Abstract
Here, Syzygium cumini leaf extract (LE) and seed extract (SE) were explored for the synthesis of gold nanoparticles (GNP). LE and SE as well as their polar (water) fractions showed potential for GNP synthesis. Comparative synthesis kinetics and morphological characterisation studies revealed the synthesis of smaller sized GNP by LE than SE. Only polar (water) fractions showed potential for GNP synthesis, which are smaller in size compared to their respective extracts. SE contained more polyphenols and biochemical constituents than LE and therefore, showed higher synthesis rate and bigger sized GNP. Atomic force microscope and scanning electron microscope analysis indicated that both extracts and their fractions catalysed the synthesis of spherical GNP. The average size of GNP synthesised by LE, leaf water fraction (LWF), SE and seed water fraction (SWF) were 24, 23, 35 and 32 nm, respectively. Fourier transform infrared analysis identified the biomolecules involved in the synthesis and stability of GNP. This study documented the potential of S. cumini for the synthesis of GNP in addition to silver nanoparticles (SNP). However, nature and types of polyphenols involved in GNP synthesis seem to be different from that involved in SNP synthesis. This might be the possible reason for smaller sized GNP that SNP.
1. Introduction
In recent times, metallic nanoparticles have been extensively synthesised for their wide applications. Attention has been paid to synthesise a variety of nanoparticles with desired properties either by modification of existing procedures or by development of new methods Citation1. Gold nanoparticles (GNP) are the most stable metal nanoparticles Citation2. Among various synthesis methods, plant-mediated synthesis is preferred due to its cost effectiveness and environment-friendly nature Citation3,Citation4. GNP have applications in therapeutic Citation5, catalysis Citation2, immunoassay and immunosensing Citation6, diagnostic and drug delivery Citation7–10, analyte sensing Citation11–13, cancer cell diagnostics and selective photothermal therapy Citation14,Citation15, photoacoustic imaging Citation16,Citation17 and cancer treatment Citation18. Various plant extracts have been explored for the synthesis of GNP, namely tamarind Citation19, Aloe vera Citation20, Pelargonium graveolens Citation21, Diopyros kaki Citation22, Eucalyptus camaldulensis and Pelargonium roseum Citation23, coriander Citation24, Scutellaria barbata Citation25, lemongrass Citation26, Datura metel, Carica papaya and Solanum melongena Citation27. Additionally, live plants like Brassica juncea Citation28, Avena sativa Citation29, Medicago sativa Citation30 and Chilopsis linearis Citation31 have also been used for GNP synthesis.
Different plants have differential potential for metallic nanoparticle synthesis in terms of size and shape Citation3,Citation22,Citation27. We have recently reported on the Syzygium cumini leaf extract (LE) and seed extract (SE) mediated biosynthesis of silver nanoparticles (SNP) and their characterisation Citation4. In this study, we report on the extracellular green synthesis of GNP by LE and SE of S. cumini plant. Different polarity-based fractions of LE and SE have also been explored for GNP synthesis. The GNP size distribution was characterised with scanning electron microscope (SEM) and atomic force microscope (AFM). Further, high performance liquid chromatography (HPLC) and Fourier transform infrared (FTIR) analyses were performed to characterise the biomolecules of S. cumini in various extracts/fractions involved in the synthesis and stability of GNP.
2. Experimental
2.1. Materials
The HAuCl4 was purchased from Sigma–Aldrich, USA; n-hexane, chloroform, ethyl acetate, butanol, methanol and acetonitrile were purchased from Merck, India. All other chemicals used in the study were of analytical grade. S. cumini leaves and seed were collected from IHBT, Palampur.
2.2. GNP synthesis reaction
LE, SE and their fractions were prepared as described in our previous study Citation4. Briefly, fresh leaves/seeds of S. cumini were thoroughly washed, dried and ground to fine powder. Dry powder was suspended in deionised Milli-Q water, vigorously vortexed and incubated overnight at room temperature. The suspension was centrifuged and the filtered solution was used for further analysis. To analyse the nature of biomolecules involved in the synthesis of GNP, the LE and SE were fractionated on the basis of polarity by solvent–solvent partitioning with n-hexane, chloroform, ethyl acetate, butanol and water to obtain corresponding fractions. All fractions along with LE and SE were tested for GNP synthesis. For GNP synthesis reaction, 1.0 mM aqueous HAuCl4 solution was incubated with 10% (v/v) of LE, SE and their polar fractions for 24 h at room temperature. The change in colour of the whole solution with increase in incubation time was an indication of GNP synthesis. The reaction suspension was centrifuged at 10,000 rpm for 10 min to purify the GNP. These GNP were washed thrice with water and stored as lyophilised powder for characterisation.
2.3. Spectroscopic analysis and synthesis kinetics of GNP
The absorption spectra for each reaction mixture including LE, SE and their polar (water) fractions were recorded between 450 and 700 nm by taking 2 µL of solution at different incubation time intervals (0, 2, 4, 8, 12 and 24 h). The kinetics of each synthesis reaction was studied using UV–vis scan on nanodrop ND1000 as a function of time. The synthesis kinetic graphs were generated as a function of time versus maximum intensity at ∼530 nm.
2.4. Morphological characterisation of GNP by SEM and AFM
Purified GNP in suspension were characterised for their morphology using Hitachi S-3400N SEM and Veeco diNanoscope 3D AFM. Clear, well-dispersed water suspended GNP solution was placed on to the carbon tape mounted on aluminium stub for SEM imaging. The samples were dried at room temperature in control environment. The images were captured on SEM mode at desired magnification. GNP suspension was spread on a well-cleaned glass cover slip mounted on AFM stub, and was dried with nitrogen flow at room temperature. Images were obtained in tapping mode using a silicon probe cantilever of 125 µm length, resonance frequency 209–286 kHz, spring constant 20–80 Nm−1 and nominal tip radius 5–10 nm. The scan rate used was 1 Hz. A minimum of three images for each sample were obtained with AFM and analysed to ensure reproducible results.
2.5. FTIR analysis
The biomolecules in extracts and fractions of S. cumini involved in GNP synthesis were characterised with Thermo Nicolet 6700 FTIRspectroscope (Thermo, USA). For this, FTIR spectra of GNP powder in KBr pellets were recorded. To obtain good signal-to-noise ratio, 256 scans of GNP were taken in the range 400–4000 cm−1 and the resolution was kept as 4.0 cm−1.
3. Results and discussion
The potential of plant extracts to reduce various metal ions into corresponding nanoparticles forms has been well documented Citation3,Citation22–26. Towards this effort, we have recently explored the potential of S. cumini for the synthesis of SNP. We have also characterised the biomolecules of S. cumini involved in SNP synthesis Citation4. In this study, we explored the LE and SE of the same plant S. cumini for GNP synthesis from aurochloric acid (HAuCl4). Also, the GNP synthesis potential of various polarity-based fractions of LE and SE was analysed. The biochemical constituents of plant extracts have been reported for their effect on size and shape of nanoparticles synthesised Citation4,Citation32. The effects of LE and SE on GNP synthesis were similar to that reported for SNP synthesis. However, GNP were smaller in size compared to SNP Citation4 synthesised by either of the extracts and fractions. Similar to SNP synthesis Citation4, the polyphenolic content was also found to be directly correlated with the rate of GNP synthesis and size distribution.
3.1. Polarity-based fractionation for the identification of biomolecules involved in GNP synthesis and stability
We followed a similar procedure in this study as reported during SNP synthesis Citation4. Dried tissue was used for the extraction in water at room temperature to maintain homogeneous phytochemical composition throughout the study. Polarity-based fractionations were also carried out in the similar way as explained earlier Citation4. HPLC profiling of LE, leaf water fraction (LWF), SE and seed water fraction (SWF) was conducted for the identification of biomolecules responsible for GNP synthesis and stability (data not shown; for details see our previous publication) Citation4.
3.2. Kinetics of GNP synthesis
Metallic nanoparticles have been reported earlier for their size-dependent collective oscillation of the conductance electron at different wavelengths. Appearance of specific colour during synthesis was due to excitation of surface plasmon resonance of GNP. With increase in incubation time, the reduction of HAuCl4 into GNP was evident from change in the reaction mixture colour and appearance of GNP characteristic absorbance peak at ∼550 nm Citation33. The involvement of biochemical constituents such as redox species, capping agents and surfactants of plant extracts has been documented earlier for metallic nanoparticle synthesis and for their size and shape control Citation20,Citation21. Here, we have studied the potential of LE, SE and their different fractions to synthesise GNP. Only LE, SE and their corresponding water fractions showed the potential for GNP synthesis. Other less polar fractions did not show GNP synthesis potential. The colours of solutions containing GNP synthesised by LE and SE were different. GNP synthesised by LE and LWF were greenish red in colour (data not shown), while, GNP synthesised by SE and SWF were ruby red in appearance (data not shown). Most of the ingredients responsible for GNP synthesis were water soluble and hence water fractions have GNP synthesis potential. The biological constituents separated by other less polar solvents could not synthesise GNP. Similar fractions have been documented earlier for the synthesis of SNP Citation4. Results suggest that similar kinds of biomolecules are responsible for the synthesis and stability of both SNP and GNP.
UV–vis absorbance spectroscopy data revealed the higher GNP synthesis potential of SE than LE (). The peak representing the synthesis of GNP in this study was at the same position as that of GNP synthesised with citrate reduction method. The absorption peaks obtained for GNP synthesis with citrate method in various studies were found to be near 550 nm Citation34–36. The time-dependent synthesis kinetics confirmed that most of the GNP syntheses were completed within 2 h of incubation at room temperature (). Hence, GNP synthesis was faster compared to SNP which was completed in 8 h Citation4. Higher amounts of biochemicals in SE than LE could be responsible for higher ability of SE for GNP synthesis. However, loss of biochemical constituents during fractionation may be responsible for lesser GNP synthesis potential of corresponding water fractions than extracts (). With increase in incubation time, quantitatively more GNP were synthesised, as evinced by increase in colour intensity (data not shown) and peak intensity ().
Figure 1. Synthesis kinetics of GNP synthesised by S. cumini extracts and fractions. (a) UV–vis spectra recorded as a function of time of reaction. The synthesis of GNP by S. cumini LE, SE, LWF and SWF was monitored between 0 and 24 h and (b) UV–vis absorption spectra of GNP taken after 2 h incubation of LE, SE, LWF and SWF with 1.0 mmol L−1 HAuCl4.
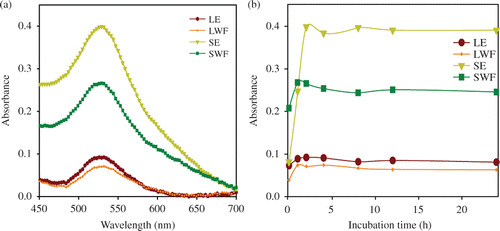
3.3. Morphological characterisation of GNP
SEM and AFM were used morphological characterisation of GNP. These techniques have been used earlier for nanoparticle characterisation Citation3,Citation4,Citation20. The initial morphological characterisation with SEM revealed that GNP synthesised by various active extracts/fractions were spherical in shape (). Detailed shape and size characterisation of GNP was carried out with AFM. The GNP synthesised by LE ( and LWF () were 24 and 23 nm, respectively, while the size of GNP synthesised by SE ( and SWF () were 35 and 32 nm, respectively. The size of GNP synthesised by water fractions of both LE and SE was reduced.
Figure 3. (Colour online) Tapping mode AFM images of GNP synthesised with (a) LE; (b)LWF; (c) SE; and (d) SWFs of S. cumini. Note: For imaging, AFM was set at 2 × 2 µm2 scale. Size distribution of synthesised GNP is shown at the bottom of each image.
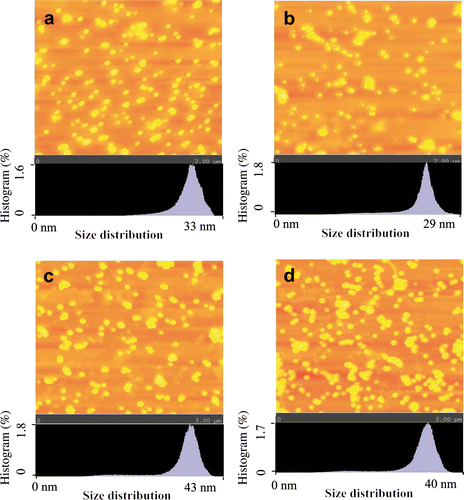
Figure 4. (a) Total polyphenols content of S. cumini LE, LWF, SE and SWFs. (b) Sizes of GNP synthesised by S. cumini LE, LWF, SE and SWFs.
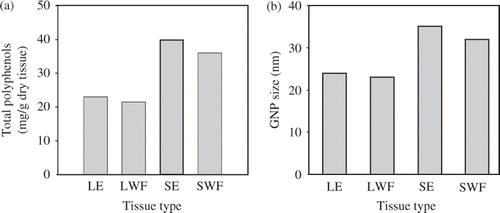
This size of GNP synthesised by various extracts and fractions was directly correlated with respective polyphenolic contents (). Similar correlation between size of nanoparticles and polyphenolic contents has been documented through previous studies. The reduction in active species like polyphenolic content in the reaction mixture, reduces the synthesis rate and nucleation process Citation4,Citation22,Citation37. As the biochemical constituents vary from plant to plant and even within different tissues of a plant in concentration and type, different types of plant extracts directed nanoparticles with different sizes and shapes.
The size of metallic nanoparticles is mainly determined by the rate of reaction and involvement of surfactants/capping agents. When HAuCl4 is reduced to Au, Au undergoes nucleation to form GNP in the presence of surfactant/capping agent. The balance between reduction speed and surfactant/capping amount directs well-dispersed GNP Citation4,Citation37. More redox species and less capping agents in SE than LE might be responsible for bigger sized GNP. During fractionation, more reducing molecules were separated out compared with surfactants; thus, the size of GNP was reduced. Similar reduction in SNP size was observed upon fractionation Citation4.
3.4. Direct relation of GNP size with polyphenols and biochemical constituents of different extracts and fractions used for GNP synthesis
Polyphenolic compounds have earlier been reported in some other plant to play important role in the synthesis of GNP Citation37. The major ingredients of LE and SE (in present extraction) as well as their water fractions were polyphenolic compounds as reported in our previous study Citation4 The polyphenolic contents of LE, SE, LWF and SWF were experimentally determined to be 23, 40, 21 and 36 mg g−1 dry tissues, respectively (). Total polyphenol content was reduced after fractionation suggesting their separation along with other constituents. The size of GNP synthesised with water fractions of LE and SE was also reduced () in a fashion similar to polyphenolic content. This reveals the direct correlation between the concentration of polyphenols and size of GNP. However, capping agents/surfactant also play a crucial role in governing size of GNP. Further, the larger size of GNP synthesised with SE could be due to greater polyphenolic contents of the SE than LE. The trend of GNP was similar to that of SNP reported in our recent study Citation4. But GNP were smaller in size compared to SNP synthesised with extracts/fractions.
A well-established citrate reduction method has been reported previously for the synthesis of GNP. Further, the control over shape and size of GNP with citrate method has been documented. Using the second pathway, a new synthetic route for the synthesis of nearly monodisperse GNP in the size range from 20 to 40 nm by simply varying the solution pH with fixed concentrations of HAuCl4 and sodium citrate has also been documented Citation34–36. However, the use of GNP synthesised through citrate method has now been found to be harmful. Presence of reducing agent sodium citrate has caused cytotoxicity and has affected the viability and proliferation of the human alveolar type-II-like cell lines A549 and NCIH441 in vitro Citation38. Therefore, the GNP synthesised in this study through green process, where plant extract was used, would be safe to use for various applications.
3.5. FTIR analysis identified GNP-associated molecules
To identify GNP-associated molecules, FTIR spectra were recorded as shown in . The spectra were obtained by making KBr disc with GNP synthesised using LE (), LWF (), SE ( and SWF (). The peaks at 1000–1200 cm−1 correspond to C–O/C–OH single bonds of alcohols Citation39,Citation40 and phenols Citation41. The absorbance peak at 1055–1066 cm−1 corresponds to C–N stretching vibration of aliphatic amines or to alcohols/phenols Citation22. The bands in 1315–1380 cm−1 range indicate the OH deformation vibrations in the aromatic ring/phenol Citation42,Citation43. The peak at 1619–1638 cm−1 corresponds to C=C groups or aromatic rings of polyphenols Citation41,Citation44 or carbonyl groups Citation44. The peak at 3407–3433 cm−1 corresponds to OH group of polyphenols Citation39. Results suggest that the biomolecules of S. cumini LE and SE involved in the GNP synthesis are largely polyphenols.
Figure 5. FTIR spectra of S. cumini synthesised GNP. Note: The GNP synthesised using different extracts and fractions were powdered and washed with double-distilled water before use in KBr disc for FTIR spectrum. (a) FTIR spectrum of LE-synthesised GNP; (b) FTIR spectrum of LWF-synthesised GNP; (c) FTIR spectrum of SE synthesised GNP; and (d) FTIR spectrum of SWF-synthesised GNP.
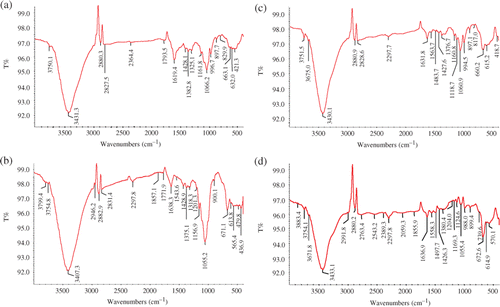
4. Conclusion
This study documented the potential of S. cumini for the synthesis of GNP in addition to SNP. The nature and quantity of polyphenols in the extracts/fractions were found to be essential for determining the synthesis rate and size distribution of GNP. However, types of polyphenols involved in GNP synthesis seem to be differing from that involved in SNP synthesis. Additionally, S. cumini showed differential behaviour in redox potential for silver and gold ions. These might be the possible reasons for smaller sized GNP that SNP synthesised using the same S. cumini LE and SE under the same reaction conditions.
Acknowledgements
We thank Dr P.S. Ahuja, Director IHBT (CSIR) for his continuous encouragement. The authors thank Council of Scientific and Industrial Research (CSIR), Government of India, New Delhi for financial support. Vineet Kumar thanks Council of Scientific and Industrial Research (CSIR), Government of India, New Delhi for providing senior research fellowship (SRF). IHBT communication number for this article is 2152.
References
- Egorova , EM and Revina , AA . 2000 . Synthesis of metallic nanoparticles in reverse micelles in the presence of quercetin . Colloids Surf., A , 168 : 87 – 96 .
- Daniel , MC and Astruc , D . 2004 . Gold nanoparticles: Assembly, supramolecular chemistry, quantum-size-related properties, and applications toward biology, catalysis, and nanotechnology . Chem. Rev. , 104 : 293 – 346 .
- Kumar , V and Yadav , SK . 2009 . Plant-mediated synthesis of silver and gold nanoparticles and their applications . J. Chem. Technol. Biotechnol. , 84 : 151 – 157 .
- Kumar , V , Yadav , SC and Yadav , SK . 2010 . Syzygium cumini leaf and seed extract mediated biosynthesis of silver nanoparticles and their characterization . J. Chem. Technol. Biotechnol. , 85 : 1301 – 1309 .
- Mukherjee , P , Bhattacharya , R , Bone , N , Lee , YK , Patra , CR , Wang , S , Lu , L , Secreto , C , Banerjee , PC Yaszemski , MJ . 2007 . Potential therapeutic application of gold nanoparticles in B-chronic lymphocytic leukemia (BCLL): Enhancing apoptosis . J. Nanobiotechnol. , 5 : 4
- Mayer , KM , Lee , S , Liao , H , Rostro , BC , Fuentes , A , Scully , PT , Nehl , CL and Hafner , JH . 2008 . A label-free immunoassay based upon localized surface plasmon resonance of gold nanorods . ACS Nano , 2 : 687 – 692 .
- Bhumkar , DR , Joshi , HM , Sastry , M and Pokharkar , VB . 2007 . Chitosan reduced gold nanoparticles as novel carriers for transmucosal delivery of insulin . Pharm. Res. , 24 : 1415 – 1426 .
- Brown , SD , Nativo , P , Smith , JA , Stirling , D , Edwards , PR , Venugopal , B , Flint , DJ , Plumb , JA , Graham , D and Wheate , NJ . 2010 . Gold nanoparticles for the improved anticancer drug delivery of the active component of oxaliplatin . J. Am. Chem. Soc. , 132 : 4678 – 4684 .
- Huang , YF , Lin , YW , Lin , ZH and Chang , HT . 2009 . Aptamer-modified gold nanoparticles for targeting breast cancer cells through light scattering . J. Nanopart. Res. , 11 : 775 – 783 .
- Gannon , CJ , Patra , CR , Bhattacharya , R , Mukherjee , P and Curley , SA . 2008 . Intracellular gold nanoparticles enhance non-invasive radiofrequency thermal destruction of human gastrointestinal cancer cells . J. Nanobiotechnol. , 6 : 2
- Darbha , GK , Ray , A and Ray , PC . 2007 . Gold nanoparticle-based miniaturized nanomaterial surface energy transfer probe for rapid and ultrasensitive detection of mercury in soil, water, and fish . ACS Nano , 1 : 208 – 214 .
- Han , A , Dufva , M , Belleville , E and Christensen , CB . 2003 . Detection of analyte binding to microarrays using gold nanoparticle labels and a desktop scanner . Lab Chip , 3 : 329 – 332 .
- Lim , SY , Kim , JH , Lee , JS and Park , CB . 2009 . Gold nanoparticle enlargement coupled with fluorescence quenching for highly sensitive detection of analytes . Langmuir , 25 : 13302 – 13305 .
- Huang , X , El-Sayed , IH , Qian , W and El-Sayed , MA . 2006 . Cancer cell imaging and photothermal therapy in the near-infrared region by using gold nanorods . J. Am. Chem. Soc. , 128 : 2115 – 2120 .
- Mallidi , S , Larson , T , Tam , J , Joshi , PP , Karpiouk , A , Sokolov , K and Emelianov , S . 2009 . Multiwavelength photoacoustic imaging and plasmon resonance coupling of gold nanoparticles for selective detection of cancer . Nano Lett. , 9 : 2825 – 2831 .
- Chamberland , DL , Agarwal , A , Kotov , N , Fowlkes , JB , Carson , PL and Wang , X . 2008 . Photoacoustic tomography of joints aided by an Etanercept-conjugated gold nanoparticle contrast agent – an ex vivo preliminary rat study . Nanotechnology , 19 : 095101
- Xu , M and Wang , LV . 2006 . Photoacoustic imaging in biomedicine . Rev. Sci. Instrum. , 77 : 041101 – 041122 .
- Letfullin , RR , Joenathan , C , George , TF and Zharov , VP . 2006 . Laser-induced explosion of gold nanoparticles: Potential role for nanophotothermolysis of cancer . Nanomedicine (Lond) , 1 : 473 – 480 .
- Ankamwar , B , Chaudhary , M and Sastry , M . 2005 . Gold nanotriangles biologically synthesized using tamarind leaf extract and potential application in vapor sensing . Synth. React. Inorg. Met. Org. Nano Met. Chem. , 35 : 19 – 26 .
- Chandran , SP , Chaudhary , M , Pasricha , R , Ahmad , A and Sastry , M . 2006 . Synthesis of gold nanotriangles and silver nanoparticles using Aloe vera plant extract . Biotechnol. Prog. , 22 : 577 – 583 .
- Shankar , SS , Ahmad , A , Pasricha , R and Sastry , M . 2003 . Bioreduction of chloroaurate ions by geranium leaves and its endophytic fungus yields gold nanoparticles of different shapes . J. Mater. Chem. , 13 : 1822 – 1826 .
- Song , JY , Jang , HK and Kim , BS . 2009 . Biological synthesis of gold nanoparticles using Magnolia kobus and Diopyros kaki leaf extracts . Process Biochem. , 44 : 1133 – 1138 .
- Ramezania , N , Ehsanfara , Z , Shamsab , F , Aminc , G , Shahverdid , HR , Esfahanic , HRM , Shamsaiea , A , Bazazb , RD and Shahverdia , AR . 2008 . Screening of medicinal plant methanol extracts for the synthesis of gold nanoparticles by their reducing potential . Z. Naturforsch. , 63b : 903 – 908 .
- Narayanana , KB and Sakthivel , N . 2008 . Coriander leaf mediated biosynthesis of gold nanoparticles . Mater. Lett. , 62 : 4588 – 4590 .
- Wang , Y , He , X , Wang , K , Zhang , X and Tan , W . 2009 . Barbated Skullcup herb extract mediated biosynthesis of gold nanoparticles and its primary application in electrochemistry . Colloids Surf., B , 73 : 75 – 79 .
- Shankar , SS , Rai , A , Ankamwar , B , Singh , A , Ahmad , A and Sastry , M . 2004 . Biological synthesis of triangular gold nanoprisms . Nat. Mater. , 3 : 482 – 488 .
- Rajasekharreddy , P , Rani , PU and Sreedhar , B . 2010 . Qualitative assessment of silver and gold nanoparticle synthesis in various plants: A photobiological approach . J Nanopart. Res. , 12 : 1711 – 1721 .
- Marshall , AT , Haverkamp , RG , Davies , CE , Parsons , JG , Gardea-Torresdey , JL and van Agterveld , D . 2007 . Accumulation of gold nanoparticles in Brassic juncea . Int. J. Phytorem. , 9 : 197 – 206 .
- Armendariz , V , Herrera , I , Peralta-Videa , JR , Jose-Yacaman , M , Troiani , H , Santiago , P and Gardea-Torresdey , JL . 2004 . Size controlled gold nanoparticle formation by Avena sativa biomass: Use of plants in nanobiotechnology . J. Nanopart. Res. , 6 : 377 – 382 .
- Gardea-Torresday , JL , Tiemann , KJ , Gamez , G , Dokken , K , Cano-Aguilera , I , Furenlid , LR and Renner , MW . 2000 . Reduction and accumulation of Gold(III) by Medicago sativa Alfalfa biomass: X-ray absorption spectroscopy, pH, and temperature dependence . Environ. Sci. Technol. , 34 : 4392 – 4396 .
- Rodriguez , E , Parsons , JG , Peralta-Videa , JR , Cruz-Jimenez , G , Romero-Gonzalez , J , Sanchez-Salcido , BE , Saupe , GB , Duarte-Gardea , M and Gardea-Torresdey , JL . 2007 . Potential of Chilopsis linearis for gold phytomining: Using XAS to determine gold reduction and nanoparticles formation within plant tissues . Int. J. Phytorem. , 9 : 133 – 147 .
- Leela , A and Vivekanandan , M . 2008 . Tapping the unexploited plant resources for the synthesis of silver nanoparticles . Afr. J. Biotechnol. , 7 : 3162 – 3165 .
- El-Brolossy , TA , Abdallah , T , Mohamed , MB , Abdallah , S , Easawi , K , Negm , S and Talaat , H . 2008 . Shape and size dependence of the surface plasmon resonance of gold nanoparticles studied by photoacoustic technique . Eur. Phys. J. Special Topics , 153 : 361 – 364 .
- Ji , X , Song , X , Li , J , Bai , Y , Yang , W and Peng , X . 2007 . Size control of gold nanocrystals in citrate reduction: The third role of citrate . J. Am. Chem. Soc. , 129 : 13939 – 13948 .
- Pham , TTH , Cao , C and Sim , SJ . 2008 . Application of citrate-stabilized gold-coated ferric oxide composite nanoparticles for biological separations . J. Magn. Magn. Mater. , 320 : 2049 – 2055 .
- Ojea-Jimenez , I , Romero , FM , Bastus , NG and Puntes , V . 2010 . Small gold nanoparticles synthesized with sodium citrate and heavy water: Insights into the reaction mechanism . J. Phys. Chem. C , 114 : 1800 – 1804 .
- Nune , SK , Chanda , N , Shukla , R , Katti , K , Kulkarni , RR , Thilakavathi , S , Mekapothula , S , Kannan , R and Katti , KV . 2009 . Green nanotechnology from tea: Phytochemicals in tea as building blocks for production of biocompatible gold nanoparticles . J. Mater. Chem. , 19 : 2912 – 2920 .
- Uboldi , C , Bonacchi , D , Lorenzi , G , Hermanns , MI , Pohl , C , Baldi , G , Unger , RE and Kirkpatrick , CJ . 2009 . Gold nanoparticles induce cytotoxicity in the alveolar type-II cell lines A549 and NCIH441 . Part Fibre Toxicol. , 6 : 18
- Susanto , H , Feng , Y and Ulbricht , M . 2009 . Fouling behavior of aqueous solutions of polyphenolic compounds during ultrafiltration . J. Food Eng. , 91 : 333 – 340 .
- Gorinstein , S , Park , YS , Heo , BG , Namiesnik , J , Leontowicz , H , Leontowicz , M , Ham , KS , Cho , JY and Kang , SG . 2009 . A comparative study of phenolic compounds and antioxidant and antiproliferative activities in frequently consumed raw vegetables . Eur. Food Res. Technol. , 228 : 903 – 911 .
- Song , JY , Kwon , EY and Kim , BS . 2010 . Biological synthesis of platinum nanoparticles using Diopyros kaki leaf extract . Bioprocess Biosyst. Eng. , 33 : 159 – 164 .
- Polunin , KE , Sokolova , NP , Gorbunov , AM , Bulgakova , RA and Polunina , IA . 2008 . FTIR spectroscopic studies of interactions of stilbenes with silicon dioxide . Prot. Metals , 44 : 352 – 357 .
- Jokic , A , Wang , MC , Liu , C , Frenkel , AI and Huang , PM . 2004 . Integration of the polyphenol and Maillard reactions into a unified abiotic pathway for humification in nature: The role of d-MnO2 . Org. Geochem. , 35 : 747 – 762 .
- Coinceanainn , MO , Astill , C and Schumm , S . 2003 . Potentiometric, FTIR and NMR studies of the complexation of metals with theaflavin . Dalton Trans. , 5 : 801 – 807 .