Abstract
Tenoxicam is one of the potent non-steroidal anti-inflammatory drugs (NSAIDs), which is used clinically in the treatment of rheumatoid arthritis. Like other NSAIDs, Tenoxicam also suffers from the drawback of being associated with gastrointestinal side effects. Further, transdermal penetration of this drug is very poor, which circumvents the use of transdermal route. The main objective of this study is to exploit the concept of ultradeformable vesicles to increase the transport of Tenoxicam through transdermal route, and also to present it as a possible replacement for the oral NSAID therapy for rheumatoid arthritis. Three concentrations (5%, 15% and 25%) of three different surfactants (Tween-80, Span-80 and sodium desoxycholate) were used along with soya lecithin to prepare the ultradeformable vesicles. Among nine different types of vesicles, T3 vesicles (with 25% Tween-80) were found to have shown the smallest size (82.80 ± 0.74 nm), highest release in 24 h (98.01 ± 0.33%) and highest deformability (31.27 ± 0.28) and hence chosen for formulating into the gel. Among three different Carbopol® 934 concentrations (1%, 1.5% and 2%), G-2 with 1.5% carbopol was chosen as the final formulation. The formulation has shown almost twice the transdermal flux as compared to the gel with free Tenoxicam. The in vivo performance was also found to be significantly better than the oral Tenoxicam and marketed aceclofenac gel (Hifenac®). This study concluded that the gel containing Tenoxicam encapsulated in ultradeformable vesicles has a better prospective for the transdermal use to treat rheumatoid arthritis.
1. Introduction
Rheumatoid arthritis is a chronic, progressive, systemic inflammatory disorder that may affect many tissues and organs, but principally attacks the joints. Apart from other categories of drugs, non-steroidal anti-inflammatory drugs (NSAIDs) remain the most widely used class of drug for its treatment. Tenoxicam is one of the NSAIDs of oxicam class Citation1. It is very potent and useful for chronic use in rheumatoid arthritis. But as with other NSAIDs, this drug is also associated with gastrointestinal (GI) side effects, such as nausea, dyspepsia, epigastric pain, indigestion, diarrhoea, vomiting and flatulence. These effects have been reported in 7–17% of patients treated, with nausea and epigastric pain being the most common symptoms Citation2,Citation3. Hence, the side effects associated with the oral Tenoxicam strongly supported the need for a transdermal formulation of Tenoxicam. This route of administration can be devoid of GI side effects as well as associated with other benefits of topical NSAIDs (e.g. site-specific delivery). But studies on topical administration of Tenoxicam have shown that its penetration is very poor by this route compared to other NSAIDs Citation4. Probably due to unfavourable physico-chemical characters of the molecule, such as its melting point range from 205°C to 213°C and log P (octanol/water) of 2.40, Tenoxicam is mostly marketed as oral formulations.
The concept of ultradeformable vesicles is a novel approach and is found to be superior in enhancing penetration of a wide range of substances across skin, whose transport is otherwise restricted due to their unfavourable physico-chemical properties. Ultradeformable vesicles are claimed to be highly flexible, which is due to the incorporation of edge activators (surfactant molecules) into the membrane bilayer Citation5.
This study aims at finding the suitable answer in ultradeformable vesicles for solving the problem of transcutaneous transport of Tenoxicam to reduce the side effects and to provide more targeted delivery for the chronic treatment of rheumatoid arthritis. Finally, the objectives of the study also include converting the colloidal suspension of the ultradeformable vesicular system into a more patient-acceptable and clinically viable formulation by incorporating it into carbopol hydrogel system.
2. Materials and methods
2.1. Materials
Tenoxicam was a kind gift from Ranbaxy India Ltd Phosphotidyl choline (99.5%) and L-carrageenan were purchased from Sigma-Aldrich Co., USA. Carbopol® 934 was obtained from SD Fines Chemical Ltd, Mumbai. Dialysis membrane (cut-off 12,000 Da) and Sodium desoxycholate were purchased form Himedia Laboratories Pvt. Ltd, Mumbai. Tween-80, Triethanolamine, methyl paraben and propyl paraben were purchased from Rankem Ltd, New Delhi. Span-80 and Triton-x-100 were purchased from CDH, Delhi and Pye-Kem Laboratories, Noida. Millipore® water was used for all practical purposes. Animals (Wistar rats) and animal skins were obtained from animal house, Delhi Institute of Pharmaceutical Sciences and Research (DIPSAR), New Delhi. All animal experiments were carried out as per approved protocols by the Institutional Animal Ethical Committee (IAEC).
2.2. Analysis of drug excipients interaction
Drug excipients interaction was studied by using differential scanning colorimetry (DSC) and Fourier transform-infrared (FT-IR) analysis of the final gel containing Tenoxicam. DSC analysis was performed by using DSC-60, Shimadzu. The sample was heated between 40°C and 400°C at the rate of 10°C min−1. Nitrogen gas was introduced at a pressure of 2 bars and a flow rate of 20 mL min−1. Data were analysed by using TA-60 Collector® software.
FT-IR analysis was done on JESCO-420, Japan in the region 400–4000 cm−1 with a resolution of 4 cm−1and the data were analysed by Spectra manager software.
2.3. Preparation of Tenoxicam-loaded ultradeformable vesicles
Vesicles were prepared by the solvent injection method with slight modifications Citation6. Chloroform (10 mL) was taken as solvent in which surfactant and lecithin were dissolved in the ratios 5:95, 15:85 and 25:75 (a mixture of chloroform and methanol in the ratio 7:3 was used in the case of sodium desoxycholate instead of chloroform alone due to solubility problem). Organic phase was added to 20 mL of 7.4 pH buffer containing drug Tenoxicam at a constant concentration of 0.5 mg mL−1 with a rate of 0.2 mL min−1 with constant stirring using stirrer with hot plate. Temperature of the aqueous phase was kept at 55°C. Organic phase (chloroform) was removed during the process. Traces of the organic phase were removed by applying vacuum to get vesicles as colloidal dispersion in aqueous phase.
2.4. Vesicle shape
Ultradeformable vesicles were visualised using a Philips TEM CM 12 electron microscope, with an accelerating voltage of 100 kV. Samples were negatively stained with a 1% aqueous solution of phosphotungstic acid. Vesicle suspension (1 mL) was dried on a microscopic carbon-coated grid for staining. The excess solution was removed by blotting. After drying, the specimen was viewed under the microscope at a 100 k fold enlargement.
2.5. Vesicle size and size distribution
The vesicle size and size distribution were determined by the dynamic light scattering (DLS) method using a computerised inspection system (Malvern Zetasizer, Nano-ZS, Malvern, UK) with DTS (nano) software. For vesicle size measurement, vesicular suspension was mixed with the appropriate medium (7.4 pH phosphate buffer) and the measurements were conducted in triplicate Citation7.
2.6. Entrapment efficiency
The Tenoxicam entrapment capacity of ultradeformable vesicles was determined by centrifugation Citation8. The entrapment efficiency was determined after separating the un-entrapped drug by centrifugation at 4°C at 15,000 rpm (50,000 g) for 2 h, the vesicles were subjected to lyses using Triton-X 100 (0.1% v/v) and analysed for drug content. Entrapment efficiency was expressed as the percentage of total drug entrapped.
2.7. Zeta potential determination
Zeta potential of the vesicles was determined using Zetasizer, Nano-ZS, Malvern, UK. The measurements were made in triplicate.
2.8. Degree of deformability
In this study, the flux of vesicles suspensions through the pores of known size (through a sandwich of different polycarbonate filters with pore diameters between 200 and 50 nm, depending on the starting suspension of ultradeformable vesicles) was driven by an external pressure of 2.5 bars. The amount of vesicle suspension, which was extruded during 5 min, was measured and the vesicle size and the size distribution were monitored by DLS measurement before and after filtration. The experiment was performed in triplicate. The degree of deformability is calculated by using the following formula, Citation9.
2.9. Preparation of nano-vesicular and free drug gels
The best achieved ultradeformable vesicle suspension (formula T3), was incorporated into Carbopol® 934 gel (1%, 1.5% and 2% w/w). Gel containing a specified amount of Carbopol® 934 was dispersed in the dispersion of ultradeformable vesicles with gentle stirring to obtain the total drug equivalent to Tenoxicam 0.1% w/w Citation10. Free drug gel was prepared by a similar method using 1.5% carbopol ().
Table 1. Composition of gels.
2.9. Rheological studies of nano-vesicular gel
2.9.1. Thixotropic study of gels using Herschel–Bulkley model
Herschel–Bulkley model was used to study the thixotropic and flow behaviours of all the three gels (G-1, G-2 and G-3) using Anton Paar MCR 301 rheometer, with cone and plate attachment. The experiment comprised three intervals. At interval-I (0–165 s), shear stress was increased. At interval-II (165–265 s), shear stress was kept constant mimicking the resting phase. At interval-III (265–380 s), shear stress was again decreased mimicking the recovery phase.
2.9.2. Frequency sweep study of gels storage modulus and loss modulus
Gels G-1, G-2 and G-3 were subjected to frequency sweep oscillatory experiments using a similar instrument with a cone and plate attachment at a frequency range of 0.1–100 s−1 in order to study the time-dependent behaviour and structural character of the substance.
2.10. Content uniformity studies of gel
Gel (1 g) was kept in 100 mL of phosphate buffer (pH 7.4) for 48 h with constant stirring with magnetic stirrer. Solution was filtered and observed with UV spectrophotometer at λ max 368 nm. The measurements were made in triplicate.
2.11. In vitro drug release studies
The in vitro evaluation was carried out in the modified Franz diffusion cell. Vesicle suspensions (equivalent to 2 mg of Tenoxicam) were placed over the dialysis membrane. For in vitro release of gels, both dialysis membrane and Wistar rat skin were used. The donor compartment was clamped over it with the help of springs, making sure that there were no bubbles in the receptor chamber. Samples of 1.5 mL were withdrawn at predetermined (5, 10, 15, 30, 60, 120, 240, 360, 720 and 1440 min) time intervals. Fresh receptor fluid was added to the receiver compartment to maintain a constant volume. The samples were then analysed using UV double-beam spectrophotometer at λ max 368 nm. Measurements were repeated in triplicate.
2.12. Pharmacokinetic modelling
The kinetics of Tenoxicam release from the colloidal vesicle suspensions as well as from the gels formulated was determined by finding the best fit of drug release data to distinct models: zero-order Citation11, first-order Citation12 and Higuchi Citation13,Citation14. Furthermore, in order to better characterise the drug-release behaviour Korsmeyer–Peppas semi-empirical model was applied Citation15,Citation16.
2.13. In vivo study
The anti-inflammatory and sustaining action of optimised formulation, marketed gel and drug suspension were evaluated by the carrageenan-induced paw oedema method in Wistar rats developed by Winter et al. Citation17. Wistar rats of either sex weighing between 150 and 200 g were randomly divided into four groups: Group A (control, carbopol gel containing empty vesicles), Group B (oral drug solution), Group C (optimised gel) and Group D (marketed aceclofenac gel), each containing six rats. The animals were starved overnight with water being provided ad libitum. The formulations were administered orally or topically (as per the group) on both paws. After 30 min rats were challenged by a sub-cutaneous injection of 0.1 mL of 1% solution of carrageenan in 0.9% saline on the plantar surfaces of both the paws. The paws were marked with ink at the level of lateral malleolus and immersed in mercury column of a plethysmometer to measure the paw volume. The paw volume was measured before the carrageenan injection and then at 1, 2, 3, 4, 5 and 6 h after injection using plethysmometer. The percentage inhibition of carrageenan-induced paw oedema was calculated for each formulation by using the following equation:
2.14. Stability study
Stability of the optimised gel was determined by physical appearance, content uniformity and release study at 5°C and 25°C at different time intervals (0, 1, 2 and 3 months).
2.15. Statistical analysis
Analysis of variance (Kruskal–Wallis One-way ANOVA) along with multiple comparison test (Student–Newman–Keuls method) and t-test (for two samples) were employed by SigmaStat® 3.5 software at p < 0.05.
3. Results and discussion
3.1. Analysis of drug excipients interaction
The scan of the Tenoxicam–lecithin–carbopol physical mixture was not found to be exactly similar to the pure drug. Amorphous nature of carbopol was quite visible in the initial portion of the scan. However, no significant shift in endothermic peak was found in the physical mixtures of drug and excipients as compared with those of pure drug, which was found to be at 213.86°C. In the physical mixtures of drug and excipients, the peak was found at 214.80°C. Also, the exothermic peak (224.80°C) which was observed in the pure sample of Tenoxicam was quite evident at 229.98°C, confirming the absence of any interaction between Tenoxicam, lecithin and carbopol ().
It was observed that an infrared spectrum () of the freeze-dried gel containing Tenoxicam in T3 vesicles did not show any significant interaction among Tenoxicam, lecithin and Carbopol® 934. A broad peak at 3445.21 cm−1 is due to strong –OH stretching vibrations. Broadness of this peak is due to hydrogen bonding. The strong band observed at 1637.27 cm−1 is attributed to the carbonyl stretching vibration in the secondary amide group (CO–NH). The band located at 1597.73 cm−1 is due to the stretching vibration of pyridyl nitrogen (C=N). Pyridine ring deformation gives a peak at 627.72 cm−1. Peaks at 1326.79 cm−1 and 1041.2 cm−1 are due to asymmetric and symmetric vibrations of sulphonyl group, respectively. All these peaks are in confirmation with the reported data Citation18.
3.2. Characteristics of vesicles
Transmission electron microscopic visualisation of the ultradeformable vesicles has shown that the outer boundaries of the vesicles were quite visible enclosing the inner sac (). The vesicle shape was not found to be completely spherical, suggesting their deformable nature. The size distribution was found to be variable among vesicles of different surfactants and within same surfactant of different concentrations (). In the case of vesicles containing Tween-80, the vesicle size was first found to increase significantly (p < 0.05) with the increase in surfactant concentration. However, on further increase in surfactant concentration, a significant decrease (p < 0.05) in vesicle size was observed. In the case of Span-80, the effects of change in surfactant concentration were found to be completely opposite and statistically significant. Vesicle containing sodium desoxycholate however found to follow the size distribution pattern similar to Tween-80.
Figure 3. Effect of concentrations of different surfactants on the size of ultradeformable vesicles. Notes: S.D.C. is sodium desoxycholate. *Significant (p < 0.05) compared to T3.
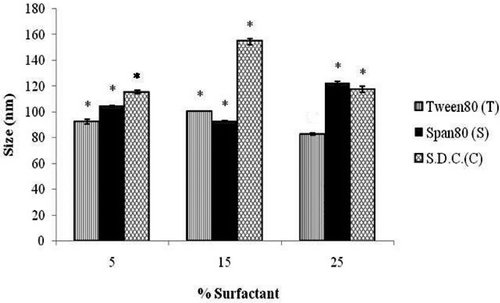
As Tenoxicam is less lipophilic, the entrapment efficiency of the vesicles was found to be low. Except for T1 and C3, all other vesicles were having comparable entrapment.
Zeta potentials of all the vesicles were found to be negative. Also there was no significant difference found between the zeta potential values of different vesicles. One possible reason for such observation might be that lecithin was the common and major excipient of all the vesicles. It behaves like zwitterion, which is negatively charged at basic pH Citation19. As the dispersion medium is phosphate buffer pH 7.4, it imparts negative charge to the vesicles. However, this might not be a reason of concern as the final formulation is a gel, which is more viscous and provides fewer opportunities for the vesicles to aggregate.
Deformability index values of the vesicles of different surfactants () as well as the vesicles of same surfactant with different concentrations were found to be significantly different (p < 0.05). Vesicles with Tween-80 (T1–T3) were found to show an increase in deformability index with the increase in concentration of the surfactant. This was because of the reason that increased concentration of surfactant had made the vesicles more elastic. The effects were totally reverse in the case of sodium desoxycholate (C1–C3) vesicles and the deformability index was found to decrease significantly (p < 0.05) with increase in surfactant concentration. This might be due to the ionic attraction between sodium desoxycholate (positively charged) and lecithin (negatively charged), leading to more rigid vesicles and less flexibility. However, the effects of Span-80 (S1–S3) on deformability index of vesicles were found to be completely different. Deformability index was observed to increase initially with increase in Span-80 concentration, followed by a decrease, which was statistically non-significant. It might be due to the reason that after certain concentration no more Span-80 molecules were able to be incorporated in the vesicle bilayer. T3 vesicles (31.273 ± 0.283 nm) were observed to be the most deformable ones.
Figure 4. Effect of concentrations of different surfactants on the deformability of ultradeformable vesicles. Note: *Significant (p < 0.05) compared to T3.
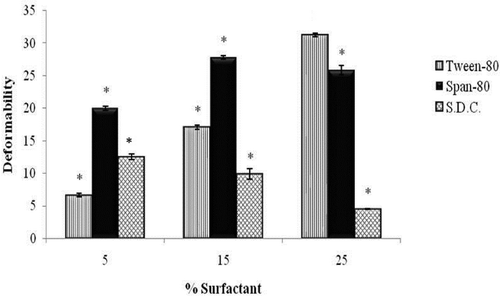
An interesting observation was found among the total in vitro percentage release (24 h) of different formulations. A correlation was clearly visible between total release and deformability index of the formulations. As the pattern followed by deformability index and total release was found to be exactly similar, it can be easily concluded that it is the deformable nature of these vesicles which is the prime feature and hence the true mechanism that increases the drug transport across skin. Maximum total release was observed with T3 vesicles (98.01 ± 0.33%).
All vesicle formulations were subjected to the zero-order, first-order and Higuchi kinetic models. A semi-empirical Korsmeyer–Peppas model was also applied to have a better understanding of the release mechanism (). Vesicle formulations T1, T2, T3, S2 and C3 were found to follow Higuchi kinetics. Release of S1 and C2 vesicles were in accordance with first-order kinetics (concentration dependent). S3 vesicle was found to follow zero-order kinetics (concentration independent). However, release profile of C1 was not completely fitting any of these kinetic models. Furthermore, in order to better characterise the drug release behaviour (i.e. whether the release mechanism is Fickian or non-Fickian), Korsmeyer–Peppas semi-empirical model was applied to the linear portion of the curve to derive more accurate conclusions. According to Korsmeyer–Peppas model, T1, C1, C2 and C3 were found to follow Fickian diffusion release (n ≤ 0.5). However, T2, T3, S1, S2 and S3 were found to release the drug by an anomalous non-Fickian transport (0.5 < n < 1.0). Therefore, it can be considered that transport of drug from ultradeformable vesicles depends upon a number of factors and found to follow a complex summation of number of mechanism, which cannot be accurately predicted by one of these kinetic models alone. Comparative characterisation of the different ultradeformable vesicles containing Tenoxicam is given in .
Table 2. Comparative kinetic analysis of vesicles: release rate constants (k), correlation coefficients (R 2), and release exponent (n) of different ultradeformable vesicles.
Table 3. Comparative features of different ultradeformable vesicles (n = 3).
On comparing release profile of Tenoxicam from optimised T3 vesicles and from the solution of free Tenoxicam, a significant difference was clearly visible (). In spite of being in soluble form Tenoxicam release from the free drug solution was found to be significantly less (p < 0.05) than the release from T3 vesicles. The results clearly indicate the superiority of ultradeformable vesicles in the enhancement of drug penetration.
3.3. Evaluation of different gel formulations containing optimised nano-encapsulated Tenoxicam (T3)
Yield point is the stress at which a material starts flowing or deforming. A very low or very high yield point corresponds to poor spreading and consistency of the formulation. Herschel–Bulkley hysteresis model showed that the yield point of the gel G-1 was very low (9 Pa), along with that hysteresis area was found to be very small (49.23 Pa s−1), suggesting it to be a very low-viscosity gel with low thixotropic recovery. Gel G-2 was found to have a yield point of 110 Pa and a hysteresis area of 638.96 Pa s−1. However, due to the high proportion of gelling agent, gel G-3 was found to be very viscous with a yield point of 200 Pa and a hysteresis area of 1132.81 Pa s−1. The thixotropic behaviour of the gels showed progressive breakdown of gel structure brought about by the applied shear rate that led to the decrease of shear stress and in turn to the decrease of viscosity by time Citation20. However, there was recovery in gel structure with the decrease in shear rate. The size of the loop (hysteresis area) was found to be dependent on the concentration of the gelling agent. It was found to progressively increase from G-1 to G-2. Oscillatory studies had indicated that increase in concentration of gelling agent from G-1 to G-3, consequently leads to increase in both storage modulus (G′) and loss modulus (G″). This clearly suggested a better structural framework with the increase in concentration of Carbopol Citation21. There was a small initial change in G′ and G″ at the low frequency, which however became fairly frequency independent thereafter. Therefore, a very less change in G′ and G″ for all three gels, inferred to a good structural stability, integrity and low ageing effect (). The drug distribution in gel was found to be quite uniform, with average uniformity of content 98.58 ± 0.82%. In vitro comparative release of the gels containing optimised nano-encapsulated Tenoxicam (T3) was studied through dialysis membrane. G-2 and G-1 were found to have shown comparable release of Tenoxicam with transdermal fluxes 30.52 ± 0.75 µg cm−2 h−1 and 28.31 ± 0.38 µg cm−2 h−1, respectively. However, G-1 was ruled out because of lack of consistency. Release from G-3 was significantly less (p < 0.05) with a transdermal flux of 24.99 ± 0.34 µg cm−2 h−1, probably due to the detrimental effect of high viscosity due to high concentration of carbopol. Since G-2 showed maximum release and better rheological properties, it was considered for the final development of the formulation. Therefore, final optimised transdermal gel formulation of Tenoxicam consists of drug entrapped in T3 ultradeformable vesicle which was ultimately converted to a gel as per the formula followed for G-2.
On kinetic modelling and comparison of the release profile, it was found that there was not much difference in the release pattern of drug as well mechanism of drug release from all three gels (). All of them were found to release the drug in accordance to first-order kinetics. Also a Fickian diffusion release (n ≤ 0.5) of drug was clearly exhibited by all the three gel formulations.
Table 4. Comparative kinetic analysis of gels: release rate constants (k), correlation coefficients (R 2), and release exponent (n) of gels of different concentrations.
A comparative in vitro release study of the optimised gel of Tenoxicam (G-2) and the gel containing free drug (G-4) through dialysis membrane had shown significant differences (). The total release after 24 h from G-2 was almost two times the release shown by G-4 with the transdermal fluxes 30.52 ± 0.75 µg cm−2 h−1 and 16.94 ± 0.85 µg cm−2 h−1, respectively. Also the initial fast release of the drug from G-2 gives it an edge over the G-4 in acute conditions. In vitro release of the drug through rat skin was found to get slightly reduced. But, still the drug release from G-2 was almost twice the release from G-4 with transdermal fluxes 26.94 ± 1.37 µg cm−2 h−1and 14.74 ± 0.62 µg cm−2 h−1, respectively ().
3.4. In vivo study
The in vivo performance of selected optimised ultradeformable vesicle containing formulation (G-2) was compared with oral Tenoxicam as well as marketed aceclofenac gel (Hifenac®) by the carrageenan-induced paw oedema model (). The ultradeformable vesicle gel was found to show highest inhibition of oedema (22.62 ± 1.19%) as compared to the marketed aceclofenac gel (14.88 ± 1.38%) and oral Tenoxicam (14.63 ± 2.07%). It was observed that not only the extent but also the beginning of the inhibitory effect of optimised Tenoxicam gel was early.
3.5. Stability studies
Stability studies have shown that content uniformity was fairly good during 3-month testing at both the temperatures. However, total percentage in vitro release for 24 h has dropped significantly (from 57.60 ± 1.30% in the zero month to 52.43 ± 0.25% in the third month), which shows that the formulation is less stable at 25°C than 5°C (). This clearly indicates the instability of the formulation at higher temperatures. Instability of vesicles containing lecithin is suspected to be the cause of this result. Therefore it is strongly recommended that we store this formulation at lower temperature conditions.
Table 5. Effect of different storage conditions on total percentage release (n = 3).
4. Conclusion
The novel ultradeformable vesicle carrier system was found to be capable of enhancing transdermal permeation of Tenoxicam and producing strong anti-inflammatory effect. Transdermal application of ultradeformable vesicle-based Tenoxicam offers all the advantages of topical NSAIDs, and overcomes the limitations of oral delivery. In addition, carrier-mediated transdermal Tenoxicam delivery is unlikely to involve shunts, lesions or damage to the skin cells. Rather than this, the drug is inferred to be transported into the body between the intact skin cells. Thus, this study leads to the generic conclusion that ultradeformable vesicles provide a suitable solution to the low transdermal transport of Tenoxicam.
Acknowledgments
Declaration of interest: The authors declare that they have no conflict of interest. The following study is the sole intellectual effort and work of authors and in no form an imitation of any previous work.
This article was originally published with errors. This version has been corrected. P lease see Erratum (http://dx.doi.org/10.1080/17458080.2013.829704).
References
- Bird , HA . 1987 . Clinical experience with tenoxicam: A review . Scand. J. Rheumatol. , 16 : 102 – 106 . doi: 10.3109/03009748709102186
- Gonzalez , JP and Todd , PA . 1987 . Tenoxicam: A preliminary review of its pharmacodynamic and pharmacokinetic properties, and therapeutic efficacy . Drugs , 34 : 289 – 310 . doi: 10.2165/00003495-198734030-00001
- Vischer , TL . 1987 . Efficacy and tolerability of tenoxicam – An overview . Scand. J. Rheumatol. , 16 : 107 – 112 . doi: 10.3109/03009748709102187
- Corderoa , J , Camachob , M , Obacha , R , Domenecha , J and Vilab , L . 2001 . In-vitro based index of topical anti-inflammatory activity to compare a series of NSAIDs . Eur. J. Pharm. Biopharm. , 51 : 135 – 142 . doi: 10.1016/S0939-6411(00)00149-1
- Cevc , G and Blume , A . 1992 . Lipid vesicles penetrate into intact skin owing to the transdermal osmotic gradients and hydration force . Biochim. Biophys. Acta. , 1104 : 226 – 232 . doi: 10.1016/0005-2736(92)90154-E
- Lasic , DD , Weiner , N , Riaz , M and Martin , F . 1996 . “ Liposomes ” . In Pharmaceutical Dosage Form: Disperse System , Edited by: Libberman , HA , Martin , MR and Banker , GS . 43 – 85 . New York : Marcel Dekker .
- El Maghraby , GMM , Williams , AC and Barry , BW . 1999 . Skin delivery from ultradeformable liposomes: Refinement of surfactant concentration . J. Pharm. Pharmacol. , 51 : 1123 – 1134 . doi: 10.1211/0022357991776813
- Bendas , ER and Tadros , MI . 2007 . Enhanced transdermal delivery of salbutamol sulfate via ethosomes . AAPS PharmSciTech. , 8 : E1 – E8 . doi: 10.1208/pt0804107
- Berge Vanden , BAI , Wertz , PW , Junginger , HE and Bouwstra , JA . 2001 . Elasticity of vesicles assessed by electron spin resonance. Electron microscopy and extrusion measurement . Int. J. Pharm. , 217 : 13 – 24 . doi: 10.1016/S0378-5173(01)00576-2
- Manosroi , A , Jantrawuta , P and Manosroi , J . 2008 . Anti-inflammatory activity of gel containing novel elastic niosomes entrapped with diclofenac diethylammonium . Int. J. Pharm. , 360 : 156 – 163 . doi: 10.1016/j.ijpharm.2008.04.033
- Varelas , CG , Dixon , DG and Steiner , C . 1995 . Zero-order release from biphasic polymer hydrogels . J. Control. Rel. , 34 : 185 – 192 . doi: 10.1016/0168-3659(94)00085-9
- Gibaldi , M and Feldman , S . 1967 . Establishment of sink conditions in dissolution rate determinations – theoretical considerations and application to nondisintegrating dosage forms . J. Pharm. Sci. , 56 : 1238 – 1242 . doi: 10.1002/jps.2600561005
- Higuchi , T . 1961 . Rate of release of medicaments from ointment bases containing drugs in suspension . J. Pharm. Sci. , 50 : 874 – 875 . doi: 10.1002/jps.2600501018
- Higuchi , T . 1963 . Mechanism of sustained-action medication: Theoretical analysis of rate of release of solid drugs dispersed in solid matrices . J. Pharm. Sci. , 52 : 1145 – 1149 . doi: 10.1002/jps.2600521210
- Korsmeyer , RW , Gurny , R , Doelker , EM , Buri , P and Peppas , NA . 1983 . Mechanism of solute release from porous hydrophilic polymers . Int. J. Pharm. , 15 : 25 – 35 . doi: 10.1016/0378-5173(83)90064-9
- Peppas , NA . 1985 . Analysis of Fickian and non-Fickian drug release from polymers . Pharm. Acta Helv. , 60 : 110 – 111 .
- Winter , CA , Risley , EA and Nuss , GW . Anti-inflammatory and antipyretic activities of indomethacin, 1-(p-chlorobenzoyl)-5-methoxy-2-methylindole-3-acetic acid, J. Pharmacol. Exp. Ther. 141 (1963), pp. 369–376
- Zayed , MA , Nour El-Dien , FA , Mohamed , GG and El-Gamel Nadia , EA . 2007 . Anti-inflammatory activity of gel containing novel elastic niosomes entrapped with diclofenac diethylammonium . Int. J. Pharm. , 360 : 156 – 163 .
- Shah , DO and Schulman , JH . 1967 . The ionic structure of lecithin monolayers . J. Lipid Res. , 8 : 227 – 233 .
- El-Gamal , IM and Gad , EAM . 1997 . Low temperature rheological behavior of umbarka waxy crude and influence of flow improver . Revue De L’institut Français Du Pétrole , 52 : 369 – 379 .
- Martinez , MR , Gallardo , JL , Benavides , MM , López-Duran , JDG and Lara , VG . 2007 . Rheological behavior of gels and meloxicam release . Int. J. Pharm. , 333 : 17 – 23 . doi: 10.1016/j.ijpharm.2006.09.045