Abstract
This study deals with the synthesis and characterisation of ZnO nanoparticles and suspension of ZnO-ethylene glycol (EG) nanofluid. Crystalline ZnO nanoparticles are synthesised using the chemical method. The nanofluids were synthesised by the dispersion of ZnO nanoparticles in EG solution using an ultrasonicator. The prepared ZnO nanoparticles are characterised by X-ray diffraction, scanning electron microscopy, transmission electron microscopy and UV-Vis absorption spectrum. The particle size distribution and ultrasonic parameters of the synthesised nanofluid are measured with the help of acoustic particle sizer (APS-100) and ultrasonic interferometer. The observed features of ZnO nanoparticles and ZnO-EG nanofluids are discussed in correlation with known properties of other nanofluids.
1. Introduction
The material ZnO is a direct wide band gap (3.3 eV) semiconductor. In recent years, ZnO has received mended attention because of its potential advantage over the group III-nitrides due to its commercial availability as a bulk single crystal having larger excitation binding energy and excellent radiation hardness Citation1. Metal oxide nanostructures have attracted considerable attention for their potential applications in many technologies, such as solar cells, electroluminescent devices, electrochromic windows and chemical sensors Citation2. There are many reports on synthesis, growth kinetics, crystallisation of ZnO, and its applications. Among various nanoforms, one-dimensional oriented nanostructures, such as nanorods, nanowires, nanotubes and nanopins, are very important because of enormous commercial applications Citation3–11. To achieve high performance of these devices, metal oxides are used in these applications. The nanostructured ZnO is found to be one of the metal oxides, possesses high-surface area as well as good electrical, electrochemical and structural properties. The ZnO powders at nanoparticles size possess medical applications such as antibacterial agent Citation12.
Recently, the sound propagation through random media has also been a subject of great interest. Such media include colloidal suspensions, porous material, magnetorheological medium and nanofluids. The nanofluid is a colloidal suspension of material nanoparticle in the carrier fluids. The nanofluids have great interest due to a broad application in different fields Citation13,Citation14. A study on the preparation and characterisation of ZnO nanoparticles, ZnO-Ethylene Glycol and ZnO-water nanofluids have been reported in the literature Citation14–16. The anomalous behaviour of ultrasonic velocity in sintered ZnO provides information about the pore size, pore shape and their distribution Citation17. Very few nanofluids and magnetic fluids were characterised with ultrasonic techniques Citation18–21 but no work has been reported for the determination of ultrasonic properties in ZnO nanofluid in the knowledge of authors. In the present investigation, we have chosen the nanofluid of ZnO in carrier fluid ethylene glycol for the characterisation. Initially, ZnO nanoparticles are synthesised through chemical route and characterised by X-ray diffraction (XRD), scanning electron microscopy (SEM), transmission electron microscopy (TEM) and UV-Vis spectroscopy. Later on, nanofluid of ZnO is synthesised using ethylene glycol solution with the help of ultrasonicator. The ultrasonic velocity and attenuation measurements are performed for ultrasonic characterisation of the prepared fluid. The obtained results are discussed for the characterisation.
2. Sample preparation and measurements
Zinc oxide nanoparticles were successfully prepared by the one-step salvo-thermal reaction of zinc acetate in alcohols. Zinc acetate dihydrate (13.4 mmol) was first dissolved in methanol and another solution of potassium hydroxide that was prepared by dissolving potassium hydroxide (28.96 mmol) in methanol. The potassium hydroxide solution was added drop-wise to the zinc acetate solution at 52°C under vigorous stirring. Drop-wise addition process took nearly 1.5 h. After this, nanoparticles started to precipitate and the solution became turbid. The heater and stirrer were removed after 2 h and the solution was allowed to sit for another 2 h. The ZnO nanoparticles settled at the bottom and the excess mother liquor was removed and the precipitate was washed twice with methanol (50 mL). The precipitates were collected by the centrifugation process and allowed to dry at room temperature for 1 day. The obtained solid was crushed to get white powder. The precipitate was then dispersed in EG with ultrasonicator. The dispersed solution was translucent and stable up to 2 weeks. The chemical reaction involved in the formation of ZnO can be written as
Several important synthesis parameters such as precursor concentration, rate of evaporation and reaction time were investigated in order to tailor the growth of ZnO nanostructures into a variety of dimensions and structures. The size and shape of the particles were controlled by the reaction condition (evaporation rate). We made two samples of ZnO nanoparticles controlling the evaporated rate.
The powdered samples of ZnO nanoparticles were analysed with XRD pattern using an X′ Pert-Pro, PANalytical (with λ = 1.5405 Å Cu-Kα radiation) operating at room temperature. TEM of the model E.M.-C.M.-12 (Philips) and SEM of Oxford model Leo 1550 are used to study the morphology. The absorption spectrum was recorded using Lambda 35, Perkin-Elmer double beam UV-Vis absorption spectrometer for the optical characterisation.
2.0 wt% and 5.0 wt% of ZnO nanoparticles synthesised at low evaporation rate were dispersed in the solution of ethylene glycol with ultrasonicator (500 W) to form the nanofluid of the ZnO. Ultrasonic velocity measurements in prepared nanofluids were made at 2 MHz of frequency with the help of a variable path interferometer technique in the temperature range from 25°C to 65°C. Water was circulated around the sample using a specific thermostat. The measured value of ultrasonic velocity was accurate to ±0.1% with an error of measurement of ±0.5°C in the temperature. The particle size distribution and ultrasonic attenuation measurements were made by an acoustic particle sizer model APS-100 (Matec Applied Sciences, Massachusetts, USA).
3. Results and discussion
3.1. XRD, SEM, TEM and UV-Vis absorption spectrum
The structural analysis of ZnO nanoparticle samples, synthesised at low and high evaporation rates is carried out by XRD, SEM, TEM and UV-Vis absorption spectrum measurements. The XRD patterns of both the samples are shown in , while SEM and TEM images are shown in .
Figure 1. (Colour online) XRD pattern of the powder sample of ZnO nanoparticles at different evaporation rates.
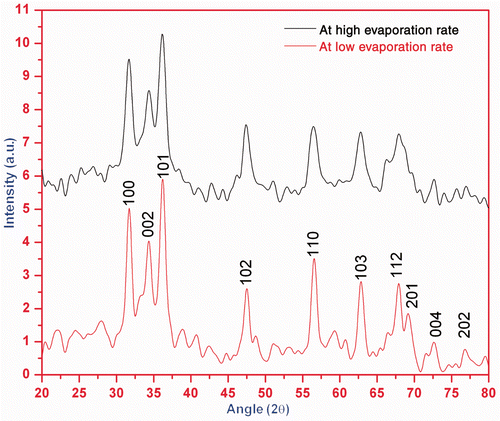
Figure 2. (a) SEM image of agglomerated ZnO nanoparticles and (b) TEM image of the ZnO nanoparticles. Inset shows the selected area electron diffraction pattern revealing the crystalline structure of the ZnO nanoparticles.
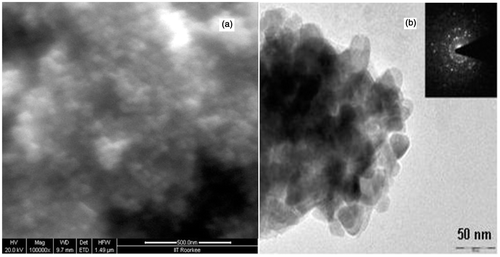
XRD pattern () shows that narrower and high-intense peaks are obtained in the diffraction pattern for sample formed at low evaporation rate than the sample formed at high evaporation rate. Zinc acetate solutions were neutralised with varying amounts of KOH solutions and then hydrothermally aged at low and high evaporation rates. ZnO nanoparticles precipitated through dissolution/reprecipitation mechanism from starting zinc acetate solutions. The evaporation rate controls the pH of solution which governs significant change in the shape and size of ZnO nanoparticles. Thus, the degree of crystallinity and particle size of samples increase with decrease in evaporation rate. The crystallite/particle size has been calculated using the Debye Scherrer equation for all diffraction peaks. The particle size of the sample is found ranging between 35 and 50 nm. The intensity peaks of XRD pattern are coordinated with JCPDS file number 790206 and it is confirmed that the crystalline sample is of ZnO which is hexagonal in structure with lattice parameters a = 3.249 Å and c = 5.206 Å. shows typical SEM image of ZnO nanoparticles. The SEM image shows that agglomerated ZnO nanoparticles have non-spherical shape and diameter. The TEM, , also confirms the approximately same size of the particles in the crystalline samples as predicted by XRD.
The room temperature UV-Vis absorption spectrum of the ZnO nanoparticles is recorded in the wavelength range of 200–800 nm. represents the UV-Vis absorption spectrum of the ZnO nanoparticles showing a broad absorption peak at 367 nm (3.38 eV). The absorption peak for 40 nm ZnO nanoparticles has been reported at 361 nm (3.44 eV) elsewhere Citation22–24. The absorption peak for 5–6 nm ZnO nanoparticles has been found between 360 and 365 nm Citation2. A slight variation in absorption peak in the present case and others is due to difference in particle size of ZnO nanoparticle.
Optical absorption of the ZnO nanoparticles is analysed by the following relationship:
The plot of versus
is shown in , which has a divergence at an energy value equal to band gap (Eg
). Hence, this plot also provides the information about the band gap of ZnO nanoparticles.
3.2. Particle size distribution and ultrasonic parameters
The measured particle size distribution in the prepared ZnO-EG nanofluid with the help of acoustic particle sizer (APS-100) is shown in , which clearly explains that the size of the suspended particle in the EG (carrier fluid) is found to be in the range 35–50 nm, while the maximum number of particles is of size ∼ 42 nm.
The measured ultrasonic velocities in pure EG and two nanofluid samples of ZnO in temperature range 25°C–65°C are shown in . This plot indicates that the ultrasonic velocity in the samples increases with the temperature and it becomes approximately constant after 55°C. Ultrasonic velocity has larger value for nanofluid in comparison to that of pure EG solution and also the magnitude of velocity increases with the particle volume fraction.
The expression of longitudinal velocity (V) of ultrasonic wave in a medium is given by following expression.
The present nanofluids are prepared with ZnO nanoparticles primed at low evaporation rate. Thus, the size of ZnO nanoparticles will be the same in all nanofluids. The velocities are measured at low frequency (2 MHz). Thus, it may be concluded that temperature-dependent velocity at low-frequency regime in ZnO nanofluid depends only on the particle volume fraction. Consequently, we can say that our study of velocity provides a justification that ultrasonic velocity in nanofluid is independent of particle size in low-frequency region and is function of particle volume fraction/concentration Citation28,Citation29. The ultrasonic velocity in nanofluid is quadratic function of temperature at low frequency Citation25.
The measured frequency-dependent ultrasonic absorption/attenuation in the present ZnO nanofluid and pure EG at room temperature is shown in . This plot indicates that the attenuation in nanofluids is approximately the same as for EG up to 30 MHz, while above this frequency, the attenuation is found to increase with particle volume fraction and frequency.
The explanation of the present attenuation curve can be understood with the understanding of thermal wave length (, where KS
, ρS
and CS
are the thermal conductivity, density and specific heat of the dispersed particle; ω is the frequency of the wave) and viscous wave length (
, η is the viscosity of the matrix). When the viscous wave length is comparable to particle radius (r), the viscous loss is a major reason behind ultrasonic absorption while the viscous drag, scattering and thermal losses is effective at
Citation26,Citation29. The scattering loss is a function of particle volume fraction and particle size Citation21, while the thermal loss is caused by the temperature variation produced by propagation of sound waves in different components of suspension. Hence, it may be stated that the ultrasonic attenuation in nanofluid sample before 30 MHz is influenced by viscous loss due to being similar viscous wavelength and particle size, while above 30 MHz, viscous drag loss and scattering loss are prominent due to similar thermal wavelength and particle size. The study states that the total attenuation in the ZnO nanofluid is equal to the sum of viscous loss, viscous drag loss, scattering loss and thermal loss. These losses depend on frequency, particle size and particle volume fraction.
The ultrasonic attenuation found in both the nanofluids is greater than the carrier fluid and increases with particle volume fraction above the 30 MHz. The different particle volume fraction for both the nanofluids is the reason behind their different ultrasonic attenuations. The larger interaction of ultrasound with dispersed particles at higher frequency causes the larger scattering which results a larger increase in the ultrasonic attenuation at high-frequency region. A polynomial fit analysis to the ultrasonic attenuation provides that attenuation in nanofluid can be written as . For n = 0, the coefficient of frequency is equivalent to attenuation in polymer matrix. While for n ≠ 0, the coefficient of frequency is a function of particle size and particle volume fraction.
The plot of α/f 2 with frequency for pure EG and 5.0 wt% ZnO-EG nanofluid is shown in . It is clear from that α/f 2 decreases with frequency. As the frequency increases, the effective viscosity of a fluid decreases monotonically towards zero Citation30. This proves that the viscous and viscous drag loss play an important role to the ultrasonic attenuation in the ZnO-EG nanofluid. The frequency-dependent α/f 2 indicate that the process of transition from pure EG solution to stable ZnO-EG nanofluid is relaxational in nature.
4. Conclusion
The present structural and morphological studies of nanoparticles show that the degree of crystallinity of the ZnO nanoparticles depends on the evaporation rate through pH during its synthesis. The factors that control the size, shape and distribution of the nanoparticles are pH of solution controlled by evaporation rate, concentration of solution and ultrasonication time. ZnO nanoparticles have an optical direct band gap of 3.38 eV which is greater than the band gap of pure ZnO material. The temperature variation of ultrasonic velocity in ZnO nanofluid depends mainly on the particle volume fraction of dispersed particles in low-frequency region and is larger than the velocity in pure matrix (EG). The non-linear relation between velocity and temperature is found for the present nanofluid samples. In the low-frequency region, the viscous loss is effective to the total attenuation while scattering and viscous drag losses are prominent in the high-frequency region. Frequency, particle size and concentration are the governing parameters to ultrasonic attenuation. The formation of stable ZnO nanofluid is relaxational in nature.
Thus, the ultrasonic properties (velocity and attenuation) are the efficient tools for the characterisation of the nanofluid, as it provides useful information and characteristics. The present results along with known physical properties of nanocrystalline ZnO offer a new dimension for the further study and characterisation of nanofluid.
Acknowledgements
The authors are thankful to the University Grant Commission-India [UGC project no. 32-18/2006(SR)] for the financial support for this work. We are extremely grateful to Emeritus Prof. O.N. Srivastava, FNA, Department of Physics, Banaras Hindu University for his kind permission for the XRD and TEM measurements in his laboratory; Prof. Vijaya Agarwal, Department of Physics, IIT, Roorkee for facilitating in SEM measurement; and Prof. Ram Gopal, Department of Physics, University of Allahabad for UV-Visible facility. We are also deeply indebted to Prof. S.K. Kor, University of Allahabad; Prof. B.P. Singh, Senior Director, ASET, New Delhi; Dr A.K. Tiwari, BSNVPGC, Lucknow; Dr Priyanka Awasthi, DMO, Lucknow; Dr Akhilesh Mishra, DST, New Delhi; Dr A.K. Gupta, NIOS, Noida; Dr A.K. Yadav, Ambedkar University, Lucknow; Dr Giridhar Mishra, Mr S.K. Verma, Mr Meherwaan Rathore, Mr Sanjay Upadhyaya and Mr Kajal De, University of Allahabad; and Mrs Neera Bhutani, ASET, New Delhi for many useful helps like reading the manuscript, discussion and knowledgeable suggestions during the preparation and revision of the manuscript.
This article was originally published with errors. This version has been corrected. P lease see Erratum (http://dx.doi.org/10.1080/17458080.2013.829704).
References
- Jagadish , C and Pearton , SJ . (eds), Zinc Oxide Bulk, Thin Films and Nanostructures: Processing, Properties and Applications, Elsevier, Oxford, 2006
- Seow , ZLS , Wong , ASW , Thavasi , V , Jose , R , Ramakrishna , S and Ho , GW . 2009 . Controlled synthesis and application of ZnO nanoparticles, nanorods and nanospheres in dye-sensitized solar cells . Nanotechnology , 20 045604, 6pp doi: 10.1088/0957-4484/20/4/045604
- Ho , GW and Wong , ASW . 2007 . One step solution synthesis towards ultra-thin and uniform single-crystalline ZnO nanowires . Appl. Phys. A , 86 : 457 – 462 . doi: 10.1007/s00339-006-3798-3
- Raykar , VS and Singh , AK . 2010 . Thermal and rheological behavior of acetylacetone stabilized ZnO nanofluids . Thermochim. Acta , 502 : 60 – 65 . doi: 10.1016/j.tca.2010.02.007
- Hossain , MK , Ghose , SC , Boontongkong , Y , Thanachayanon , C and Datta , J . 2005 . Growth of zinc oxide nanowires and nanobelts for gas sensing applications . J. Metastable Nanocryst. Mater. , 23 : 27 – 30 . doi: 10.4028/www.scientific.net/JMNM.23.27
- Ma , X-Y and Zhang , W-D . 2009 . Effects of flower-like ZnO nanowhiskers on the mechanical, thermal and antibacterial properties of waterborne polyurethane . Polym. Degrad. Stab. , 94 : 1103 – 1109 . doi: 10.1016/j.polymdegradstab.2009.03.024
- Du , T , Song , H and Ilegbusi , OJ . 2007 . Sol–gel derived ZnO/PVP nanocomposite thin film for superoxide radical sensor . Mater. Sci. Eng. C , 27 : 414 – 420 . doi: 10.1016/j.msec.2006.05.040
- Schmid , G . 1992 . Large clusters and colloids. Metals in the embryonic state . Chem. Rev. , 92 : 1709 – 1729 . doi: 10.1021/cr00016a002
- Pan , ZW , Dai , ZR and Wang , ZL . 2001 . Nanobelts of semiconducting oxides . Science , 291 : 1947 – 1949 . doi: 10.1126/science.1058120
- Guo , L , Ji , YL , Xu , H , Simon , P and Wu , Z . 2002 . Regularly shaped, single-crystalline ZnO nanorods with wurtzite structure . J. Am. Chem. Soc. , 124 : 14864 – 14865 . doi: 10.1021/ja027947g
- Ko , GH , Heo , K , Lee , K , Kim , DS , Sohn , Y and Choi , M . 2007 . An experimental study on the pressure drop of nanofluids containing carbon nanotubes in a horizontal tube . Int. J. Heat Mass Transfer , 50 : 4749 – 4753 . doi: 10.1016/j.ijheatmasstransfer.2007.03.029
- Zhang , L , Ding , Y , Povey , M and York , D . 2008 . ZnO nanofluids – A potential antibacterial agent . Prog. Nat. Sci. , 8 : 939 – 944 . doi: 10.1016/j.pnsc.2008.01.026
- Choi , US . 1995 . Enhancing thermal conductivity of fluids with nanoparticles . ASME FED , 231 : 99 – 105 .
- Sastry , NNV , Bhunia , A , Sundararajan , T and Das , SK . 2008 . Predicting the effective thermal conductivity of carbon nanotube-based nanofluids . Nanotechnology , 19 055704, 8pp doi: 10.1088/0957-4484/19/24/245604
- Chung , SJ , Leonard , JP , Nettleship , I , Lee , JK , Soong , Y , Martello , DV and Chyu , MK . 2009 . Characterization of ZnO nanoparticle suspension in water: Effectiveness of ultrasonic dispersion . Powder Technol. , 194 : 75 – 80 . doi: 10.1016/j.powtec.2009.03.025
- Yu , W , Xie , H , Chen , L and Li , Y . 2009 . Investigation of thermal conductivity and viscosity of ethylene glycol based ZnO nanofluids . Thermochim. Acta , 491 : 92 – 96 . doi: 10.1016/j.tca.2009.03.007
- Martin , LP and Rosen , M . 1997 . Analysis of ultrasonic velocity measurements on sintering zinc oxide . Ultrasonics , 35 : 65 – 71 . doi: 10.1016/S0041-624X(96)00093-5
- Raj , B , Philip , J , Rajkumar , KV and Kalyanasundara , P . 2006 . Effect of magnetic field on ultrasonic velocity in a magnetic nanofluids . INSA J Part B – Phys. Sci. , 72 : 145 – 151 .
- Taketomi , S . 1986 . The anisotropy of the sound attenuation in magnetic fluid under an external magnetic field . J. Phys. Soc. Japan , 55 : 838 – 844 . doi: 10.1143/JPSJ.55.838
- Skumiel , A . 2004 . The effect of temperature on the anisotropy of ultrasound attenuation in a ferrofluid . J. Phys. D: Appl. Phys. , 37 : 3073 – 3079 . doi: 10.1088/0022-3727/37/22/003
- Biwa , S , Watanabe , Y , Motogi , S and Ohno , N . 2004 . Analysis of ultrasonic attenuation in particle-reinforced plastics by a differential scheme . Ultrasonics , 43 : 5 – 12 . doi: 10.1016/j.ultras.2004.03.002
- Wu , L and Wu , Y . 2007 . Synthesis and optical characteristic of ZnO nanorod . J. Mater. Sci. , 42 : 406 – 408 . doi: 10.1007/s10853-006-0727-y
- Singh , DP , Singh , J , Mishra , PR , Tiwari , RS and Srivastava , ON . 2008 . Synthesis, characterization and application of semiconducting oxide (Cu2O and ZnO) nanostructures . Bull. Mater. Sci. , 31 : 319 – 325 . doi: 10.1007/s12034-008-0051-z
- Yadav , A , Prasad , V , Kathe , AA , Raj , S , Yadav , D , Sundaramoorthy , C and Vigneshwaran , N . 2006 . Functional finishing in cotton fabrics using zinc oxide nanoparticles . Bull. Mater. Sci. , 29 : 641 – 645 . doi: 10.1007/s12034-006-0017-y
- Singh , DK , Pandey , DK and Yadav , RR . 2009 . An ultrasonic characterization of ferrofluid . Ultrasonics , 49 : 634 – 637 . doi: 10.1016/j.ultras.2009.03.005
- Urick , RJ . 1948 . The absorption of sound in suspensions of irregular particles . J. Acoust. Soc. Am. , 102 : 283 – 289 . doi: 10.1121/1.1906373
- Temkin , S . 1998 . Sound propagation in dilute suspensions of rigid particles . J. Acoust. Soc. Am. , 103 : 838 – 849 . doi: 10.1121/1.421244
- Gomez Alvarez-Arenas , TE , Elvira Segura , L and Riera Franco de Sarabia , E . 2002 . Characterization of suspensions of particles in water by an ultrasonic resonant cell . Ultrasonics , 39 : 715 – 727 . doi: 10.1016/S0041-624X(02)00375-X
- Józefczak , A and Skumiel , A . 2006 . Field induced aggregates in a bilayer ferrofluid characterized by ultrasound spectroscopy . J. Phys.: Condens. Matter , 18 : 1869 – 1876 . doi: 10.1088/0953-8984/18/6/004
- Edmonds , PD . (ed.), Methods of Experimental Physics, Vol. 19, Academic Press, New York, 1981