Abstract
In this study, olsalazine (3,3′-azobis 6-hydroxy benzoic acid) was intercalated into Mg–Al layered double hydroxides (LDHs) by ion exchange or coprecipitation methods to obtain olsalazine–LDH nanohybrids. Powder X-ray diffraction (XRD), Fourier transform infrared spectra and elemental analyses indicate a successful intercalation of olsalazine with a vertical orientation. Intercalation of organic anion of olsalazine into Mg–Al LDH caused the interlayer spacing of LDH to increase from 0.884 to 1.665–1.666 nm as determined by XRD studies. Thermogravimetric analyses propose that the thermal stability of the intercalated organic anion of olsalazine is largely improved compared to the pure form before intercalation due to the host–guest interaction involving the hydrogen bond. Furthermore, in vitro drug release experiments in pH 7.4 phosphate buffer solution have been investigated. The drug molecules during dissolution were exchanged with anions in the medium, thus leading to a slow release, much slower than when the same matrix was used simply mixed with the drug.
1. Introduction
Layered double hydroxides (LDHs) or hydrotalcite-like compounds are presented by the general formula The basic structure of an LDH may be derived by substitution of a fraction of the divalent cations in a brucite lattice with trivalent cations such that the layers acquire a positive charge, which is balanced by intercalation of anions (and, usually, water) between the layers. It is the possibility of varying the identity and relative proportions of the di- and trivalent cations, as well as the identity of the interlayer ions, that gives rise to the large variety of materials. On the other hand, nanomaterial-based drug delivery systems have attracted increasing attention to improve the pharmacological and therapeutic efficacy of drugs. Among the various nanoparticles, LDHs are most suitable as drug delivery carriers due to their low toxicity, high reserving capacity and enhanced cellular uptake behaviour Citation1–6. Recently, incorporation of organic anions in the interlayer space of LDHs by an ion-exchange mechanism, a so-called intercalation phenomenon, was used for the synthesis of drugs and inorganic hybrid materials Citation1. Intercalation is a process in which a layered material, referred to as the host, swells or opens to accommodate other molecules or ions, known as the guests Citation7. The degree of swelling of the layers is dependent upon a number of factors, including the size of the guest species and the nature of host–guest and guest–guest interactions. Ion exchange is the most common form of intercalation reaction and occurs with a wide variety of materials. Intercalation materials have a wide range of uses, such as bio-medical applications Citation8, solid state batteries Citation9, catalysts Citation10, acid scavengers Citation11 and composites Citation12. In this study, olsalazine, an anti-inflammatory drug () widely used to treat of inflammatory bowel disease such as ulcerative colitis, is intercalated into Mg–Al–NO3 LDH by coprecipitation or ion-exchange techniques Citation13.
2. Experiment
2.1. Materials
Olsalazine was obtained as described elsewhere Citation14,Citation15. All other chemical reagents were purchased from Merck and used without further purification.
2.1.1. Mg–Al–NO3 LDH preparation
The Mg–Al–NO3 LDH was prepared as described in the literature works Citation16,Citation17 with slight modification at pH 9 by a hydrothermal process in a mixed water/ethanol solvent system and glycerol was used as a surfactant. An NaOH solution (0.75 M) was added dropwise into a 36 mL solution containing Mg(NO3)2.6H2O (0.471 g, 0.0024 mol) and Al(NO3)3.9H2O (0.450 g, 0.0012 mol) in a mixture of distilled water (30 mL), ethanol (5 mL) and glycerol (1 mL) under a nitrogen atmosphere with vigorous magnetic stirring until the final pH of 9. It was then transferred into a Teflon-lined autoclave and hydrothermally treated at 100°C for 24 h. The obtained precipitates were filtered and washed with distilled water to remove any ions possibly remaining in the final products and then dried at 60°C in a vacuum.
2.1.2. Olsalazine–LDH preparation by coprecipitation
The olsalazine-intercalated Mg–Al–NO3 LDH was prepared via the hydrothermal method as described in the literature works Citation13,Citation18 with slight modification. A solution was prepared by adding 36 mL of a mixture of distilled water (30 mL), ethanol (5 mL) and glycerol (1 mL) to Mg(NO3)2.6H2O (0.471 g, 0.0024 mol) and Al(NO3)3.9H2O (0.450 g, 0.0012 mol). The pH of the solution was adjusted to 9 with a solution of 0.75 M NaOH. The solution thus formed was slowly added into a basic solution of the drug prepared by dissolving 0.362 g (0.0012 mol) of olsalazine in 20 mL water under a nitrogen atmosphere with vigorous magnetic stirring. The required amount of 0.75 M NaOH was added during the addition of the salts solution in order to maintain the pH at 9. The resulting slurry was transferred into a Teflon-lined autoclave and hydrothermally treated at 100°C for 24 h. The resultant was then filtered and washed with distilled water and dried at 60°C in a vacuum.
2.1.3. Olsalazine–LDH preparation by anion exchange
The olsalazine-intercalated nitrate LDHs were prepared via the anion-exchange method as described in the literature works Citation13,Citation18 with slight modification. A solution containing 0.330 g (0.0011) of olsalazine in a mixture of distilled water (30 mL) and ethanol (5 mL) at a pH of 9 (with 1 M NaOH) was added to 20 mL of an aqueous suspension of 0.4 g (0.0020 mol) of Mg–Al–NO3 LDHs. The mixture was magnetically stirred under an N2 atmosphere for two days at 65°C. It was then centrifuged and washed several times with decarbonated water. The yellow products were separated via filtration and dried at 60°C in a vacuum.
2.2. Characterisation
Fourier transform infrared (FT-IR) spectra were obtained on a Shimadzu 8400 s spectrophotometer in the range of 4000–400 cm−1 using the KBr pellet technique. Powder X-ray diffraction (XRD) patterns of the samples were recorded on a Bruker AXS model D8 Advance diffractometer using Cu-Kα radiation (λ = 1.542 Å), with the Bragg angle ranging from 2° to 70°. Mg and Al contents of the samples were determined using an atomic absorption (Varian AA 220, including Zeeman) instrument after dissolving the samples in nitric acid. C, H and N contents were determined using a Perkin-Elmer model 240B elemental analyzer. Thermogravimetric (TG) analysis was carried out on a Mettler-Toledo TGA 851e apparatus at a heating rate of 10°C min−1 under a nitrogen atmosphere. The morphology of the nanohybrids were observed using scanning electron microscopy (SEM; LEO 440 i).
2.3. Drug release studies
The release studies have been carried out on a Shimadzu 1601 UV–Vis Spectrometer as follows: a phosphate-buffered solution (0.2 M) at pH 7.4 was employed as a release medium. The release of the drug from olsalazine–Mg–Al–NO3 LDH nanohybrids into the media was performed by soaking 100 mg of nanohybrid in 250 mL of buffer solution while continuously stirring (at a speed of 150 rpm) at a temperature of 37 ± 0.1°C. At specific time intervals, 5 mL of solution was removed and immediately replaced with an equal volume of fresh buffer to keep the volume constant. The removed solution was measured by UV–Vis spectrophotometer at 254 nm on a UV–Vis Shimadzu spectrometer Citation19. For comparison, the release test of the physical mixture of LDH and olsalazine was also processed.
3. Results and discussion
3.1. Characterisation of samples
The XRD pattern for the LDH precursor and the LDH with the intercalated drug showed characteristic reflections corresponding to a crystalline layered phase () Citation17,Citation20. In the range of 2θ = 2–70° for the Mg–Al–NO3 LDHs (), the sharp and strong diffraction peaks at low 2θ values, shown in , indicate good crystallinity of LDH nanoparticles. No characteristic peaks from any other impurity were observed. 2θ = 60° is also characteristic of hydrotalcite structure. The results are consistent with those of previous reports, indicating that we obtained the layered structure of LDH while keeping the ion-exchange ability. The strong positions of crystallographic indices (0 0 3), (0 0 6) and (1 1 0) allow for the calculation of lattice distances Citation21. The interlayer distance value of d 0 0 3, representing the summation of thickness of the brucite-like layer (0.48 nm) Citation21 and the gallery height, is a function of the number, size and orientation of intercalated anions Citation19.
Figure 1. The XRD patterns of (a) LDH–NO3 precursor; (b) LDH with intercalated olsalazine prepared by coprecipitation and (c) LDH with intercalated olsalazine prepared by ion exchange.
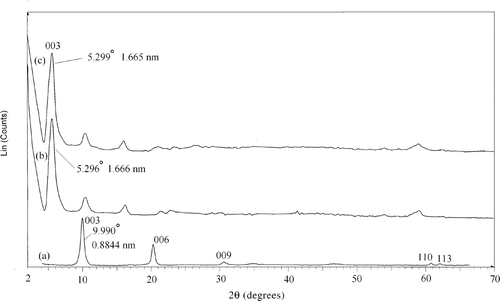
The XRD patterns of the samples with the intercalated drug prepared by coprecipitation and anion exchange are shown in , respectively. During ion exchange of the nitrate anions, the layers of LDH expand to host the drug anions and this expansion is reflected by the value of d 0 0 3. The values of d 0 0 3 are 1.666 nm for drug–LDHs prepared by the coprecipitation method and 1.665 nm for drug–LDHs prepared by the anion-exchange method (). This swelling of the layers is due to the intercalation of the olsalazine molecules and the basal spacing corresponding to diffraction by planes (0 0 3) which were much larger than for the LDH. Taking into account that the thickness of LDH layer is ∼4.8 Å and the longitudinal van der Waals’ radius of the olsalazine anion is 11.741 Å (determined by the software ChemSketch), it was confirmed that olsalazine molecules were successfully intercalated in their anionic form in both preparation methods Citation13,Citation18, and that they were arranged as a monolayer within the interlayer.
Olsalazine intercalation by the LDH was also confirmed by FT-IR spectroscopy. The FT-IR spectra of the Mg–Al–NO3 LDH precursor, olsalazine in pure form and the olsalazine-intercalated LDH are shown in .
Figure 2. The FT-IR spectra of samples: (a) Mg–Al–NO3 LDH precursor; (b) olsalazine and (c) LDH–olsalazine.
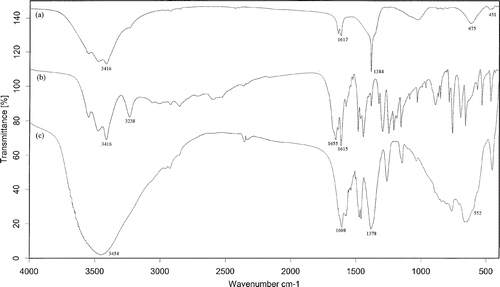
The spectrum for Mg–Al–NO3 LDH is similar to that reported in the literature Citation22,Citation23. Absorption at 1384 cm−1can be assigned to the υ3 vibration of NO3. A broad, strong absorption band centred at 3416 cm−1 is attributed to the stretching vibrations of hydroxyl groups and surface and interlayer water molecules Citation24,Citation25, which are found at a lower frequency in LDHs compared with the O–H stretch in free water at 3600 cm−1 Citation26. This is attributed to the formation of hydrogen bonds between the interlayer water and the different guest anions as well as with the hydroxide groups of the layers. A weaker band at 1617 cm−1was due to the bending mode of water molecules. The bands centred at 675 and 451 cm−1 are attributed to Al–O and Mg–O lattice vibrations Citation22.
In the case of pure olsalazine, strong absorption bands centred at 3416 and 3238 cm−1are attributed to the stretching vibrations of OH and NH groups. Furthermore, absorption bands at 1655, 1615 and 1480 cm−1 are related to the C=O, C=C (aromatic rings) and azo functional groups, respectively.
After the intercalation of olsalazine, the band at 1655 cm−1 due to the COOH group disappears, while the two bands at ca 1608 and 1378 cm−1 due to the anti-symmetric and symmetric stretching vibrations of –appear and shift to lower wavenumbers, compared to free –
in olsalazine, indicating that the intercalation of olsalazine in the interlayer space involves hydrogen bonding, besides the obvious electrostatic attraction between the electropositive cations in layer and organic anions in interlayer Citation19,Citation27,Citation28. In addition, there was an absorption band at 1460 cm−1 attributed to the stretching of azo group, which is found at a lower frequency in olsalazine–LDH nanohybrids compared with the azo stretching in free olsalazine at 1480 cm−1, due to the tautomerism in hydroxyazo dyes.
The thermal stabilities of pure olsalazine, Mg–Al–NO3 LDH and olsalazine-intercalated LDH are investigated using TG analyses. The TG–DTG profiles are depicted in .
Figure 3. TG–DTG thermograms of (a) olsalazine; (b) Mg–Al–NO3 LDH precursor and (c) olsalazine-intercalated LDH.
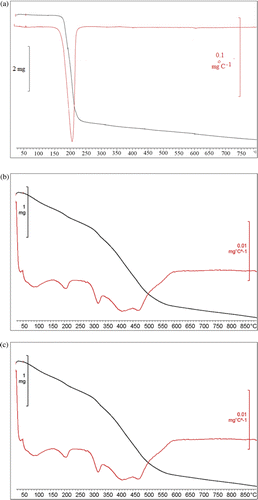
In the case of pure olsalazine, there is only one step in the temperature range 150–230°C that is attributed to the decomposition of olsalazine. shows the TG and DTG curves obtained for Mg–Al–NO3 LDH precursor. Decomposition of Mg–Al–NO3 LDH precursor occurred in the four steps. The first step observed in the range of 50–160°C is due to adsorbed and interlayer water in Mg–Al–NO3 LDH. The second and third steps observed in the ranges 120–360°C and 360–400°C were mainly attributable to the dehydroxylation of the brucite-like octahedral layers in Mg–Al–NO3 LDH. Finally, the fourth step observed above 400°C is due to the elimination of intercalated in the Mg–Al–NO3 LDH interlayers Citation29. As seen in , the TG–DTG curves of olsalazine-intercalated LDH reveal five distinguishable weight loss steps. In the olsalazine-intercalated LDH, the first and second steps in the temperature range 50–210°C are attributed to the loss of the surface adsorbed and the interlayer water. The mass loss which follows (210–340°C) is due to the removal of residual intercalated water and to trace dehydroxylation of the LDH layer. The fourth and fifth mass losses of 340–500°C correspond to the decomposition of intercalated olsalazine under an N2 atmosphere. It should be noted that this temperature region is obviously higher than that of the pure free olsalazine, suggesting that the thermal stability of organic olsalazine in LDH–olsalazine is clearly enhanced due to the host–guest interaction involving the hydrogen bond demonstrated previously by IR analysis.
lists the chemical compositions of all samples. The samples were analysed for their Mg and Al contents for the composition of the high temperature layers and for their C, H and N contents for the composition of the interlayer region. It should be noted that the determination of the chemical compositions of the intercalated materials is due to the results of elemental analysis, TG analysis (amount of water), the rule of charge balance and the charge on the ion of the interlayer guests. Since no nitrate was determined to be present in the drug–LDH nanohybrids, complete charge balancing was assumed between each Al atom in the LDH sheets and in the negative charge of the olsalazine anions Citation17.
Table 1. Results from element chemical analysis.
The molar C/N ratio in samples containing olsalazine coincided with the value for the pure drug, indicating that no contamination from atmospheric carbon dioxide has taken place in these samples. Consequently, the amount of the drug has been determined from the element chemical analysis values for the samples Citation13,Citation18. The SEM images of NO3–LDH and olsalazine–LDH are shown in . As can be seen in , NO3–LDH has a hexagonal, plate-like shape when observed using SEM. With olsalazine intercalated into the interlayer gallery, the LDH nanoparticle shape changes from a regular hexagon and the particles’ well-formed, layered shapes are clearly observed (images b and c), suggesting that the intercalation of drug anions influences the LDH crystallisation behaviour.
Figure 4. SEM images of (a) NO3–LDH; (b) olsalazine–LDH, prepared via the coprecipitation method and (c) olsalazine–LDH, prepared by the ion-exchange method.
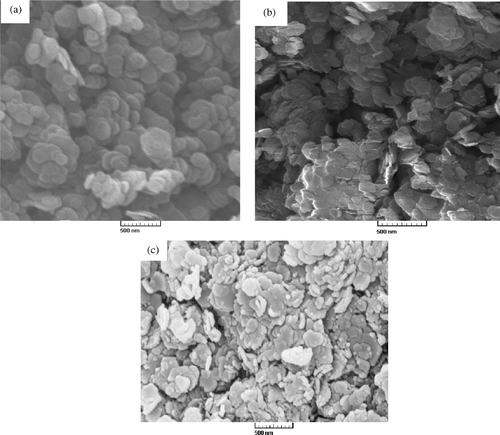
A possible schematic representation for intercalation of the olsalazine in LDH is shown in .
On the basis of the basal spacing d 0 0 3 of 1.666 nm for the olsalazine–LDH observed by XRD, and after subtracting the thickness of the brucite layer (0.48 nm), the gallery height is calculated to be 1.174 nm. The enlargement of the interlayer distance is caused by the exchange of the larger olsalazine anions with the nitrate ones.
3.2. Release study
The release of olsalazine–LDH nanohybrids was monitored via UV–Vis spectrophotometer. shows the curves of release for samples of the physical mixture of olsalazine and LDH and the olsalazine–LDH nanohybrid in a simulated phosphate buffer solution at a pH of 7.4.
For the physical mixture, the total drug content was immediately released in the initial 15 min at a pH of 7.4. In the initial 20 min, the release of the drug from the nanohybrids was a slow and persistent process and the release percentage of olsalazine from the nanohybrids reached 23%, 65%, 85% and 88% for olsalazine–LDH prepared via the coprecipitation method after 20, 60, 120 and 240 min, respectively, at a pH of 7.4. Probably, the slow release step may be due to an ion-exchange process between the intercalated drug anions in the interlayer and the phosphate anions in the solution Citation13,Citation19,Citation30. The release behaviour of olsalazine–LDH prepared via coprecipitation method and ion-exchange method does not indicate significant differences.
On the basis of the release curve, it can be determined that the equilibrium percentage of olsalazine released does not reach 100%. This is probably due to the characteristics of the ion-exchange reaction Citation13,Citation31,Citation32, i.e. this is an equilibrium process and the interlayer anions cannot be exchanged completely. In order to confirm this result, the solid residues after the dissolution studies were dried and their powder XRD diagrams were recorded (). The XRD diagrams of residues when compared with those of the olsalazine–LDHs show a reduction in the d 0 0 3 value from 1.66 to 1.05 nm for both nanohybrids (prepared by coprecipitation and ion exchange), which is quite similar to the d 0 0 3 value of phosphate-containing LDHs Citation19.
This behaviour is similar to that of the captopril-intercalated Mg–Al LDHs as reported by Xue Duan and co-workers Citation19. This suggests that the anion exchange between the drug-intercalated anions and the phosphate anions in the buffer may occur during the release process.
4. Conclusion
Olsalazine-intercalated Mg–Al LDHs with an Mg/Al ratio of 1.94 as a drug-inorganic nanohybrid has been successfully synthesised by coprecipitation or anion-exchange techniques. The XRD, FT-IR, UV–Vis spectroscopy, TG and atomic absorption measurements show that the original interlayer nitrate anions of hydrotalcite can be replaced by olsalazine anion. The larger interlayer spacing of 1.665–1.666 nm for olsalazine-intercalated LDHs determined by XRD analyses than the molecular size of olsalazine (1.174 nm) suggests a vertical arrangement of the olsalazine anion between the layers. In addition, on the basis of the expansion of the interlayer distance value of d 0 0 3 in the olsalazine–LDH nanohybrid, we conclude that the olsalazine anion was arranged as a monolayer within the interlayer.
The TG analyses indicate that the thermal stability of organic olsalazine in the LDH–olsalazine nanohybrid is clearly improved due to the host–guest interaction involving the hydrogen bonds. The release study demonstrates that the release rate of the olsalazine–LDH nanohybrid is much slower than when the same matrix is used simply mixed with the drug. XRD analyses for the samples recovered from release media indicate that the ion-exchange mechanism is mainly responsible for the release behaviour of drug–LDH at a pH of 7.4.
Acknowledgement
Financial support from Payam Noor University research council is greatly appreciated.
References
- Nakayama , H , Kuwano , K and Tsuhako , M . 2008 . Controlled release of drug from cyclodextrin-intercalated layered double hydroxide . J. Phys. Chem. Solids , 69 : 1552 – 1555 .
- Playle , AC , Gunning , SR and Llewellyn , AF . 1974 . The in vitro antacid and anti-pepsin activity of hydrotalcite . Pharm. Acta Helv. , 49 : 298 – 302 .
- Ookubo , A , Ooi , K and Hayashi , H . 1992 . Hydrotalcites as potential adsorbents of intestinal phosphate . J. Pharm. Sci. , 81 : 1139 – 1140 .
- Nakayama , H , Takeshita , K and Tsuhako , M . 2003 . Preparation of 1-hydroxyethylidene-1,1-diphosphonic acid-layered double hydroxide nanocomposite and its physicochemical properties . J. Pharm. Sci. , 92 : 2428 – 2435 .
- Zhao , H and Vance , GF . 1997 . Intercalation of carboxymethyl-β-cyclodextrin into magnesium-aluminum layered double hydroxide . J. Chem. Soc., Dalton Trans. , 11 : 1961 – 1965 .
- Choy , JH , Jung , EY , Son , YH and Park , M . 2004 . The orientation of anionic β-cyclodextrin in the interlayer space of Zn/Al layered double hydroxide . J. Phys. Chem. Solids , 65 : 509 – 512 .
- Bringley , FJ and Liebert , NB . 2003 . Controlled chemical and drug delivery via the internal and external surfaces of layered compounds . J. Dispersion Sci. Technol. , 24 : 589 – 605 .
- Choy , JH , Kwak , SY , Jeong , YJ and Park , JS . 2000 . Inorganic layered double hydroxides as nonviral vectors . Angew. Chem. Int. Ed. , 39 : 4042 – 4045 .
- Tillement , O . 1994 . Solid-state ionics electrochemical devices . Solid State Ionics , 68 : 9 – 33 .
- Clearfield , A . 1993 . Ion exchange and adsorption in layered phosphates . Mater. Chem. Phys. , 35 : 257 – 265 .
- Technical Specifications Bulletin, SORBACID-911, Sud-Chemie AG, 2002.
- Leroux , F and Besse , JP . 2001 . Polymer interleaved layered double hydroxide: A new emerging class of nanocomposites . Chem. Mater. , 13 : 3507 – 3515 .
- Xia , SJ , Ni , ZM , Xu , Q , Hu , BX and Hu , J . 2008 . Layered double hydroxides as supports for intercalation and sustained release of antihypertensive drugs . J. Solid State Chem. , 181 : 2610 – 2619 .
- Mahkam , M , Mohammadi , R and Ranaei-Siadat , SO . 2006 . Synthesis and evaluation of biocompatible pH-sensitive hydrogels as colon-specific drug delivery systems . J. Chin. Chem. Soc. , 53 : 727 – 733 .
- Mahkam , M , Assadi , MG , Zahedifar , R , Ramesh , M and Davaran , S . 2004 . Linear type azo-containing polyurethanes for colon specific drug delivery . J. Bioact. Compat. Polym. , 19 : 45 – 53 .
- Ren , L , Hu , JS , Wan , LJ and Bai , CL . 2007 . A simple method to synthesize layered double hydroxide nanoscrolls . Mater. Res. Bull. , 42 : 571 – 575 .
- Ay , AN , Karan , BZ and Temel , A . 2007 . Boron removal by hydrotalcite-like, carbonate-free Mg–Al–NO3-layered double hydroxide and a rationale on the mechanism . Microporous Mesoporous Mater. , 98 : 1 – 5 .
- del Arco , M , Fernández , A , Martín , C and Rives , V . 2009 . Release studies of different NSAIDs encapsulated in Mg, Al, Fe-hydrotalcites . Appl. Clay Sci. , 42 : 538 – 544 .
- Zhang , H , Zou , K , Guo , S and Duan , X . 2006 . Nanostructural drug-inorganic clay composites: Structure, thermal property and in vitro release of captopril-intercalated Mg–Al–layered double hydroxides . J. Solid State Chem. , 179 : 1792 – 1801 .
- Kustrowski , P , Sulkowska , D , Chmielarz , L , Rafalska-Lasocha , A , Dudek , B and Dziembaj , R . 2005 . Influence of thermal treatment conditions on the activity of hydrotalcite-derived Mg–Al oxides in the aldol condensation of acetone . Microporous Mesoporours Mater. , 78 : 11 – 22 .
- X. Duan and D.G. Evans (eds.), Layered Double Hydroxides, Springer-Verlag, Berlin/Heidelberg, 2006.
- Li , C , Wang , G , Evans , DG and Duan , X . 2004 . Incorporation of rare-earth ions in Mg–Al layered double hydroxides: Intercalation with an [Eu(EDTA)]− chelate . J Solid State Chem. , 177 : 4569 – 4575 .
- Klopprogge , JT , Hickey , L and Frost , JL . 2001 . Heating stage Raman and infrared emission spectroscopic study of the dehydroxylation of synthetic Mg-hydrotalcite . Appl. Clay Sci. , 18 : 37 – 49 .
- Wei , M , Xu , X , He , J , Yuan , Q , Rao , G , Evans , DG , Pu , M and Yang , L . 2006 . Preparation and thermal decomposition studies of l-tyrosine intercalated MgAl, NiAl and ZnAl layered double hydroxides . J. Phys. Chem. Solids. , 67 : 1469 – 1476 .
- Cavani , F , Trifiro , F and Vaccari , A . 1991 . Hydrotalcite-type anionic clays: Preparation, properties and applications . Catal. Today , 11 : 173 – 301 .
- Nakamoto , K . 1997 . Infrared and Raman Spectra of Inorganic and Coordination Compounds, , 5th , New York : Wiley .
- Santosa , SJ , Sri Kunarti , E and Karmanto , E . 2008 . Synthesis and utilization of Mg/Al hydrotalcite for removing dissolved humic acid . Appl. Surf. Sci. , 254 : 7612 – 7617 .
- Gunzler , H and Gremlich , HU . 2002 . IR Spectroscopy: An Introduction , Weinheim : Wiley–VCH .
- Kameda , T , Fubasami , Y , Uchiyama , N and Yoshioka , T . 2010 . Elimination behavior of nitrogen oxides from a NO3-intercalated Mg–Al layered double hydroxide during thermal decomposition . Thermochim. Acta. , 499 : 106 – 110 .
- Gu , Z , Thomas , AC , Xu , ZP , Campbell , JH and Qing (Max) Lu , G . 2008 . In vitro sustained release of LMWH from MgAl-layered double hydroxide nanohybrids . Chem. Mater. , 20 : 3715 – 3722 .
- Fogg , AM , Dunn , JS , Shyu , SG , Cary , DR and O’Hare , D . 1998 . Selective ion-exchange intercalation of isomeric dicarboxylate anions into the layered double hydroxide [LiAl2(OH)6]Cl · H2O . Chem. Mater. , 10 : 351 – 355 .
- Fogg , AM , Dunn , JS and O’Hare , D . 1998 . Formation of second-stage intermediates in anion exchange intercalation reactions of the layered double hydroxide [LiAl2(OH)6]Cl · H2O as observed by time-resolved, in situ x-ray diffraction . Chem. Mater. , 10 : 356 – 360 .