Abstract
A large number of MnO2 nanowires were fabricated by a facile hydrothermal method. The nanowires have a tetragonal pyrolusite structure and a smooth surface. The common bulk defects such as dislocations, twinnings and stacking faults are not detected by HRTEM measurement. The magnetisation dependence of temperature indicates that the magnetisation, linearly and monotonically, increases with decreasing temperature in the range 300–80 K, revealing the paramagnetic properties of the nanowires. The first discharge capacity reaches 223.5 mA h g−1, and the value of capacity steadily decreases during the following cycles, down to an acceptable 122.3 mA h g−1 after 25 cycles. The high surface ratio of nanowires is the main reason for the excellent discharge cycle property of the MnO2 nanowires.
1. Introduction
In the past decades, one-dimensional (1-D) nanostructures including nanowires, nanoribbons and nanotubes have attracted considerable attention due to their promising applications in numerous areas such as nanoelectronics, nano-optoelectronics, nanosensors and solar energy storages Citation1–14. As an important functional inorganic material, manganese dioxide (MnO2) has numerous applications in catalysis, ion exchange, molecular adsorption, rechargeable batteries and supercapacitors Citation15–23. Recently, much attention has focused on the synthesis of 1D MnO2 nanostructures because of its promising applications in catalysis, rechargeable batteries, ion sieves and supercapacitors Citation24–30. Up to now, various methods including sol-gel route, wet chemical method, template-assisted method and hydrothermal route have been developed to synthesise 1-D MnO2 nanostructures Citation31–36. Among these methods, the hydrothermal route is the most useful and efficient because the method can control the nanostructures by choosing the reaction temperature, concentration and time. Moreover, the method is a low cost one and the yield is high enough to meet the industrial demand. In this study, a facile hydrothermal route was developed to fabricate a large number of MnO2 nanowires. The chemical composition, microstructure and magnetic properties were investigated, which present some new features on the MnO2 nanowires.
2. Experiment
All the chemicals were of analytic grade and used without further purification. In the experiment, 0.002 mol manganese chloride tetrahydrate (MnCl2 · 4H2O), 0.001 mol potassium permanganate (KMnO4) and 30 mL distilled water were added into 50 mL Teflon-lined stainless steel autoclaves and stirred for half an hour. Then, 10 mL H2SO4 (2 M) was slowly added into the mixed solution under vigorous stirring. Finally, the autoclave was sealed and maintained at 150°C for 24 h. After the autoclave was cooled to room temperature, precipitates were taken out and washed with distilled water for several times and dried in air for further characterisation. The chemical reaction involves two steps:
1. | Mn2+ + 2H2O → MnO2 + 4 H+ | ||||
2. |
|
The black precipitates are the MnO2 products, which can be confirmed by the following characterisation. Phase identifications were performed on X-ray diffractometer (PANalytical X’PERT MPD) with a Cu-Kα radiation (λ = 1.5406 Å) operated at 40 kV and 40 mA. Morphology and microstructure of the products were characterised by field-emission scanning electron microscopy (SEM; FEI XL30 S-FEG) and transmission electron microscopy (TEM; Philips CM200) equipped with energy-dispersive X-ray spectroscopy (EDS). The magnetic properties were measured by Physical Property Measurement System (PPMS, Quantum Design). The as-synthesised MnO2 nanowires were employed as cathode active materials for rechargeable lithium-ion cells. The detailed measurement process is in accordance with the literature Citation37.
3. Results and discussion
shows the XRD patterns of the as-prepared precipitates. All the diffraction peaks in can be indexed to pure tetragonal β-MnO2 with lattice constants a = 4.392 Å and c = 2.871 Å, agreeing well with the standard card (ICDD-PDF No: 24-0735). Impurities such as α- and γ-structures are not detected within the instrumental resolution. The broad diffraction peaks are maybe due to the nano-size effects on the products. The corresponding SEM image indicates that the precipitates consist of a large number of nanowires, as shown in . The average diameter and length of these nanowires are ca. 30 nm and 1 µm, respectively, further confirming that the broad diffraction peaks are induced by the nano-size effects. The corresponding TEM image further indicates regular and dispersed nanowire formation, as shown in . shows the TEM image of an individual MnO2 nanowire. The nanowire has a smooth surface and it is straight along the growth direction. The corresponding EDS spectrum () indicates that the nanowire is mainly composed of Mn and O elements. The atomic ratio of Mn and O is about 1:1.926, further confirming the MnO2 formation of the nanowires. The HRTEM image indicates that the clear interplanar spacing of the lattice planes is ca. 0.312 nm, agreeing well with the distance value of (1 1 0) lattice planes of the tetragonal MnO2, as shown in . The surface of the nanowire is smooth and the common thin amorphous layer cannot be detected. Moreover, the common bulk defects such as dislocations, twinnings and stacking faults are not detected, revealing that the nanowires are largely free of the bulk defects.
Figure 2. (a) TEM image of a large area of MnO2 nanowires; (b) TEM image of a individual MnO2 nanowire; (c) EDS spectrum of the MnO2 nanowires; and (d) HRTEM image of the MnO2 nanowire.
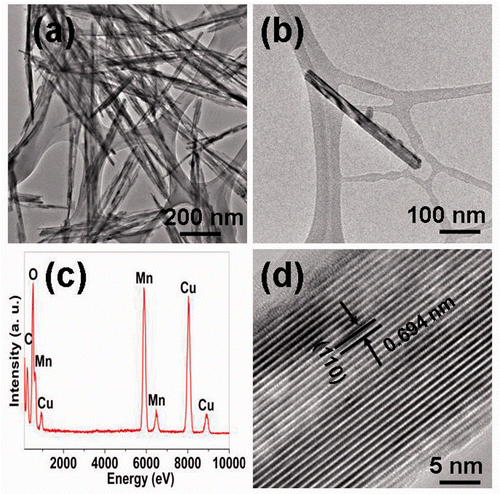
Magnetisation dependence of temperature was measured under a field-cooled mode in the magnetic field of 3000 Oe at temperature range 80–300 K, as shown in . The magnetisation of MnO2 nanowires was weak and its values were ca. 4.44, 5.85, 7.14 memu g−1 measured at 300, 150 and 80 K, respectively. The magnetisation, linearly and monotonically, increases with decreasing temperature, which means that the MnO2 nanowires are paramagnetic. shows the magnetic hysteresis loops measured at 300, 150 and 80 K, respectively. Linear magnetic hysteresis loops at 300, 150 and 80 K are clearly observed, further demonstrating the paramagnetic properties of MnO2 nanowires.
Figure 3. (a) Temperature dependence of magnetisation measured under a field-cooled mode at the temperature range from 80 to 300 K and (b) (colour online) magnetic hysteresis loop of MnO2 nanowires measured at 300, 150, and 80 K, respectively.
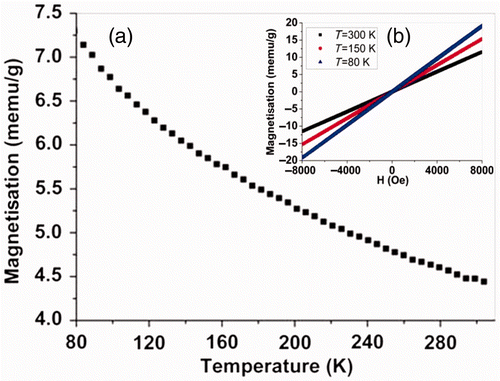
shows the discharge capacities of Li–MnO2 cells over the first 25 cycles. The first discharge capacity reaches 223.5 mA h g−1. The value is slightly higher than that observed in the data of commercial β-MnO2 powders and reported 1-D nanostructures, and much higher than that of bulk materials Citation37,Citation38. The value of discharge capacity is relatively decreased rapidly with cycling from 1 to 10 times, and is steadily decreased in the following cycles, down to an acceptable capacity of 122.3 mA h g−1 after 25 cycles. These results indicate that the reversibility during Li insertion/extraction reactions can easily occur in the Li–MnO2 nanowire cells, and the reactions have a steady loss of capacity on cycling. Moreover, because of the high surface ratio nature of the nanowires, the capacities of the nanowires are much higher than that of bulk materials and the aggregated nanostructures.
4. Conclusions
Tetragonal β-MnO2 nanowires were obtained by a facile hydrothermal method. The average length and diameter of the nanowires are about 1 µm and 30 nm, respectively. The magnetisation dependence of temperature indicates that the magnetisation values of MnO2 nanowires are ca. 4.44, 5.85, 7.14 memu g−1 at 300, 150 and 80 K, respectively. The magnetisation, linearly and monotonically, increases with decreasing temperature, indicating the paramagnetic properties of the MnO2 nanowires. The first discharge capacity reaches 223.5 mA h g−1, much higher than that of bulk materials. The value of capacity is steadily decreased during the following cycles, down to an acceptable capacity of 122.3 mA h g−1 after 25 cycles. We deduce that high surface ratio of nanowires facilitates the Li insertion/extraction reactions in the Li–MnO2 nanowire cell, resulting in an excellent cycle property.
Acknowledgements
This study was financially supported by the National Natural Science Foundation of China (nos 51002143, 51072184) and the Natural Science Foundation of Henan Provincial Educational Department (no. 2010B140015).
References
- Lou , X W , Deng , D , Lee , J Y , Feng , J and Archer , L A . 2008 . Self-supported formation of needlelike Co3O4 nanotubes and their application as lithium-ion battery electrodes . Adv. Mater. , 20 : 258 – 262 .
- Lei , M , Hu , Q R , Wang , X , Wang , S L and Tang , W H . 2010 . Facile route to straight ZnGa2O4 nanowires and their catholuminescence properties . J. Alloys Compd. , 489 : 663 – 666 .
- Dong , Y J , Tian , B Z , Kempa , T J and Lieber , C M . 2009 . Coaxial group III-nitride nanowire photovoltaics . Nano Lett. , 9 : 2183 – 2187 .
- Teng , X W , Han , W Q , Ku , W and Hucker , M . 2008 . Synthesis of ultrathin palladium and platinum nanowires and a study of their magnetic properties . Angew. Chem. Int. Ed. , 47 : 2055 – 2058 .
- Lei , M , Yang , H , Li , P G and Tang , W H . 2008 . Synthesis of GaN nanowires on gold-coated SiC substrates by novel pulsed electron deposition technique . Appl. Surf. Sci. , 254 : 1947 – 1952 .
- Acharya , S , Gautam , U K , Sasaki , T , Bando , Y , Golan , Y and Ariga , K . 2008 . Ultra narrow PbS nanorods with intense fluorescence . J. Am. Chem. Soc. , 130 : 4594 – 4595 .
- Lei , M , Hu , Q R , Wang , S L and Tang , W H . 2010 . Structural and optical properties of Al-doped SnO2 nanowires . Mater. Lett. , 64 : 19 – 21 .
- Carlson , L J , Maccagnano , S E , Zheng , M , Silcox , J and Krauss , T D . 2007 . Fluorescence efficiency of individual carbon nanotubes . Nano Lett. , 7 : 3698 – 3703 .
- Lei , M , Hu , Q R , Wang , S L and Tang , W H . 2009 . Catalytic-free growth of ZnGa2O4 nanowires on amorphous carbon layers . Mater. Lett. , 63 : 1928 – 1930 .
- Meng , G , Fang , X D , Zhou , Y K , Seo , J , Dong , W W , Hasegawa , S , Asahi , H , Tambo , H , Kong , M G and Li , L . 2010 . Ultra-low turn-on field from ultra-long ZnO nanowire arrays emitters . J. Alloys Compd. , 491 : 72 – 76 .
- Lei , M , Yang , H , Li , P G and Tang , W H . 2008 . Synthesis and characterization of straight and stacked-sheet AlN nanowires with high purity . J. Alloys Compd. , 459 : 338 – 342 .
- Barrelet , C J , Greytak , A B and Lieber , C M . 2004 . Nanowire photonic circuit elements . Nano Lett. , 4 : 1981 – 1985 .
- Lei , M , Yang , H , Guo , Y F , Song , B , Li , P G and Tang , W H . 2007 . Synthesis and optical property of high purity AlN nanowires . Mater. Sci. Eng. B , 143 : 85 – 89 .
- Zhang , L , Zaric , S , Tu , X , Wang , X , Zhao , W and Dai , H . 2008 . Assessment of chemically separated carbon nanotubes for nanoelectronics . J. Am. Chem. Soc. , 130 : 2686 – 2691 .
- Kijima , N , Ikeda , T , Oikawa , K , Izumi , F and Yoshimura , Y . 2004 . Crystal structure of an open-tunnel oxide alpha-MnO2 analyzed by Rietveld refinements and MEM-based pattern fitting . J. Solid State Chem. , 177 : 1258 – 1267 .
- Espinal , L , Suib , S L and Rusling , J F . 2004 . Electrochemical catalysis of styrene epoxidation with film of MnO2 nanoparticles and H2O2 . J. Am. Chem. Soc. , 126 : 7676 – 7682 .
- Wang , X and Li , Y D . 2003 . Synthesis and formation mechanism of manganese dioxide nanowires/nanorods . Chem. Eur. J. , 9 : 300 – 306 .
- Mathew , V , Lim , J , Kang , J , Gim , J , Rai , A K and Kim , J . 2011 . Self-assembled mesoporous manganese oxide with high surface area by ambient temperature synthesis and its enhanced electrochemical properties . Electrochem. Commun. , 13 : 730 – 733 .
- Cheng , F , Tao , Z , Liang , J and Chen , J . 2008 . Template-directed materials for rechargeable lithium-ion battery . Chem. Mater. , 20 : 667 – 681 .
- Winter , M and Brodd , R J . 2004 . What are batteries, fuel cells and supercapacitors? . Chem. Rev. , 104 : 4245 – 4269 .
- Reddy , A LM , Shaijumon , M M , Gowda , S R and Ajayan , P M . 2009 . Coaxial MnO2/carbon nanotubes arrays electrodes for high-performance lithium batteries . Nano. Lett. , 9 : 1002 – 1006 .
- Jiao , F , Harrison , A and Bruce , P G . 2007 . Order three-dimensional arrays of monodispersed Mn3O4 nanoparticles with a core-shell structure and spin-glass behavior . Angew. Chem. Int. Ed. , 46 : 3946 – 3950 .
- Jiao , F and Bruce , P G . 2007 . Mesoporous crystalline beta-MnO2-a reversible positive electrode for rechargeable lithium batteries . Adv. Mater. , 19 : 657 – 660 .
- Hu , C C and Wang , C C . 2003 . Nanostructures and capacitive characteristics of hydrous manganese oxide prepared by electrochemical deposition . J. Electrochem. Soc. , 150 : A1079 – A1084 .
- Kunduraci , M and Amatucci , G G . 2006 . Synthesis and characterization of nanostructured 4.7 VLixMn1.5Ni0.5O4 spinels for high-power lithium-ion batteries . J. Electrochem. Soc. , 153 : 1345 – 1352 .
- Kuratani , K , Tatsumi , K and Kuriyama , N . 2007 . Manganese oxide nanorod with 2 x 4 tunnel structure: Synthesis and electrochemical properties . Cryst. Growth Des. , 7 : 1375 – 1377 .
- Wei , M , Konishi , Y , Zhou , H , Sugihara , H and Arakawa , H . 2005 . Synthesis of single-crystal manganese dioxide nanowires by a soft chemical process . Nanotechnology , 16 : 245 – 249 .
- Wu , M S . 2005 . Electrochemical capacitance from manganese oxide nanowire structure synthesized by cyclic voltammetric electrodeposition . Appl. Phys. Lett. , 87 : 153102(1) – 153102(3) .
- Zhong , C , Wang , J Z , Zhu , Z Z , Chou , S L , Chen , Z X , Li , Y and Liu , H K . 2010 . Hydrothermal synthesis of nanostructured MnO2 under magnetic field rechargeable lithium batteries . J. Solid State Electrochem. , 14 : 1743 – 1747 .
- Li , G , Jiang , L , Pang , H T and Peng , H . 2007 . Synthesis of gamma-MnO2 single-crystalline nanobelts . Mater. Lett. , 61 : 3319 – 3322 .
- Sugantha , M , Ramakrishnan , P A , Hermann , A M , Warmsingh , C P and Ginley , D S . 2003 . Nanostructured MnO2 for Li batteries . Int. J. Hydrogen Energy , 28 : 597 – 600 .
- Wang , H E , Lu , Z G , Qian , D , Fang , S and Zhang , J . 2008 . Facile synthesis and electrochemical characterization of hierarchical alpha-MnO2 spheres . J. Alloys Compd. , 466 : 250 – 257 .
- Kim , T W , Yoo , H , Kim , I Y , Ha , H W , Han , A R , Chang , J S , Lee , J S and Hwang , S J . 2011 . A composite formation route to well-crystalline manganese oxide nanocrystals: High catalytic activity of manganate-alumina nanocomposites . Adv. Funct. Mater. , 21 : 2301 – 2310 .
- Li , F , Wu , J F , Qin , Q H , Li , Z and Huang , X T . 2010 . Facile synthesis of gamma-MnOOH micro/nanorods and their conversion to beta-MnO2 Mn3O4 . J. Alloys Compd. , 492 : 339 – 346 .
- Gong , L Y , Su , L H and Jiang , H Y . 2011 . Rapid synthesis of homogeneous MnO2/multi-wall carbon nanoturbes nanostructure and its electrochemical capacitive behavior . Mater. Lett. , 65 : 1588 – 1590 .
- Teng , F , Santhanagopalan , S , Wang , Y and Meng , D D . 2010 . In-stiu hydrothermal synthesis of three-dimensional MnO2-CNT nanocomposites and their electrochemical properties . J. Alloys Compd. , 499 : 259 – 264 .
- Cheng , F Y , Zhao , J Z , Song , W N , Li , C S , Ma , H , Chen , J and Shen , P W . 2006 . Facile controlled synthesis of MnO2 nanostructures of novel shapes and their application in batteries . Inorg. Chem. , 45 : 2038 – 2044 .
- Cui , H J , Huang , H Z , Fu , M L , Yuan , B L and Pearl , W . 2011 . Facile synthesis and catalytic properties of single crystalline beta-MnO2 nanorods . Catal. Commun. , 12 : 1339 – 1343 .