 are shown in . With respect to the XPS peaks of Ti 2p, although there are slight differences in the locations of binding energies of Ti 2p1/2 and Ti 2p3/2, they are still in good agreement with the values of Ti4+ Citation34. The O 1s binding energies of the samples are located at about 531 eV, which is assigned to bulk oxide in the TiO2 lattice. Therefore, it can be concluded that no obvious changes on the chemical state of Ti and O can be caused by co-doped B3+ and F−. The calculated O/Ti intensity ratio in the titania film is 2.12. This indicates that the doped titania film has a composition close to the stoichiometry of TiO2. B3+ or F− has not been detected by XPS due to the small amount that was present.
PL emission is useful to disclose the efficiency of charge carrier trapping, immigration and transfer, and to understand the fate of electron–hole pairs in semiconductor particles Citation35. The PL emission spectra of the three samples were examined in the wavelength range 300–550 nm in our study, as shown in . It can be observed that the positions of the peaks at about 380 nm which are assigned to the emission of the band gap transition are similar while PL intensities are different among these samples. The PL intensity of B3+ and F− external co-doped TiO2 is the lowest among all samples, indicating that the recombination of electron and hole has been effectively suppressed compared with pure TiO2 or B3+ and F− internal co-doped TiO2. Our PL measurement results confirm that the higher the photo-catalytic activity, the lower the intensity of PL spectra.
3.3. Photo-catalytic activity measurement
shows the UV–Vis absorption spectra of doped B3+ and un-doped TiO2 films on glass substrates in ANR solutions under UV-lamp irradiation with wavelength of 365 nm for 45 min. From , it can be seen that B3+ cations doping can apparently improve the photo-decomposition of aqueous ANR and the dopant concentrations can also influence the degradation activities. The absorbance becomes lower, that is the degradation rate grows higher, so that the degradation ratio is in the order of B3+ doped concentration: 1.3 × 10−2 M > 1.7 × 10−2 M > 0.9 × 10−2 M > pureTiO2. Since the ion dopant can serve not only as a mediator of interfacial charge transfer but also as a recombination centre, the photo-catalytic activity of B3+ doped TiO2 is strongly dependent on the dopant concentration. In our case, the doping content of B3+ in the TiO2 film is 0.11 wt%. Above the concentration, B3+ ions may occupy the active sites on the TiO2 surface or become the recombination centres of photo-generated electrons and holes, which will lead to the photo-catalyst to lose its activity.
Figure 7. UV–Vis absorption spectra of B3+ doped and un-doped TiO2 films on glass substrates in ANR solutions after irradiated for 45 min: (1) 0.9 × 10−2 M, (2) 1.3 × 10−2 M and (3) 1.7 × 10−2 M.
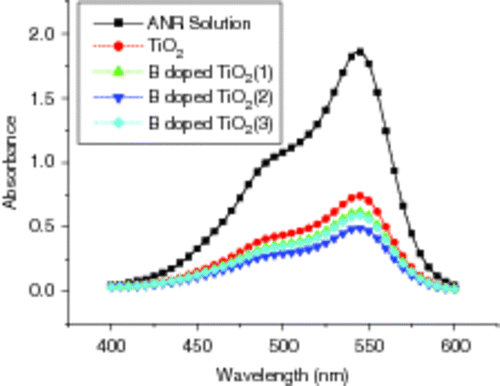
shows the UV–Vis absorption spectra of doped F− and un-doped TiO2 films on glass substrates in ANR solutions under UV-lamp irradiation with wavelength of 365 nm for 45 min. From , it can be seen that that F− anions doping can also improve the photo-decomposition of aqueous ANR, and the degradation ratio is in the order of F− doped concentration: 5 × 10−3 M > 1 × 10−3 M > 9 × 10−3 M > pureTiO2. In our case, the doping content of F− in the TiO2 film is 0.09 wt%. Above the concentration, F− ions may induce more trapping sites and cause the distance between them to decrease due to the recombination of photo-excited electrons and holes, which then reduce the photo-catalytic degradation.
Figure 8. UV–Vis absorption spectra of F− doped and un-doped TiO2 films on glass substrates in ANR solutions after irradiated for 45 min: (1) 1 × 10−3 M, (2) 5 × 10−3 M and (3) 9 × 10−3 M.
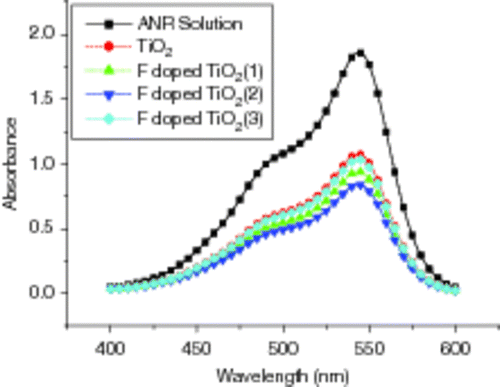
shows the UV–Vis absorption spectra of B3+ and F− co-doped TiO2 films on glass substrates in ANR solutions under UV-lamp irradiation with wavelength of 365 nm for 45 min. From , it can be seen the dependence of the degradation of aqueous ANR on the various amounts of B3+ and F− co-doping. In our case, the volume of B3+ doping is fixed to 0.05 mL (B3+ doping concentration chosen 1.3 × 10−2 M) while the volume of F− doping is changeable (F− doping concentration chosen 5 × 10−3 M). We can know from that with the increase in F− doped amount, the degradation rate increases quickly. When the amount of F− increases to 0.15 mL, the degradation rate reaches the highest, while above 0.15 mL it declines. Therefore, co-doping ions also need an appropriate amount. In our case, the doping contents of B3+ and F− in the TiO2 film are 0.11 and 0.27 wt%, respectively.
Figure 9. UV–Vis absorption spectra of B3+ and F− co-doped TiO2 films on glass substrates in ANR solutions after irradiated for 45 min: the amount of B3+ doping fixed to 0.05 mL, while those of F− are (1) 0.025 mL, (2) 0.05 mL, (3) 0.10 mL, (4) 0.15 mL, (5) 0.20 mL and (6) 0.25 mL.
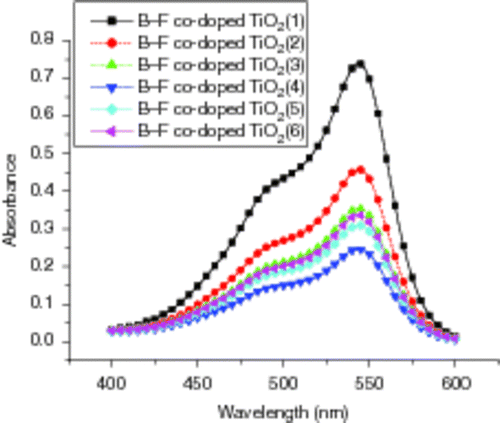
shows the degradation rate of doped and un-doped TiO2 films on glass substrates in ANR solutions under UV-lamp irradiation with wavelength of 365 nm for 45 min. From , it can be seen that the degradation rate of aqueous ANR using TiO2 film is 25%, compared with that of B3+ doped TiO2 film, 36%; F− doped TiO2 film, 42% and B3+ and F− co-doped TiO2 film, 58%. Obviously, we can draw the conclusion that B3+ and F− co-doped TiO2 films have the best photo-catalytic activity under the same conditions. This may be owing to the synergistic effect of co-doped B3+ and F− on the enhancement of photo-catalytic activity. An appropriate amount of B3+ ions mainly act as photo-generated electron trap due to their positive charge and to inhibit the hole–electron recombination. Meanwhile, an appropriate amount of F− ions mainly act as photo-generated hole trap due to their negative charge and to inhibit the hole–electron recombination. Thus, the photo-catalytic activity can be improved greatly.
Figure 10. Degradation rate of ANR solutions using doped and un-doped TiO2 films on glass substrates under UV-lamp irradiation with a wavelength of 365 nm for 45 min.
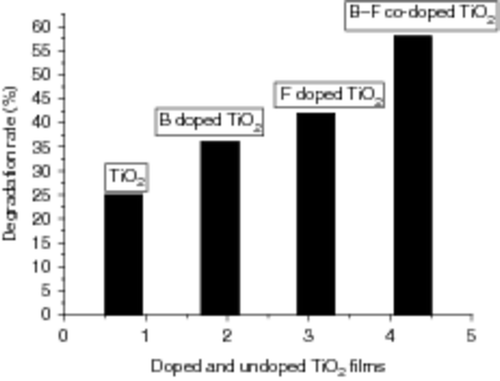
Diffuse reflection spectra of pure TiO2 films and B3+ and F− co-doped TiO2 films are shown in . It is clear that the absorbance onset of co-doped TiO2 film red shift to about 450 nm compared with that of pure TiO2 film 350 nm, since B3+ and F− co-doped ions can enhance the intensity of absorption in the visible light region, decrease the band gap and result in the response to the visible light of photo-catalysts Citation26. Moreover, the impurity states of the nonmetals are near the valance band edge and their roles as recombination centres might be minimised Citation7. These factors can induce more photo-generated electrons and holes to participate in the photo-catalytic reactions, and improve the photo-catalytic activity of the TiO2.
Figure 11. Diffuse reflection UV–Vis spectra of pure TiO2 film and TiO2 film co-doped with B3+ and F−.
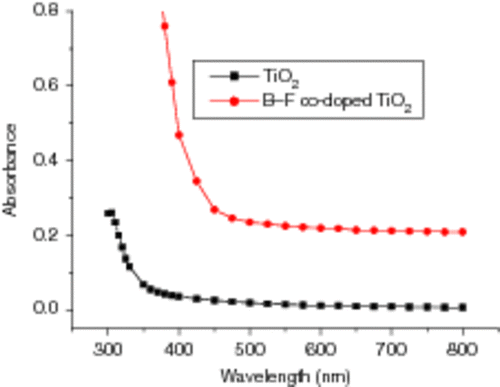
shows the degradation curves of ANR solutions using an appropriate amount of F−, B3+, F−/B3+ doped and un-doped TiO2 films on glass substrates after visible light irradiated for 50 min. A 300-W tungsten halogen lamp equipped with a UV cut-off filters (λ > 400 nm) was used as a visible light source. From it can seen that the F− and B3+ co-doped TiO2 films exhibit excellent photo-catalytic activity under visible light irradiation. The enhancement of the photo-reactivity with F− and B3+ co-doping may be caused by the wavelength shift to visible region and may increase the number of photo-excited electrons and holes. This result is very important for the efficient use of visible or solar light in the future Citation18.
Figure 12. Photo-catalytic degradation curves of ANR solutions using doped and un-doped TiO2 films on glass substrates after being visible light irradiated.
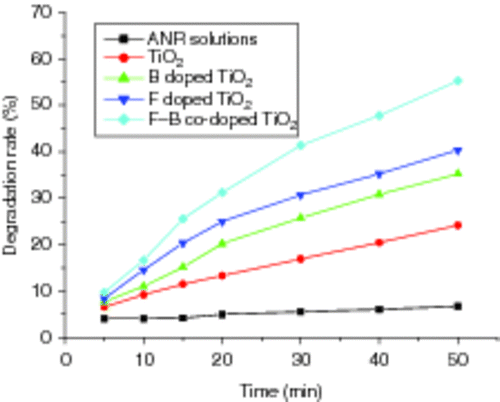
It is on the surface of TiO2 film that photo-generated electrons and holes are produced, and other different types of radicals, such as OH, are subsequently formed, which are then free to carry out further chemical reaction at the titania surface. The photo-catalytic efficiency depends on the competition between the surface charge carrier transfer rate and the electron–hole recombination rate. If the recombination rate is very high, there is no enough time for any other reactions to occur; that is to say, there is no photo-catalytic activity. To reduce the recombination of photo-generated electrons and holes, we adopted an improved sol–gel process. By the method, nonmetals were doped onto the surface of TiO2 colloidal films, i.e. outer doping. This results in that B3+ ions, which are on the surface of the TiO2 film, can effectively attract photo-generated electrons and separate holes on the surface of the TiO2 film, while F− ions, which also are on the surface of the TiO2 film, can effectively attract photo-generated holes and separate electrons on the surface of the TiO2 film. If the amount of B3+ and F− co-doping is appropriate, it allows positive holes or negative electrons to have relatively long life, and enhance the photo-catalytic activity of the TiO2 film. The major steps in the mechanism may be summarised by Equations (Equation1–8).
In a word, B3+ and F− co-doping may have the synergistic effect. B3+ ion has a completely empty p orbital (p0), which is relatively stable, and electrons trapped can be easily released, so it elongates the life of photo-excited electrons and holes. While F− ion has a completely filled p orbital (p6), which is also relatively stable, and holes trapped can be easily released, it elongates the life of photo-excited electrons and holes. Moreover, B3+ and F− co-doping can change the surface microstructure of TiO2 films, including morphology of surface, grain size and crystalline phase of TiO2, pore size and distribution, and so on, while the surface microstructure is of great influence on the photo-catalytic activity of TiO2 films. According to FE-SEM, XRD and BET analyses, B3+ and F− co-doping can effectively lessen the aggregation of the TiO2 nano-particles, induce the uniformity in the pores, decrease the number of micro-pores and increase the specific surface area of the TiO2 films, as well as inhibit the transformation of anatase to rutile at high temperature. These factors lead to effective photo-degradation of organic dyes. Based on the above-mentioned beneficial properties of B3+ and F− ions, their modifications to TiO2 film photo-catalyst show significant improvement effect in the degradation of organic dye solutions. Moreover, such modification can lead to the redshift of absorption edge of TiO2 improving the visible light activity.
4. Conclusions
B3+ and F− co-doped TiO2 films have been prepared on the surface of glass substrate by an improved sol–gel process. Experimental results show that the B3+ and F− co-doped TiO2 films have much higher photo-catalytic efficiency as well as an obvious redshift, which may be due to the microstructure of the TiO2 films and the synergistic effect of the two nonmetal ions. The redshift of absorption edge of TiO2 by co-doping may promise a potential application for TiO2 films in visible light, such as photo-catalytic degradation of organic pollutants in solar light. Moreover, modifying TiO2 films can realise recycle and reuse in real wastewater treatment.
Acknowledgements
This study was financially supported partially by National Natural Science Foundation of China (grant No. 20674005) and the Doctorial Foundation of University of Jinan (B0606).
References
- Mori , K , Maki , K , Kawasaki , S , Yuan , S and Yamashita , H . 2008 . Hydrothermal synthesis of TiO2 photocatalysts in the presence of NH4F and their application for degradation of organic compounds . Chem. Eng. Sci. , 63 : 5066 – 5070 .
- Han , F , Kambala , VSR , Srinivasan , M , Rajarathnam , D and Naidu , R . 2009 . Tailored titanium dioxide photocatalysts for the degradation of organic dyes in wastewater treatment: A review . Appl. Catal., A , 359 : 25 – 40 .
- Fujishima , A , Rao , TN and Tryk , DA . 2000 . Titanium dioxide photocatalysis . J. Photochem. Photobiol., C , 1 : 1 – 12 .
- Hu , C , Yu , JC , Hao , ZP and Wong , PK . 2003 . Photocatalytic degradation of triazine-containing azo dyes in aqueous TiO2 suspensions . Appl. Catal., B , 42 : 47 – 55 .
- Yu , JC , Ho , W and Yu , J . 2005 . Efficient visible-light-induced photocatalytic disinfection on sulfur-doped nanocrystalline titania . Environ. Sci. Technol. , 39 : 1175 – 1179 .
- Wu , XH , Ding , XB , Qin , W , He , WD and Jiang , ZH . 2006 . Enhanced photo-catalytic activity of TiO2 films with doped La prepared by micro-plasma oxidation method . J. Hazard. Mater. , 137 : 192 – 197 .
- Im , JS , Yun , SM and Lee , YS . 2009 . Investigation of multielemental catalysts based on decreasing the band gap of titania for enhanced visible light photocatalysis . J. Colloid Interface Sci. , 336 : 183 – 188 .
- Yu , JC , Yu , J , Ho , W , Jiang , Z and Zhang , L . 2002 . Effects of F-doping on the photocatalytic activity and microstructures of nanocrystalline TiO2 powders . Chem. Mater. , 14 : 3808 – 3816 .
- Sclafani , A , Mozzanega , MN and Herrmann , JM . 1997 . Influence of silver deposits on the photocatalytic activity of titania . J. Catal. , 168 : 117 – 120 .
- Anpo , M and Takeuchi , M . 2003 . The design and development of highly reactive titanium oxide photocatalysts operating under visible light irradiation . J. Catal. , 216 : 505 – 516 .
- Choi , W , Termin , A and Hoffmann , MR . 1994 . The role of metal ion dopants in quantum-sized TiO2: Correlation between photoreactivity and charge carrier recombination dynamics . J. Phys. Chem. , 98 : 13669 – 13679 .
- Wang , J , Uma , S and Klabunde , KJ . 2004 . Visible light photocatalysis in transition metal incorporated titania-silica aerogels . Appl. Catal., B , 48 : 151 – 154 .
- Lam , RCW , Leung , MKH , Leung , DYC , Vrijmoed , LLP , Yam , WC and Ng , SP . 2007 . Visible-light-assisted photocatalytic degradation of gaseous formaldehyde by parallel-plate reactor coated with Cr ion-implanted TiO2 thin film . Sol. Energy Mater. Sol. Cells , 91 : 54 – 61 .
- Xu , AW , Gao , Y and Liu , HQ . 2002 . The preparation, characterization, and their photocatalytic activities of rare-earth-doped TiO2 nanoparticles . J. Catal. , 207 : 151 – 157 .
- Wang , YQ , Cheng , HM , Zhang , L , Hao , YZ , Ma , JM , Xu , B and Li , WH . 2000 . The preparation, characterization, photoelectrochemical and photocatalytic properties of lanthanide metal-ion-doped TiO2 nanoparticles . J. Mol. Catal. A: Chem. , 151 : 205 – 216 .
- Li , YZ , Hwang , DS , Lee , NH and Kim , SJ . 2005 . Synthesis and characterization of carbon-doped titania as an artificial solar light sensitive photocatalyst . Chem. Phys. Lett. , 404 : 25 – 29 .
- Zou , ZG , Zhao , JC , Fu , XZ , Zhang , PY , Chen , J , Zhu , HM and Ye , JH . 2004 . Development on solar energy conversion and environmental applications of photocatalysis . J. Funct. Mater. , 35 : 83 – 88 .
- Asahi , R , Morikawa , T , Ohwaki , T , Aoki , K and Taga , Y . 2001 . Visible-light photocatalysis in nitrogen-doped titanium oxides . Science , 293 : 269 – 271 .
- Li , DZ , Huang , HJ , Chen , X , Chen , ZX , Li , WJ , Ye , D and Fu , XZ . 2007 . New synthesis of excellent visible-light TiO2 − xNx photocatalyst using a very simple method . J. Solid State Chem. , 180 : 2630 – 2634 .
- Li , Y , Hwang , DS , Lee , NH and Kim , SJ . 2005 . Synthesis and characterization of carbon-doped titania as an artificial solar light sensitive photocatalyst . Phys. Lett. , 404 : 25 – 29 .
- Takeshita , K , Yamakata , A , Ishibashi , TA , Onishi , H , Nishijima , K and Ohno , T . 2006 . Transient IR absorption study of charge carriers photogenerated in sulfur-doped TiO2 . J. Photochem. Photobiol. A , 177 : 269 – 275 .
- Ho , W , Yu , JC and Lee , S . 2006 . Synthesis of hierarchical nanoporous F-doped TiO2 spheres with visible light photocatalytic activity . Chem. Commun. , 10 : 1115 – 1117 .
- Zhao , W , Ma , WH , Chen , CC , Zhao , JC and Shuai , ZG . 2004 . Efficient degradation of toxic organic pollutants with Ni2O3/TiO2 − xBx under visible irradiation . J. Am. Chem. Soc. , 126 : 4782 – 4783 .
- Lin , L , Zheng , RY , Xie , JL , Zhu , YX and Xie , YC . 2007 . Synthesis and characterization of phosphor and nitrogen co-doped titania . Appl. Catal., B , 76 : 196 – 202 .
- Li , D , Haneda , H , Hishita , S and Ohashi , N . 2005 . Visible-light-driven N–F–codoped TiO2 photocatalysts. 1. Synthesis by spray pyrolysis and surface characterization . Chem. Mater. , 17 : 2588 – 2595 .
- Ling , QC , Sun , JZ and Zhou , QY . 2008 . Preparation and characterization of visible-light-driven titania photocatalyst co-doped with boron and nitrogen . Appl. Surf. Sci. , 254 : 3236 – 3241 .
- Xie , Y and Zhao , XJ . 2008 . The effects of synthesis temperature on the structure and visible-light-induced catalytic activity of F–N-codoped and S–N-codoped titania . J. Mol. Catal. A: Chem. , 285 : 142 – 149 .
- Du , WC , Wang , HT , Zhong , W , Shen , L and Du , QG . 2005 . High refractive index films prepared from titanium chloride and methyl methacrylate via a non-aqueous sol–gel route . J. Sol-Gel Sci. Technol. , 34 : 227 – 231 .
- Avci , N , Smet , PF , Poelman , H , Velde , NVK , Buysser , D , Driessche , IV and Poelman , D . 2009 . Characterization of TiO2 powders and thin films prepared by non-aqueous sol–gel techniques . J. Sol-Gel Sci. Technol. , 52 : 424 – 431 .
- Yao , MM , He , YD , Zhang , W and Gao , W . 2005 . Oxidation resistance of boiler steels with Al2O3–Y2O3 nano- and micro-composite coatings produced by sol-gel process . Mater. Trans. , 46 : 2089 – 2092 .
- Li , J , Yao , MM , Zhang , Y and Yang , H . 2009 . Photo-catalytic properties of TiO2–Fe2O3–La2O3 nano-composite films . Surf. Rev. Lett. , 16 : 315 – 321 .
- Liu , G , Wang , XW , Chen , ZG , Cheng , HM and Qing , G . 2009 . The role of crystal phase in determining photocatalytic activity of nitrogen doped TiO2 . J. Colloid Interface Sci. , 329 : 331 – 338 .
- Zhou , MH , Yu , JG , Cheng , B and Yu , HG . 2005 . Preparation and photocatalytic activity of Fe-doped mesoporous titanium dioxide nanocrystalline photocatalysts . Mater. Chem. Phys. , 93 : 159 – 163 .
- Wu , YM , Zhang , JL , Xiao , L and Chen , F . 2009 . Preparation and characterization of TiO2 photocatalysts by Fe3+ doping together with Au deposition for the degradation of organic pollutants . Appl. Catal., B , 88 : 525 – 532 .
- Yamashita , H , Harada , M , Misaka , J , Takeuchi , M , Neppolian , B and Anpo , M . 2003 . Photocatalytic degradation of organic compounds diluted in water using visible light-responsive metal ion-implanted TiO2 catalysts: Fe ion-implanted TiO2 . Catal. Today , 84 : 191 – 196 .