Abstract
Nanoparticles that synchronously exhibit fluorescence and magnetism have great potential in biomedical applications. In this study, porous magnetic nanospheres ranging mainly from 300 to 800 nm in diameter were prepared by spray-drying an ethanol solution containing an ethyl cellulose (EC) polymer and Fe3O4 nanoparticles, carried out at lower temperatures (e.g. 40°C). The pores in the nanospheres were mainly caused by the addition of the Fe3O4 nanoparticles in the EC precursor. It was found that the more Fe3O4 nanoparticles added into the EC precursor, the greater the number and size of the pores in the nanospheres. Positively charged quantum dots (QDs) could be easily adsorbed onto the surface of these porous magnetic nanospheres through a simple electrostatic interaction. It was also found that the greater the porosity of the nanospheres, the more QDs could be adsorbed. The QD-coated magnetic nanospheres obtained are expected to have good multifunctionality due to the synchronous exhibition of bright fluorescence and superparamagnetism. This is a novel, simple and highly efficient method for preparing magneto-fluorescent nanospheres, which are expected to be of considerable interest to material scientists and biologists.
1. Introduction
A nanocomposite containing both superparamagnetic Fe3O4 nanoparticles and quantum dots (QDs) has great potential in areas of biomedical research since this nanocomposite synchronously exhibits magnetic and fluorescent properties. This magneto-fluorescent nanocomposite can be mainly used for (1) bio-labelling, (2) fluorescence imaging and magnetic resonance imaging, (3) bio-separation and (4) cancer therapy (including photodynamic therapy of QDs and thermotherapy of Fe3O4 nanoparticles). As such, in the recent years, this multifunctional nanocomposite has attracted much attention, and many literature works on the preparation of this nanocomposite have been reported Citation1–10. The methods employed for the formation of Fe3O4–QDs nanocomposites are summarised here. One method involves incorporating Fe3O4 and QDs into polymer nanospheres through covalent conjugation Citation1 or through a self-assembly approach Citation2,Citation3. Another method involves incorporating Fe3O4 and QDs into silica nanospheres through the hydrolyzation reaction of tetraethyl orthosilicate Citation4–10. A third method involves directly growing QDs onto the surfaces of the Fe3O4 nanoparticles Citation11. Among these methods, self-assembly involving a physical electrostatic adsorption process is a simple and highly efficient one.
For adsorbing more QDs on the surface of magnetic nanospheres, the nanospheres should have a large surface area. If the nanospheres are porous, they will have a large surface-to-volume ratio. Therefore, the greater the number of pores in the nanosphere surface, the larger the surface-to-volume ratio. Therefore, in this study, we used a spray-dryer equipped with an ultrasonic atomiser for incorporating Fe3O4 nanoparticles into an ethyl cellulose (EC) matrix to yield water-insoluble porous magnetic nanospheres. Since the porous nanospheres are negatively charged, a large amount of positively charged QDs can be easily adsorbed onto the surface of these porous nanospheres. This is a novel, simple and highly efficient method for preparing magneto-fluorescent nanospheres.
2. Experimental
2.1. Materials
EC, oleic acid, 1-octadecene and FeCl3 · 6H2O were purchased from Sigma–Aldrich Corporation, Te powder from Fluka Chemical Corp., Cysteamine from TCI Co. Ltd., n-Hexane, CdCl2 · 2.5H2O, NaBH4, sodium oleate, NaCl, NaOH, ethanol and HPLC water from Shanghai Chemical Regent Co., and N2 were purchased from Shanghai Pujiang Specialty Gases Co., Ltd.
2.2. Methods
1. | Synthesis of oleic acid – capped Fe3O4 nanoparticles. Monodispersed Fe3O4 nanoparticles were synthesised by a thermal decomposition method described in Park et al. Citation12. Briefly, the metal–oleate complex was prepared first by reacting metal chlorides and sodium oleate at 70°C; 36 g of the resulting metal–oleate was then dissolved in 200 g of 1-octadecene and reacted at 320°C for 30 min, and the oleate-capped Fe3O4 nanoparticles obtained were then naturally cooled to room temperature. The precipitates were washed with ethanol five times to remove the excess oleate. | ||||
2. | Synthesis of cysteamine-capped CdTe QDs. Water-soluble CdTe QDs were synthesised as follows: fresh NaHTe solution was prepared first by dissolving Te powder into NaBH4 solution (1 : 2 molar ratio) and reacting for more than 24 h under a nitrogen atmosphere at room temperature. The NaHTe solution was then added into a solution of CdCl2-containing cysteamine and the pH value of the mixture obtained was adjusted to 6.5 by addition of NaOH. The concentration of CdCl2 was 2 mM and the molar ratio of Cd to Te to cysteamine 5 : 1 : 8. The mixture was then heated to 98°C under a nitrogen atmosphere flow. About 40 h later, the reaction solution was cooled naturally to room temperature. | ||||
3. | Preparation of porous magnetic nanospheres. A spray-dryer equipped with an ultrasonic atomiser (Jiangyin Spray Dryer Factory, China) was used for preparing the nanospheres. The spray-drying conditions are as follows. EC powder (0.5 g) was dissolved in ethanol (100 mL) and the Fe3O4 nanoparticles were then dispersed in the EC solution and sonicated for about 1 h. For investigating the effect of the concentration of the Fe3O4nanoparticles and spray dry temperature on the morphology of the pores in the nanospheres, the concentrations of the Fe3O4 nanoparticles in the EC solution were set at 0, 0.325, 0.65 and 1.95 mg/mL, respectively, and the inlet temperatures at 40°C, 60°C and 80°C, respectively. The feed flow rate for all spray-drying conditions was about 65 mL/h. | ||||
4. | Adsorption of QDs onto the magnetic nanospheres. In this study, 2.5 mg of magnetic nanosphere powders was dispersed in aqueous solution and sonicated for 10 min; 1 mL of QD aqueous solution (∼1.5 µmol/L) was then mixed with the nanosphere suspension. After being continuously and vigorously stirred for 30 min, the mixed suspension was precipitated by centrifugation. The precipitate obtained was washed three times with water to remove the free QDs. | ||||
5. | Characterisations. The morphologies of the magnetic nanospheres before and after the adsorption of QDs were observed by scanning electron microscopy (SEM). The room-temperature magnetic properties were measured on a Vibrating Sample Magnetometer (Lakeshore 735, USA), with a maximum applied field of 1.5 T. The photoluminescent (PL) spectra of the QDs and the QD-coated magnetic nanospheres were measured on a fluorescence spectrometer (F-2500; Hitachi, Japan) with a xenon lamp source. To detect the fluorescence stability, the EC–Fe3O4–QD nanosphere powders were dispersed in water and continuously excited at 365 nm for 60 min. During the irradiation process, the maximum fluorescent intensities of the sample were measured. The brightfield and true-colour fluorescent images of the QD-coated magnetic nanospheres were recorded on an upright fluorescent microscope (Leica DME, Germany). To detect how many QDs were adsorbed onto the magnetic nanospheres, the QD-coated magnetic nanospheres were incubated with aqua regia for 1 week until the QDs were completely dissolved into Cd and Te. The aqua regia was diluted with water and the Cd atoms in the diluted solutions were then measured using inductive coupled plasma atomic emission spectrometry (ICP-AES, IRIS-Advantage 1000; Thermo Jarrell Ash Co., USA). |
3. Results and discussion
3.1. Effect of Fe3O4 concentration on the morphology of magnetic nanospheres
SEM images of the nanospheres prepared at a 60°C inlet temperature are shown in . When the precursors for spray-drying have no Fe3O4 nanoparticles, the EC nanospheres obtained exhibited smooth surfaces and no pores could be observed on the surfaces (). This indicates that the EC is a good plasticity polymer. In general, the surface of the droplet flying in the spray-dryer is dried first and a solidified shell is formed on the droplet surface. The shells with good plasticity will not be broken by the relatively high vapour pressure within the particles, and the channels (or pores) in the particles formed by the vapour will also close quickly. Additionally, the anamorphic droplets flying in the spray-dryer can return to their normal spherical shape before the drying finishes. Spherical nanoparticles of EC without surface defects were therefore obtained. The integrity of the EC nanospheres was not destroyed when the precursor for spray-drying contained even a small amount of Fe3O4 nanoparticles (0.325 mg/mL) (). However, when the concentration of Fe3O4 nanoparticles in the precursor increased from 0.325 to 0.65 mg/mL, many larger EC–Fe3O4 nanospheres were obtained with pores. When the concentration of Fe3O4 nanoparticles in the precursor was 1.95 mg/mL, most of the nanospheres had a large number of pores, which could be clearly observed (). The pore size and number of pores increased with an increasing concentration of Fe3O4 nanoparticles, which indicates that these pores in the EC–Fe3O4 nanospheres may be caused by the addition of larger amounts of Fe3O4 nanoparticles. It is known that the plasticity of a polymer will decrease after being mixed with inorganic particles, and the more inorganic particles added, the poorer the plasticity of the polymer. Therefore, when the droplets containing EC and a large amount of Fe3O4 nanoparticles were sprayed using the spray-dryer, the solidified shells with poor plasticity coated around the droplets will inhibit further evaporation of the remaining solution, and these shells will be broken by the high vapour pressure within the particles, resulting in the formation of pores (or holes). The detailed mechanism for the formation of porous nanoparticles is still under consideration. It was found that the size distribution of the nanospheres slightly shifted to a larger size after the Fe3O4 nanoparticles were introduced into the EC precursor. The average diameters of the EC nanospheres prepared from the precursor containing 0, 0.325, 0.65 and 1.95 mg/mL of Fe3O4 nanoparticles were 498.75 ± 252.64, 534.90 ± 296.05, 536.07 ± 324.20 and 560.99 ± 289.067 nm (), respectively.
3.2. Effect of inlet temperature on the morphology of magnetic nanospheres
Dry temperature is an important factor affecting the morphology of particles prepared by the spray-drying method. The higher the inlet temperature, the more anamorphic particles are formed Citation13. In this study, however, the morphologies of the EC nanospheres without Fe3O4 nanoparticles were not affected by the inlet temperature. As shown in , the EC nanospheres formed, respectively, at 40°C and 80°C inlet temperatures have similar morphology. None of the nanospheres were observed to have obvious pores on their surface, further indicating the good plasticity of the EC polymer. Although further enhancing the inlet temperature (such as 100°C) may result in the formation of pores on the nanosphere surface, high inlet temperatures may present risks because the precursor solution used was ethanol and the carrier gas air. As shown in and (B′), after the Fe3O4 nanoparticles were added into the precursor (Fe3O4 concentration: 0.65 mg/mL), the EC–Fe3O4 nanospheres prepared at 80°C have slightly more pores than those prepared at 40°C. When the Fe3O4 concentration in the precursor was increased to 1.95 mg/mL, however, the EC–Fe3O4 nanospheres prepared at different inlet temperatures had many pores, and the pore number obtained at lower () and higher () inlet temperatures showed no obvious difference. Combined with the results shown in , it can be concluded that the pores in the nanospheres were mainly controlled by the addition of Fe3O4 nanoparticles. In this regard, porous magnetic nanospheres can be formed at lower inlet temperatures (e.g. 40°C). Because the formation of dry particles in the spray-dryer is a rapid process (several seconds or fewer), this method for preparing porous magnetic nanospheres at low temperatures is highly efficient and safe.
Figure 3. Effect of inlet temperature on the morphologies of nanospheres. (A, A′) EC nanospheres and (B, B′) EC–Fe3O4 nanospheres. The concentration of Fe3O4 nanoparticles in the EC precursor was 0.65 mg/mL. (C, C′) EC–Fe3O4 nanospheres, where the concentration of Fe3O4 nanoparticles in the EC precursor was 1.95 mg/mL. Inlet temperature of A, B and C was 40°C and that of A′, B′ and C′ 80°C.
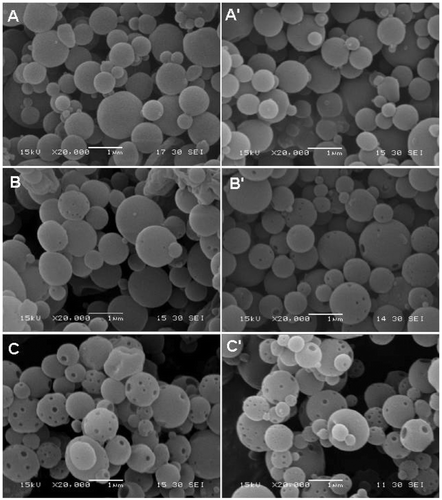
3.3. Amount of QDs loaded in the nanospheres and the morphology of the QD-coated magnetic nanospheres
Porous nanospheres usually have great potential in applications in biomedical research areas since the pores can be used as drug, gene vector or other material carriers. The greater the number of pores in the nanospheres, the higher the nanosphere surface-to-volume ratio and therefore, the greater the amount of foreign materials that can be loaded into the nanospheres. In this study, CdTe QDs were loaded into the EC–Fe3O4 nanospheres. In order to investigate the role, the pores play with respect to the QD loading, the same amounts of EC nanospheres, respectively, containing 0, 0.65 and 1.95 mg/mL of Fe3O4 nanoparticles were incubated with QD solutions of the same amount. The results show that the maximal concentrations of Cd adsorbed onto the above three kinds of EC nanospheres were 19.65, 24.82 and 29.38 µg/mg, respectively, which were measured by ICP-AES. This indicates that the greater the number of Fe3O4 nanoparticles in the EC nanospheres, the greater the adsorption of QDs on the surface of the nanospheres. After QDs were adsorbed onto the surfaces of the porous EC–Fe3O4–QD nanospheres, the nanospheres obtained were more rough (). Because the cysteamine-capped QDs were positively charged with diameters of several nanometers in size and the EC–Fe3O4 nanospheres negatively charged with larger diameters, the QDs could be easily adsorbed onto the EC–Fe3O4 nanospheres without the addition of any conjugation agents (such as polymers), and all the EC–Fe3O4–QD nanospheres obtained were still separate from each other.
3.4. Fluorescence and magnetism of the EC–Fe3O4–QD nanospheres
After QDs were adsorbed onto the surfaces of the porous EC–Fe3O4 nanospheres, the fluorescent emission peak slightly red-shifted from 643 to 646 nm (), indicating that the EC–Fe3O4–QD nanospheres obtained had a fluorescence similar to that of the QDs. After being excited for 60 min, the maximum fluorescent intensity of EC–Fe3O4–QD decreased only about 15% compared with that of initial EC–Fe3O4–QD nanospheres (). Each EC–Fe3O4–QD nanosphere exhibited a bright red fluorescence (), whereas EC–Fe3O4 alone exhibits a blue autofluorescence (data not shown), which further indicates that a large amount of QDs have been successfully adsorbed onto the nanospheres. The EC–Fe3O4–QD nanospheres also exhibited superparamagnetism since their remanence and coercivity were close to zero (). The saturation magnetisation was close to 25.01 memu/g, indicating stronger magnetism. These nanospheres synchronously exhibit a bright fluorescence and strong magnetism that is expected to be of considerable interest to biologists because such nanospheres can be used for biolabelling, bioseparation and local therapy via hyperthermia.
Figure 5. (Colour online) (A) PL spectra of QDs (a) before and (b) after being adsorbed onto the EC–Fe3O4 nanospheres. (B) Stability of EC–Fe3O4–QD nanospheres with continuous excitation of 365 nm over time. (C) Brightfield and true-colour fluorescent images of the EC–Fe3O4–QD nanospheres. The sample was the same as that shown in .
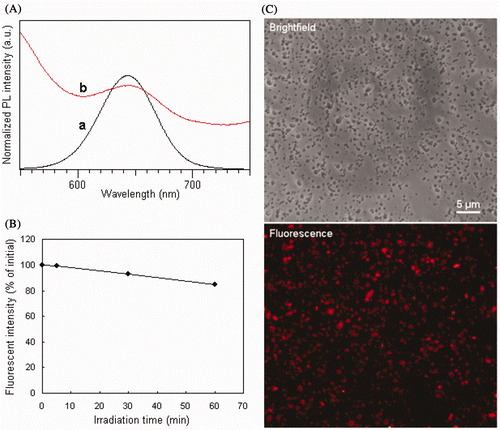
4. Conclusions
Porous magnetic nanospheres consisting of an EC framework and Fe3O4 cores were successfully prepared by a highly efficient spray-drying method, using a spray-dryer equipped with an ultrasonic atomiser. Positively charged QDs were subsequently adsorbed onto the surfaces of these nanospheres, and magneto-fluorescent nanospheres (EC–Fe3O4–QDs) thus obtained. The formation of pores in the nanospheres was mainly controlled by the introduction of Fe3O4 nanoparticles, and the contribution of the dry temperature was nearly negligible. The pores in the EC–Fe3O4 nanospheres were very useful, where the greater the number of pores, the greater the adsorption of QDs onto the surface of the nanospheres. The EC–Fe3O4–QD nanospheres not only exhibit a bright fluorescence, but also superparamagnetism, suggesting that these nanospheres may have great potential in areas of biomedical research. The porous magnetic nanospheres are expected to be used not only as fluorescent QD carriers, but also as drug, gene and other inorganic material carriers in the future.
Acknowledgements
This study was supported in part by the Program for New Century Excellent Talents in University (No. NCET-07-0618), the Key Technologies R&D Program in the 11th Five-year Plan of China (No. 2009ZX09103-701), National High Technology R&D Program of China (No. 2007AA03Z319) and the Nanoscience Foundation of Shanghai (No. 0952nm04700).
This article was originally published with errors. This version has been corrected. P lease see Erratum (http://dx.doi.org/10.1080/17458080.2013.829704).
References
- Shi , D , Cho , HS , Chen , Y , Xu , H , Gu , H , Lian , J , Wang , W , Liu , G , Huth , C , Wang , L , Ewing , RC , Budko , S , Paulett , GM and Dong , Z . 2009 . Fluorescent polystyrene-Fe3O4 composite nanospheres for in vivo imaging and hyperthermia . Adv. Mater. , 21 ( 21 ) : 2170 – 2173 . doi: 10.1002/adma.200803159
- Chu , M , Song , X , Cheng , D , Liu , S and Zhu , J . 2006 . Preparation of the quantum dots -coated magnetic polystyrene nanospheres for cancer cells labeling and separation . Nanotechnology , 17 : 3267 – 3273 .
- Kim , BS and Taton , TA . 2007 . Multicomponent nanoparticles via self-assembly with cross-linked block copolymer surfactants . Langmuir , 23 ( 4 ) : 2198 – 2202 . doi: 10.1021/la062692w
- Guo , J , Yang , W , Wang , C , He , J and Chen , J . 2006 . Poly(N-isopropylacrylamide)-coated luminescent/magnetic silica microspheres: Preparation, characterization, and biomedical applications . Chem. Mater. , 18 ( 23 ) : 5554 – 5562 . doi: 10.1021/cm060976w
- Sun , P , Zhang , H , Liu , C , Fang , J , Wang , M , Chen , J , Zhang , J , Mao , C and Xu , S . 2010 . Preparation and characterization of Fe3O4/CdTe magnetic/fluorescent nanocomposites and their applications in immuno-labeling and fluorescent imaging of cancer cells . Langmuir , 26 ( 2 ) : 1278 – 1284 . doi: 10.1021/la9024553
- Xu , Y , Karmakar , A , Wang , D , Mahmood , MW , Watanabe , F , Zhang , Y , Fejleh , A , Fejleh , P , Li , Z , Kannarpady , G , Ali , S , Biris , AR and Biris , AS . 2010 . Multifunctional Fe3O4 cored magnetic-quantum dot fluorescent nanocomposites for RF nanohyperthermia of cancer cells . J. Phys. Chem. C , 114 ( 11 ) : 5020 – 5026 . doi: 10.1021/jp9103036
- Salgueiriño , MV , Correa , DM , Spasova , M , Liz , ML and Farle , M . 2006 . Composite silica spheres with magnetic and luminescent functionalities . Adv. Funct. Mater. , 16 ( 4 ) : 509 – 514 . doi: 10.1002/adfm.200500565
- Gaponik , N , Radtchenko , IL , Sukhorukov , GB and Rogach , AL . 2004 . Luminescent polymer microcapsules addressable by a magnetic field . Langmuir , 20 ( 4 ) : 1449 – 1452 . doi: 10.1021/la035914o
- Earhart , C , Jana , NR , Erathodiyil , N and Ying , JY . 2008 . Synthesis of carbohydrate-conjugated nanoparticles and quantum dots . Langmuir , 24 ( 12 ) : 6215 – 6219 . doi: 10.1021/la800066g
- Gao , J , Zhang , W , Huang , P , Zhang , B , Zhang , X and Xu , B . 2008 . Intracellular spatial control of fluorescent magnetic nanoparticles . J. Am. Chem. Soc. , 130 ( 12 ) : 3710 – 3711 . doi: 10.1021/ja7103125
- McDaniel , H and Shim , M . 2009 . Size and growth rate dependent structural diversification of Fe3O4/CdS anisotropic nanocrystal heterostructures . ACS Nano , 3 ( 2 ) : 434 – 440 . doi: 10.1021/nn800737a
- Park , J , An , K , Hwang , Y , Park , JG , Noh , HJ , Kim , JY , Park , JH , Hwang , NM and Hyeon , T . 2004 . Ultra-large-scale syntheses of monodisperse nanocrystals . Nat. Mater. , 3 : 891 – 895 . doi: 10.1038/nmat1251
- Chu , M , Zhou , L , Song , X , Pan , M , Zhang , L , Sun , Y , Zhu , J and Ding , Z . 2006 . Incorporating quantum dots into polymer microspheres via a spray-drying and thermal-denaturizing approach . Nanotechnology , 17 : 1791 – 1796 . doi: 10.1088/0957-4484/17/6/041