Abstract
Zinc sulphide (ZnS) nanotubes were synthesised via simple surfactant emulsion template under hydrothermal conditions. X-ray diffraction, scanning electron microscopy and transmission electron microscope were used to characterise the nanotubes. The results indicate that the nanotubes are composed of nanoparticles. The diameters of the nanotubes vary from 300 to 700 nm and lengths range from 1 to 2 µm. In addition, it is found that the reaction time, reaction temperature and cetyltrimethylammonium bromide play key roles in the phase and morphology control of ZnS nanotubes. Furthermore, room temperature photoluminescence was recorded to investigate the optical property of the obtained product. The stable and strong green emission band centred at 513 nm was attributed to some self-activated centres, probably vacancy states or interstitial states related to the peculiar structure.
Keywords:
1. Introduction
The discovery of carbon nanotubes by Iijima Citation1 has put the scientific community into a kind of continuous gold-rush mood. Since then, many efforts have been made worldwide to synthesise and determine the properties of various nanotubes. Zinc sulphide (ZnS) is one of the most widely studied IIB-VI group semiconductor compounds with wide band gap energy of 3.7 eV Citation2 at 300 K. It is well-known for its photoluminescence (PL) Citation3 and electroluminescence Citation4 properties. Over the past few years, various nanoforms of ZnS such as nanorods, nanowires, nanoribbons and nanospheres have been reported Citation5–11. However, there are few reports on the synthesis of ZnS nanotubes Citation12.
In this article, a facile solution route was used to synthesise ZnS nanotube at low temperature (120°C) using cetyltrimethylammonium bromide (CTAB) as surfactant emulsion template.
2. Experiment
For a typical preparing process, 4 g CTAB was added into distilled water to form transparent emulsion under intensive stirring for 30 min at 60°C and cooled down to room temperature naturally (solution A). 0.01 mol of ZnCl2 was added into distilled water and the pH value of the ZnCl2 solution was adjusted to 12 with ammonia to form complexes (solution B). Solution B was added into solution A and stirred for 10 min, then 0.01 mol of thiourea (TU) was added into the mixture and stirred for another 10 min. Finally, the solution was transferred into a Teflon-lined autoclave. The autoclave was maintained at 120°C for 20 h and cooled down to room temperature naturally. The white precipitate was collected and washed with absolute ethanol and distilled water for several times. Then, ZnS sample was obtained by centrifugation and drying in vacuum at 60°C.
The structures and morphologies of the as-prepared products were characterised with X-ray diffraction (XRD, Philips PW with Cu-Kα radiation), field emission scanning electron microscope (SEM, FEI Sirion-200), a transmission electron microscope (TEM, JEM-2010) and selected area electron diffraction (SAED). Room temperature PL was recorded with an Edinburgh luminescence spectrometer (FLS 920) using Xe lamp as the excitation source.
3. Results and discussion
XRD was used to determine the phase of the as-prepared product. All the diffraction peaks () can be indexed to cubic phase ZnS (JCPDS Card No. 01-0792). No other diffraction peaks corresponding to oxides or impurities can be observed, indicating that the final product is highly crystallised with high purity under current synthetic conditions.
The morphology of the as-prepared product was examined by SEM. shows the low-magnification SEM image of the product. Large-scale ZnS nanotubes can be seen clearly. Some of the nanotubes are open-ended and some of them are close-ended. The diameters of the nanotubes vary from 300 to 700 nm and lengths are in the range of 1 to 2 µm. The high-magnification SEM image shown in demonstrates clearly that the nanotubes are composed of nanoparticles. Further structural characterisation of the ZnS nanotubes was performed by TEM. also shows that the nanotubes are composed of small nanoparticles. The diameter of the nanotube is about 400 nm and the length is about 1 µm, which is consistent with the results of SEM. The SAED pattern displayed in Figure 2(d) shows that there are some diffraction spots and discontinuous concentric rings, which indicates that the obtained ZnS nanotubes are a kind of mixed crystal structure including single crystal and polycrystal. The diffraction rings can be indexed to (111), (220) and (311) planes of the cubic ZnS phase.
Figure 2. (a) Low-magnification SEM images of ZnS nanotubes, (b) high-magnification SEM images of ZnS nanotubes, (c) TEM image of individual nanotube and (d) corresponding SAED pattern.
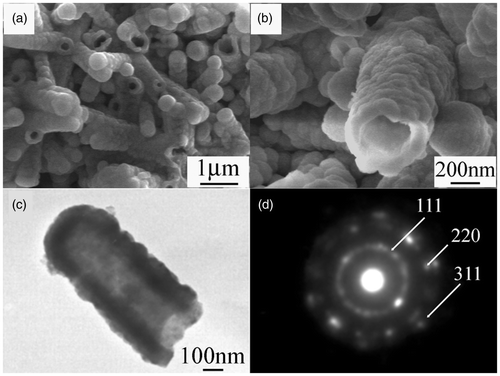
Further studies show that the reaction time is very important for the formation of ZnS nanotubes. When the reaction time was 3 h, the as-synthesised products were the mixture of short nanotubes and nanoparticles (). Prolonging the reaction to 60 h, most of the nanotubes turned to be nanorods (). In addition, the reaction temperature also plays an important role for the phase and morphology of the as-synthesised products. When the reaction temperature was lower than 80°C, the products were the mixture of ZnS and ZnO. It is because of the gradual dissolution of Tu and the slow rate release of S2− at lower temperature. Chen has reported that Tu can dissolve in water and release S2− ions in aqueous solution rapidly at elevated temperature under hydrothermal process Citation13. So, it is probably that the transformation of ZnO to ZnS at 80°C needs more time compared with that at 120°C. Furthermore, it is found that CTAB is crucial for the formation of ZnS nanotubes. If CTAB is not added into the solution, the final products will be solid ZnS nanospheres composed of nanoparticles ().
Figure 3. SEM images of the products prepared at 120°C for (a) 3 h, (b) 60 h, (c) without CTAB and (d) the corresponding TEM image.
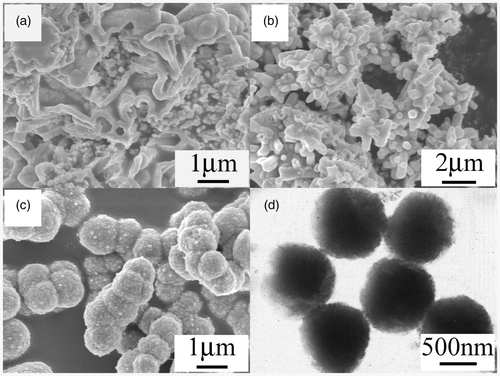
From the above analysis, the reactions under hydrothermal condition could be described by the following equations:
Initially, the ZnO nanoparticles were formed by the reaction between and OH−. At the same time, Tu was hydrolysed and released S2− under elevated temperature and pressure. As the thermodynamic constant of ZnO (ΔG (f
0) (400 K) = −366 kJ mol−1) is bigger than that of ZnS (ΔG (f
0) (400 K) = −220 kJ mol−1), the formation of ZnO is preferential than ZnS at the same experimental condition. Then ZnO or Zn2+ reacted with S2− to form ZnS nanocrystals.
The proposed mechanism for the formation of ZnS nanotubes via surfactant soft-template inducing growth could be divided into three steps. (a) The CTAB cationic surfactant condensed into column aggregation. (b) When the temperature of Teflon-line autoclave increased gradually, the ZnO nanoparticles began to aggregate on the surface of the surfactant and react with H2S or S2− to form ZnS nanoparticles. (c) After the reaction completed, the surfactant was removed by washing with ethanol and distilled water, finally the ZnS nanotubes was formed.
PL was carried out to investigate the optical property of ZnS nanotubes. The emission spectrum shown in reveals that the as-synthesised product has a stable and strong green emission band centred at 513 nm. Previous reports indicate that the nanocrystalline ZnS doped with Cu2+ and Mn2+ can show a visible-region emission from blue to green Citation14,Citation15. Wang et al. Citation16 and Li and Wang Citation17 observed this emission peak too and explained the peak as Au-catalyst impurity-related deep level emission. But in this work, we have neither incorporated any dopants into ZnS nanotubes nor used Au as the catalyst. The possibilities of foreign materials as the reason for the green emission observed in ZnS nanotubes could be ruled out. Thus the green emission could be attributed to some self-activated centres, probably vacancy states or interstitial states related to the peculiar structure.
4. Conclusion
ZnS nanotubes were successfully synthesised via hydrothermal method with the presence of CTAB as surfactant. It is found that the reaction time, reaction temperature and CTAB are very important for the formation of ZnS nanotubes. The PL peak at about 513 nm is attributed to some self-activated centres. The one-step synthetic method employed in this work is relatively simple and environment-benign, which may be used to prepare other metal sulphide nanotubes.
Acknowledgements
This work was financially supported by the NSF of China (Grant Nos. 50532070, 60876014 and 60976014), the Knowledge Innovation Program of Chinese Academy of Sciences (grant no. KJCX3.5YW.W01) and the NSF of Henan province (grant nos. 072300410180, 092300410131 and 092300410156). The project is also sponsored by program for science and technology innovation talents in universities of Henan province (Grant No. 2008HASTIT029).
References
- Iijima , S . 1991 . Helical microtubules of graphitic carbon . Nature , 354 : 56 – 58 .
- Sooklal , K , Cullum , B S , Angel , S M and Murphy , C J . 1996 . Photophysical properties of ZnS nanoclusters with spatially localized Mn2 + . J. Phys. Chem. , 100 : 4551 – 4555 .
- Falcony , C , Garcia , M , Ortiz , A and Alonso , J C . 1992 . Luminescent properties of ZnS:Mn films deposited by spray pyrolysis . J. Appl. Phys. , 72 : 1525 – 1527 .
- Tang , W and Cameron , D C . 1996 . Electroluminescent zinc sulphide devices produced by sol–gel processing . Thin Solid Films , 280 : 221 – 226 .
- Wang , M , Fei , G T , Zhu , X G , Wu , B , Kong , M G and Zhang , L D . 2009 . Density-controlled homoepitaxial growth of ZnS nanowire arrays . J. Phys. Chem. C , 113 : 4335 – 4339 .
- Hu , P A , Liu , Y Q , Fu , L , Cao , L C and Zhu , D B . 2004 . Self-assembled growth of ZnS nanobelt networks . J. Phys. Chem. B , 108 : 936 – 938 .
- Ye , C H , Fang , X S , Li , G H and Zhang , L D . 2004 . Origin of the green photoluminescence from zinc sulfide nanobelts . Appl. Phys. Lett. , 85 : 3035 – 3037 .
- Pradhan , N and Efrima , S . 2004 . Supercrystals of uniform nanorods and nanowires, and the nanorod-to-nanowire oriented transition . J. Phys. Chem. B , 108 : 11964 – 11970 .
- Kar , S , Biswas , S and Chaudhuri , S . 2005 . Nanometre to micrometre wide ZnS nanoribbons . Nanotechnology , 16 : 3074 – 3078 .
- Kar , S and Chaudhuri , S . 2005 . Controlled synthesis and photoluminescence properties of ZnS nanowires and nanoribbons . J. Phys. Chem. B , 109 : 3298 – 3302 .
- Zhang , Y and Li , Y D . 2004 . Synthesis and characterization of monodisperse doped ZnS nanospheres with enhanced thermal stability . J. Phys. Chem. B , 108 : 17805 – 17811 .
- Farhangfar , S , Yang , R B , Pelletier , M and Nielsch , K . 2009 . Atomic layer deposition of ZnS nanotubes . Nanotechnology , 20 : 325602
- Chen , X J , Xu , H F , Xu , N S , Zhao , F F , Lin , W J , Lin , G , Fu , Y L , Huang , Z L , Wang , H Z and Wu , M M . 2003 . Kinetically controlled synthesis of wurtzite ZnS nanorods through mild thermolysis of a covalent organic−inorganic network . Inorg. Chem. , 42 : 3100 – 3106 .
- Wang , M W , Sun , L D , Fu , X F , Liao , C H and Yan , C H . 2000 . Synthesis and optical properties of ZnS:Cu(II) nanoparticles . Solid State Commun. , 115 : 493 – 496 .
- Gan , L M , Liu , B , Chew , C H , Xu , S J , Chua , S J , Loy , G L and Xu , G Q . 1997 . Enhanced photoluminescence and characterization of Mn-doped ZnS nanocrystallites synthesized in microemulsion . Langmuir , 13 : 6427 – 6431 .
- Wang , Y W , Zhang , L D , Liang , C H , Wang , G Z and Peng , X S . 2002 . Catalytic growth and photoluminescence properties of semiconductor single-crystal ZnS nanowires . Chem. Phys. Lett. , 357 : 314 – 318 .
- Li , Q and Wang , C R . 2003 . Fabrication of wurtzite ZnS nanobelts via simple thermal evaporation . Appl. Phys. Lett. , 83 : 359 – 361 .