Abstract
The anodic oxidation of sulphadiazine (SD) was investigated at a glassy carbon electrode modified by multi-walled carbon nanotube (MWCNT–GCE), using cyclic voltammetry and chronoamperometry. The results indicate that MWCNT-modified GCEs show an efficient and selective electrocatalytic activity towards the anodic oxidation of SD among biologically important compounds in buffered solutions at pH = 7. It was found that oxidation of SD at the surface of MWCNT–GCE occurs at a potential less positive than that of unmodified GCE (about 100 mV). The diffusion coefficient of SD was also estimated using chronoamperometry. The kinetic parameters such as the electron transfer coefficient between SD and modified electrode, α, and the charge transfer rate constant, ks , for oxidation of SD at the MWCNT–GCE surface were determined according to the Laviron procedure. The dissociation constants of oxidised and reduced acid–base species of SD can be obtained from the E 1/2 versus pH curves. The linear dependence of the peak current on the concentration was observed in the range 10–2000 µmol L−1 with a detection limit of 7.1 µmol L−1. The method was also applied to determinate the SD in human blood plasma and urine samples.
1. Introduction
Sulphonamides (4-amino-N-2-pyrimidinylbenzenesulphonamide) are synthetic antibacterial drugs with low potency used against a wide range of parasitic organisms including Plasmodia, Toxoplasma gondii and Pneumocystis carinii. Their mechanism of action is based on the inhibition of dihydropteroate synthetase. Resistance arises through multiple mutations in this gene. Sulphonamides are used for therapeutic, prophylactic and growth-promoting purposes in animals and in the treatment of trachoma and ocular toxoplasmosis in ophthalmology.
Sulphonamide compounds can be electrochemically oxidised at the –NH2 group and reduced at the –SO2NH– group Citation1,Citation2. Thus, several electrochemical methods have been developed for the determination of residual SD according to both oxidation and reduction reactions Citation3,Citation4. Owing to concerns regarding the redox reaction, some studies have reported the determination of sulphonamides in pharmaceutical products Citation5–8.
Direct electron transfer of biological molecules in the film-modified electrode can not only provide information of their structure and mechanism of redox transformations, but also establish a foundation for constructing biosensors, bioreactors and clinic biomedical devices Citation9–11. Recently, carbon nanotubes can provide a suitable electrode substrate for the preparation of modified electrodes. Carbon nanotubes are an interesting class of non-metals which offer high electrical conductivity, high surface area, significant mechanical strength and good chemical stability. They have been known to promote electron transfer reactions when used as an electrode-modifying material. Utilisation of these properties has led to the application of carbon nanotubes as biosensors Citation12, electron field emission sources Citation13, actuators Citation14, nanoelectronic devices Citation15 as well as electrocatalytic behaviour towards many biomolecules such as enzymes Citation16, drugs Citation17–19, glucose Citation20 and lysozyme Citation21. Due to the high reactivity of multi-walled carbon nanotube (MWCNT), it seems that the use of MWCNT as modifiers can be of importance in the catalysis of SD's slow oxidation reaction. Following our recent studies Citation22–24, we herein describe the preparation of glassy carbon electrodes (GCEs) modified by MWCNT and its electrocatalytic activity towards SD oxidation.
2. Experiments
2.1. Apparatus
Electrochemical measurements were carried out with a Metrohm model 746 VA trace analyzer connected to a 747 VA stand. The working electrode was a GCE (2 mm diameter). Before use, the working electrode was sequentially polished with graded 10 µM alumina powder, and rinsed with doubly distilled water. A platinum wire and a commercial Ag/AgCl saturated KCl electrode from Metrohm were used as auxiliary and reference electrodes, respectively. The scan rate in cyclic voltammetry was 100 mVs−1, with the exception of the experiments in which the influence of this variable was studied. Chronoamperometric measurements were conducted using different concentrations of SD in solution at the MWCNT–GCE. The step potential (Es ) was 0.98 V with duration time 50 s.
2.2. Reagents
SD was obtained from Alfa Aesar. All other reagents used were of analytical grade without further purification. A SD solution (1 mmolL−1) was used in a Britton–Robinson (B–R) buffer (pH 7.0) solution. A stock B–R buffer solution 0.04 molL−1 with respect to boric acid, orthophosphoric acid and acetic acid was prepared from proanalysis reagents. From this stock, buffer solutions with various values of pH were prepared by the addition of 0.2 molL−1 sodium hydroxide solution. MWCNT with purity 95% (10–30 nm diameters and 5 µm length) were obtained from io-li-tec, Ionic Liquid Technologies. Solutions were deaerated by bubbling high-purity argon gas for 10 min through them prior to the experiments. All electrochemical experiments were carried out at room temperature.
2.3. Preparation of the MWCNT film-modified GCE
The GCE was sanded using ultrafine sand paper, polished with 10 µm alumina slurry in sequence and successively sonicated in water for 10 min.
MWCNT (4 mg) was added to 1 mL of DMF. A homogeneous and stable suspension of 4 mgmL−1 was achieved with the aid of ultrasonic agitation for about 30 min. The GCE was coated by casting with a 5 µL suspension of MWCNT–DMF and dried in the air.
2.4. Procedure for analysis of real samples
In this section, 1 mL urine or serum sample was collected and diluted to 10 mL buffer solution. The resulted solution was subsequently transferred to the electrochemical cell, and the voltammograms were recorded. To confirm the validity of the results, the sample was spiked with a certain amount of SD and the recovery rates of the spiked samples were determined.
3. Results and discussion
3.1. Electrochemical behaviour of SD at the MWCNT–GCE
The cyclic voltammograms of SD at a bare GCE and MWCNT–GCE in 0.04 molL−1 buffer solution (pH = 7) were shown in . In the absence of SD, no redox peaks were observed at either the bare or modified GCEs during the cyclic voltammetry measurements within the potential window (). Compared to the bare GCE (), a large background current was observed at the MWCNT–GCE () during the cyclic voltammetric scan, which can probably be attributed to a high double-layer capacity Citation25. The oxidation peak at the bare GCE was weak and broad due to slow electron transfer, while the response was considerably improved at the MWCNT–GCE. The SD showed only one irreversible oxidation peak at approximately 1.02 V at the bare GCE (). In comparison, at the MWCNT–GCE, the anodic peak was observed at the less positive potential of 0.90 V and clearly showed an increased peak current (about 6-fold increase) compared to the bare GCE (). The increased current as well as the negative shift of the anodic peak demonstrated an efficient catalytic oxidation of SD on the MWCNT–GCE. Nevertheless, it was found that the oxidation peak current of sulphonamides has a remarkable decrease during successive cyclic voltammetric sweeps at bare GCE. This phenomenon may be caused by the fact that the adsorption of sulphonamides or their oxidative products occurs at the electrode surface.
3.2. The effect of the scan rate
Cyclic voltammograms of SD at the MWCNT–GCE in 0.04 M buffer solutions (pH = 7) at various scan rates are shown in . As observed in , the anodic peak current of SD is proportional to the scan rate (lower than 200 mVs−1), which indicates that the electrode process is surface-controlled. However, at higher scan rates (more than 200 mVs−1), the plot of I versus was linear, most likely due to the diffusion-controlled SD oxidation (). From the observations, it can be concluded that the electrochemical process consists of a mixture of diffusion- and adsorption-controlled processes, depending on the scan rate Citation26–29. Furthermore, from the slope of the linear plot of I versus υ, the surface concentration of the electroactive species (
) can be estimated to be approximately 2.37 × 10−6 molcm−2 according to the following equation Citation30:
Figure 2. (A) Cyclic voltammetric responses of 1.0 mmolL−1 SD at MWCNT–GCE (B–R buffer solution, pH 7.0) at scan rates, (inner to outer) 10–400 mVs−1. (B and C) The plots of peak currents vs. υ and υ 1/2, respectively. (D and E) The variation of peak potential vs. υ and log υ, respectively.
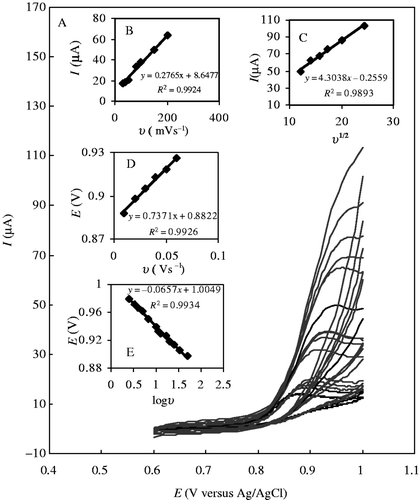
Laviron-derived general expressions for the linear potential sweep voltammetric response for the case of surface-confined electroactive species. This theory enables the determination of the apparent charge transfer rate constant (ks ) for electron transfer between the electrode and surface-deposited layer as well as the transfer coefficient (α) by measuring the variations of the peak potentials with scan rates.
Our results showed that by increasing the scan rate, the peak potential is shifted to more positive potentials. Because of the irreversible electrode process of SD's oxidation reaction, the Laviron's equation Citation31 was used to estimate αn and ks values, as follows:
The E 0 value can be deduced from the intercept of Ep versus υ plot on the ordinate by extrapolating the line to υ = 0 (). Knowing E 0 and from the graphical representations of Ep versus log υ for SD in the presence of MWCNT (), the values of αn = 0.90 and ks = 2616 s−1 were obtained from the slope and intercept, respectively.
3.3. The effect of pH
Like other sulphonamide compounds, the oxidation-wave potential for the –NH2 group of SD depends on the pH of the buffer solution. The plot of the oxidation-wave potential versus the pH value for SD at MWCNT–GCE is demonstrated in . By increasing the pH, the oxidation peak potential of SD shifts to less positive potentials due to the more easy oxidation of SD at low concentrations of protons. Here, pH 7 was selected as optimum pH due to two reasons: first, it is a physiological pH in biology and medicine; second, the peak shape is more well-defined with relatively maximum peak current.
In SD molecule, two acid–base centres, amine and amide, are always present. shows three defined breaks corresponding to the apparently pK a values, 1.9, 4.2 and 7.2. The values of pK a are in excellent agreement with the literature Citation32. The formal value E 0' is linear with pH in acidic media, with a slope of 66 mVpH−1. This value is close to the theoretical value of 59 mVpH−1 Citation33, indicating the participation of the same proton and electron numbers in the electrochemical process.
3.4. Chronoamperometric studies
Chronoamperometry can be used for the determination of SD's diffusion coefficient (D). We obtained chronoamprograms at a fixed potential of 0.98 V over 50 s in B–R buffer (pH = 7) solution containing different concentrations of SD ().
Figure 4. (A) Chronoamperograms of SD in B–R buffer solution, pH 7.0, containing: (a) 0.1, (b) 0.4, (c) 0.7 and (d) 1.0 mmolL−1 SD. (B) Plot of I versus t −1/2 for different concentrations. (C) The plot of the slope of straight lines of plots in (B) against the SD concentration.
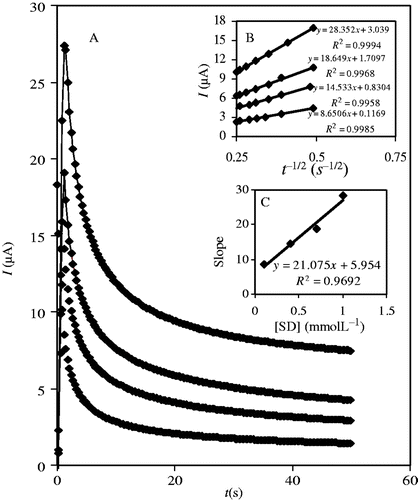
From the slope of a plot of I versus t −1/2 at different concentrations (), D values were estimated according to the Cottrell equation Citation33. The slopes of the resulting straight lines were then plotted versus the SD concentration (), from whose slope an average value of D = 4.16 × 10−5 cm s−1 was obtained.
3.5. The effect of the amount of MWCNT composite
In preliminary experiments, it became obvious that both the amount and injected volume of MWCNT influence the voltammetric analysis. Hence, these parameters were studied and optimised. The effect of the amount of MWCNT on the anodic peak was examined by varying the amount from 2 to 8 mg in 1 mL of DMF. The results showed that the peak current reached a maximum at 4 mg and decreased thereafter. The effect of the injected volume of MWCNT composite film was also investigated and a maximum current was obtained at 5 µL. However, the background current was also increased by increasing the injected volume. Thus, the optimum of the amount of MWCNT was selected to be about 5 µL.
3.6. Analytical application, analytical figures of merit
The cyclic voltammograms of SD at various SD concentrations are shown in . The relationship between the variation of the optimum current and SD concentration was found to be linear over the two ranges of SD concentration. Under the optimised conditions mentioned above, a linear range was yielded for SD, 10–2000 µmol L−1 (, inset). A detection limit of 7.1 µmol L−1was calculated according to the YLOD = XB + 3SB, where YLOD is the signal at the limit of detection and XB and 3SB are the mean and the standard deviation (n = 7) of the blank signal, respectively Citation34.
Figure 5. Cyclic voltammograms of SD in B–R buffer solution, pH 7.0, at scan rate 100 mVs−1 with various concentrations of SD (10–2000 molL−1). Inset: the plot of the current vs. SD concentration.
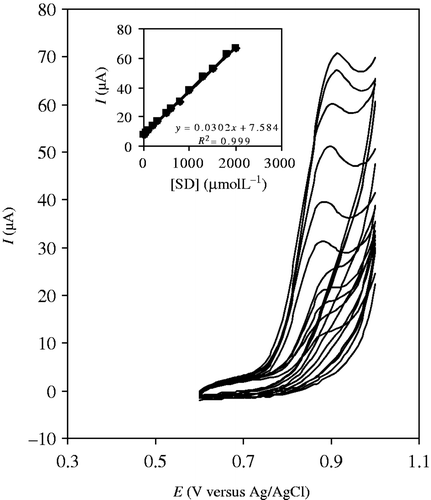
Reproducibility of the method was evaluated by successive determinations (n = 7) of SD. A relative standard deviation (RSD) value of less than 1.43 was obtained for 1.0 mmolL−1 SD, which demonstrated a good reproducibility in film deposition on the electrode surface.
3.7. Interferences
Under optimum experimental conditions, the effects of some foreign species on the determination of SD at 1.0 mmolL−1 were evaluated in detail. It was observed that 100-fold concentrations of , Li+, Al3+,
, Cd2+, Cu2+,
, Sn2+, Na+, K+, Ni2+, Fe2+, Zn2+, Ba2+, Sr2+, Pb2+, Cr2+, Cl−,
and
and 50-fold concentrations of L-lysine, L-arginine, L-serine, L-histidine, L-glutamic acid and L-threonine had almost no influence on the current response of SD (signal change below 5%). All the obtained results clearly represent the efficiency of the method for the determination of SD in the presence of the potentially interfering species.
3.8. Recovery studies and SD determination in serum and urine
To evaluate the applicability of the present method on real matrices, assays were performed in serum and urine. Recovery experiments were carried out by adding standard solutions of SD to matrices of serum and urine. The results showed that satisfactory recovery for SD could be acquired. summarises the data obtained for SD assays performed on the matrices studied using the optimised experimental methodology.
Table 1. Results of the analysis of real samples.
3.9. Comparison of the applied methods with other reported methods
A comparison of the electroanalytic behaviour of MWCNT–GCE for SD oxidation with some of the previously reported methods is shown in . As can be concluded, the method is quite comparable to the existing methods, however, with clear advantages including wider linear dynamic range, simplicity, low cost and the use of non-toxic reagents, which are not potentially hazardous in terms of human health.
Table 2. Comparison of the electroanalytic behaviour of MWCNT–GCE for SD oxidation with some of the previously reported methods.
4. Conclusions
In this article, an easily prepared MWCNT–GCE was used to investigate the detailed electrochemical behaviour of SD. The reported modified electrode significantly improved SD's electrochemical response and clearly demonstrated the excellent electrocatalytic activity of the MWCNT–GCE towards the redox reaction of SD. Some electrochemical parameters such as the apparent charge transfer rate constant, transfer coefficient, diffusion coefficient and surface concentration of the electroactive species were calculated. Under optimised experimental conditions, good analytical performance was obtained, including suitable precision together with excellent linear dynamic range and detection limit. The method is sensitive enough for the analysis of lower concentrations of SD in serum and urine. Furthermore, the proposed method does not require expensive instruments or critical analytical reagents.
Acknowledgements
The authors gratefully acknowledge the financial support from the Department of Chemistry of Alzahra University.
References
- Voorhies , J D and Adams , R N . 1958 . Voltammetry solid electrodes anodic polarography of sulfadrugs . Anal. Chem. , 30 : 346 – 350 . doi: 10.1021/ac60135a010
- Campestrini , I , De Braga , O C , Vieira , I C and Spinelli , A . 2010 . Application of bismuth-film electrode for cathodic electroanalytical determination of sulfadiazine . Electrochim. Acta. , 55 : 4970 – 4975 . doi: 10.1016/j.electacta.2010.03.105
- Ali , A MM . 1993 . Electroanalytical studies of azo sulpha drugs . application to novel heterocyclo-sulphonamideazo dye, Anal. Lett. , 26 : 1635 – 1647 .
- Diaz , T G , Cabannillas , A G , Valenzuela , M I and Sallinas , F . 1996 . Polarographic behaviour of sulfadiazine . sulfamerazine, sulfamethazine and their mixtures. Use of partial least squares in the resolution of the non-additive signals of these compounds, Analysis , 121 : 547 – 552 .
- Joseph , R and Kumar , K G . 2010 . Differential pulse voltammetric determination and catalyticoxidation of sulfamethoxazole using [5,10,15,20-tetrakis (3-methoxy-4- hydroxy phenyl) porphyrinato] Cu(II) modified carbon paste sensor . Drug Test. Anal. , 2 : 278 – 283 . doi: 10.1002/dta.129
- Carrazon , J MP , Corona , P C and Diez , L MP . 1987 . Electroanalytical study of sulphadiazine at solid electrodes, determination in pharmaceutical preparations . Electrochim. Acta. , 32 : 1573 – 1575 . doi: 10.1016/0013-4686(87)90006-5
- Rao , T N , Sarada , B V , Tryk , D A and Fujishima , A . 2000 . Electroanalytical study of sulfa drugs at diamond electrodes and their determination by HPLC with amperometric detection . J. Electroanal. Chem. , 491 : 175 – 181 . doi: 10.1016/S0022-0728(00)00208-4
- Preechaworapun , A , Nuwatanakul , S C , Einaga , Y , Grudpan , K , Motomizu , S and Chailapakul , O . 2006 . Electroanalysis of sulfonamides by flow injection system/high-performance liquid chromatography coupled with amperometric detection using boron-doped diamond electrodes . Talanta , 68 : 1726 – 1731 . doi: 10.1016/j.talanta.2005.08.040
- Borzooeian , Z , Safavi , A , Sheikhi , M H , Aminlari , M and Doroodmand , M M . 2010 . Preparation and investigation on properties of lysozyme chemically bonded to single-walled carbon nanotubes . J. Exp. Nanosci. , 5 : 536 – 574 . doi: 10.1080/17458081003699122
- Saxena , U , Das , M , Ahmad , S , Barbora , L , Borthakur , M , Verma , A , Bora , U and Goswami , P . 2011 . Multiwalled carbon nanotube-based bi-enzyme electrode for total cholesterol estimation in human serum . J. Exp. Nanosci. , 6 : 84 – 95 . doi: 10.1080/17458080.2010.487230
- Iram , M , Guo , C , Liu , H and Ishfaq , A . Highly ordered mesostructured silica spheres as template for polyaniline nanofibres: Synthesis, characterisation and their electrochemical properties, J. Exp. Nanosci. Available online: 27 Apr 2011
- Lyons , M EG and Keedey , G P . 2008 . Carbon nanotube based modified electrode biosensors: Electrochemical studies of the flavin group redox kinetics at SWCNT/glucose oxidase composite modified electrodes . Int. J. Electrochem. Soc. , 3 : 819 – 853 .
- De Heer , W A , Chatelain , A and Ugarte , D . 1995 . A carbon nanotube field-emission electron source . Science , 270 : 1179 – 1180 . doi: 10.1126/science.270.5239.1179
- Baughman , R H , Cui , C C , Zakhidov , A A , Iqbal , Z , Barisci , J N , Spinks , G M , Wallace , G G , Mazzoldi , A , De Rossi , D , Rinzler , A G , Jaschinski , O , Roth , S and Kertesz , M . 1999 . Carbon nanotube actuators . Science , 284 : 1340 – 1344 . doi: 10.1126/science.284.5418.1340
- Tans , S , Verschueren , A and Dekker , C . 1998 . Room-temperature transistor based on a single carbon nanotube . Nature , 393 : 49 – 52 . doi: 10.1038/29954
- Lyons , M EG . 2009 . Transport and kinetics at carbon nanotube–redox enzyme composite modified electrode biosensors. Part 2. redox enzyme dispersed in nanotube mesh of finite thickness . Int. J. Electrochem. Soc. , 4 : 1196 – 1236 .
- Ganjali , M R , Alipour , A , Riahi , S , Larijani , B and Norouzi , P . 2009 . Quantitative analysis of pseudoephedrine in formulation by potentiometric membrane sensor; computational investigation . Int. J. Electrochem. Soc. , 4 : 1262 – 1276 .
- Wan , Q , Wang , X , Yu , F , Wang , X and Yang , N . 2009 . Effects of capacitance and resistance of MWNT-film coated electrodes on voltammetric detection of acetaminophen . J. Appl. Electrochem. , 39 : 1145 – 1151 . doi: 10.1007/s10800-008-9773-2
- Moghaddam , A B , Ganjali , M R , Saboury , A A , Moosavi-Movahedi , A A and Norouzi , P . 2008 . Electrode position of nickel oxide nanoparticles on glassy carbon surfaces: Application to the direct electron transfer of tyrosinase . J. Appl. Electrochem. , 38 : 1233 – 1239 . doi: 10.1007/s10800-008-9541-3
- Filiz , K , Tayfun , V , Cem , B , Baki , D E and Serdar , A . 2011 . Carbon nanotube-chitosan modified disposable pencil graphite electrode for Vitamin B(12) analysis . Colloids Surf. B: Biointerfaces , 87 : 18 – 22 . doi: 10.1016/j.colsurfb.2011.03.030
- Fotouhi , L , Nemati , M and Heravi , M M . 2011 . Electrochemistry and voltammetric determination of furazolidone with a multi-walled nanotube composite film-glassy carbon electrode . J. Appl. Electrochem. , 41 : 137 – 142 . doi: 10.1007/s10800-010-0216-5
- Fotouhi , L , Raei , F , Heravi , M M and Nematollahi , D . 2010 . Electrocatalytic activity of 6,7-dihydroxy-3-methyl-9-thia-4,4a-diazafluoren-2-one/multi-wall carbon nanotubes immobilized on carbon paste electrode for NADH oxidation: Application to the trace determination of NADH . J. Electroanal. Chem. , 639 : 15 – 20 . doi: 10.1016/j.jelechem.2009.10.013
- Fotouhi , L and Alahyari , M . 2010 . Electrochemical behavior and analytical application of ciprofloxacin using a multi-walled nanotube composite film-glassy carbon electrode . Colloids Surf. B: Biointerfaces , 81 : 110 – 114 . doi: 10.1016/j.colsurfb.2010.06.030
- Britto , P J , Santhanam , K SV and Ajayan , P M . 1996 . Carbon nanotube electrode for oxidation of dopamine . Bioelectrochem. Bioenerg. , 41 : 121 – 125 . doi: 10.1016/0302-4598(96)05078-7
- Astrid , M V , Maria , E , Carrera , B , Dietrich , V B and Carls , B . 1984 . The oxidative voltammetric behaviour of some sulphonamides at the glassy carbon electrode . Anal. Chim. Acta , 159 : 119 – 127 . doi: 10.1016/S0003-2670(00)84288-9
- Salimi , A , Banks , C E and Compton , R G . 2004 . Abrasive immobilization of carbon nanotubes on a basal plane pyrolytic graphite electrode: application to the detection of epinephrine . Analyst , 129 : 225 – 228 . doi: 10.1039/b315877b
- Ye , J S , Wen , Y , Zhang , W D , Cui , H F , Xu , G Q and Sheu , F S . 2005 . Electrochemical biosensing platforms using phthalocyanine-functionalized carbon nanotube electrode . Electroanal. , 17 : 89 – 96 . doi: 10.1002/elan.200403124
- Siswana , M P , Ozoemena , K I and Nyokong , T . 2006 . Electrocatalysis of asulam on cobalt phthalocyanine modified multi-walled carbon nanotubes immobilized on a basal plane pyrolytic graphite electrode . Electrochim. Acta , 52 : 114 – 122 . doi: 10.1016/j.electacta.2006.03.090
- Salimi , A , Miranzadeh , L and Hallaj , R . 2008 . Amperometric and voltammetric detection of hydrazine using glassy carbon electrodes modified with carbon nanotubes and catechol derivatives . Talanta , 75 : 147 – 156 .
- Shap , M , Petersson , M and Edstrom , K . 1979 . Preliminary determinations of electron transfer kinetics involving ferrocene covalently attached to a platinum surface . J. Electroanal. Chem. , 95 : 123 – 130 . doi: 10.1016/S0022-0728(79)80227-2
- Laviron , E . 1979 . General expression of the linear potential sweep voltammogram in the case of diffusionless electrochemical systems . J. Electroanal. Chem. , 101 : 19 – 28 . doi: 10.1016/S0022-0728(79)80075-3
- Torres-Lapasio , J R , Ruiz-Angel , M J , Garcia-Alvarez-Coque , M C and Abraham , M H . 2008 . Micellar versus hydro-organic reversed-phase liquid chromatography: A solvation parameter-based perspective . J. Chromatogr. A , 1182 : 176 – 196 . doi: 10.1016/j.chroma.2008.01.010
- Bard , A J and Faulkner , L R . 2001 . Electrochemical Methods: Fundamentals and Applications , New York : Wiley .
- Miller , J C and Miller , J N . 1992 . Statistical for Analytical Chemistry , 2nd , Chichester, UK : Eliis Horwood Ltd. .
- Braga , O C , Campestrini , I , Vieira , I C and Spinelli , A . 2010 . Sulfadiazine determination in pharmaceuticals by electrochemical reaction on a glassy carbon electrode . J. Braz. Chem. Soc. , 21 : 813 – 820 . doi: 10.1590/S0103-50532010000500008
- Liua , M , Xua , L , Chenga , W , Zenga , Y and Yana , Z . 2008 . Surface-modified CdS quantum dots as luminescent probes for sulfadiazine determination . Spectrochim. Acta A Mol. Biomol. Spectrosc. , 70 : 1198 – 1202 . doi: 10.1016/j.saa.2007.10.040