Abstract
The effect of electrolyte pH and annealing temperature on the formation of TiO2 nanotube arrays in connection with the photoelectrochemical response was investigated in this article. Well-aligned TiO2 nanotube arrays were fabricated by anodisation of Ti foil in an electrolyte consisting of 1 M of glycerol (85 wt% of glycerol and 15 wt% of water) with 0.5 wt% of NH4F at 30 V for 30 min. The pH of the electrolyte was varied from pH 1 to 7. With the increase of electrolyte pH to neutral condition, the length of the nanotube arrays was increased from ∼320 to 1100 nm. As-anodised TiO2 nanotube arrays were amorphous in nature. However, anatase phase was observed after annealing at 400°C and polycrystalline anatase and rutile phase could be observed by heating up to 500°C in air atmosphere. Based on the results obtained, the length and crystalline phases of TiO2 nanotube arrays affect the performance of photoelectrochemical response and photoconversion efficiency significantly.
1. Introduction
Today, our world's hunger for energy is a growing matter of concern. In recent years, the rise of the carbon dioxide (CO2) emission is one of the most serious global environmental problems due to the increase in energy consumption and lack of long-term sustainability of existing energy sources Citation1–5. In these aspects, we have to find out an alternate non-polluting and renewable energy source to meet our world's future needs. Many scientists believe that it should be superseded by an economy based on hydrogen Citation3,Citation6–9. This hydrogen economy has been established as a potential future energy and would have numerous benefits over the existing system. The novel feature of hydrogen is that it can be produced from water using solar energy. Both the energy (solar light) and reactive media (water) are readily available and are renewable Citation7–12. However, there are several large technological, social and economic barriers impeding the emergence of hydrogen energy technology. In order to steer the economy away from its dependency on fossil fuels and towards a hydrogen-based renewable energy, improvement of photoconversion efficiency in photoelectrochemical (PEC) system will be a monumental challenge.
PEC system is one of the most attractive methods to produce hydrogen which has high potential in hydrogen economy Citation13–16. N-type semiconducting titanium dioxide (TiO2) with band gap of approximately 3.2 eV has been widely investigated to meet the requirement of the PEC Citation14–17. TiO2 has attracted great attention owing to their excellent characteristic such as great capacity for oxidation, wide band gap, non-toxicity, low cost and long-term stability Citation15,Citation16. In spite of its promising properties, TiO2 nanoparticles are still far away from becoming a potential semiconductor for PEC cell. Despite its low cost, making high efficient PEC cell using TiO2 nanoparticles as photoanode is a challenge unless two important issues are addressed Citation18,Citation19. It is recognised that the performance of the PEC cell is restricted by electron loss in nanoparticle network and limitation of the photon absorption from illumination due to the small surface area. Therefore, achievement of high efficiency of TiO2 photoanode requires a suitable architecture that minimises the electron loss at nanostructure connections and maximises photons absorption Citation18–21. Such architecture ought to have high surface area in contact with the electrolyte species, such as nanotubes, nanowires and nanorods Citation18,Citation20–24.
Among these architectures, considerable attention has been focused on the development of TiO2 nanotube arrays by anodisation method. Studies have indicated that vertically oriented and highly ordered TiO2 nanotubes are of great importance due to their one-dimensional nature, ease of handling and simple preparation Citation25,Citation26. Moreover, the geometrical features of the anodised nanotube arrays can be tailored by controlling the process parameters. Therefore, in recent years, considerable efforts have been focused on synthesising highly ordered TiO2 nanotube arrays to achieve specific light absorption and propagation characteristics. In this article, a detailed study has been performed to evaluate the surface morphology of TiO2 nanotube arrays in different electrolyte pH values and discuss the influence of tube length and crystalline phase on the PEC performance. This consecutively will aid on building up a base level of knowledge regarding a suitable architecture of TiO2 nanotube arrays by utilising an electrolyte with different pH values and crystalline phases for better photoconversion efficiency.
2. Experimental
TiO2 nanotube arrays were grown by anodic oxidation of Ti foils (4 cm × 1 cm) with 99.6% purity of 0.127 mm thickness. Prior to anodisation, the Ti foils were degreased by sonication in ethanol for 30 min. The Ti foils were then rinsed in deionised water and dried with nitrogen stream. Anodisation was performed in a two-electrode configuration bath with the Ti foil as the anode and platinum foil as the counter electrode at 30 V for 30 min. The electrolyte was homogenised via magnetic stirring. The electrolyte was 1 M of glycerol (85 wt% of glycerol and 15 wt% of water) containing 0.5 wt% of NH4F. The pH of the electrolyte was adjusted to 1, 3, 5 and 7, with the addition of 0.1 M sulphuric acid (H2SO4) and 0.1 M sodium hydroxide (NaOH). The anodised Ti foils were cleaned using acetone and dried in nitrogen stream. The second set of experiment involves anodisation of the sample in electrolyte with pH 7 for further investigation. The effect of annealing temperature on the crystallinity of TiO2 nanotube arrays was investigated. The annealing temperature was varied from 200°C, 400°C and 500°C for 2 h in air atmosphere.
The morphology of the anodised Ti foils was viewed using a field-emission scanning electron microscope (FESEM) Zeiss SUPRA 35VP at a working distance of around 1 mm. In order to obtain the thickness of the anodic oxide formed, cross-sectional measurements were carried out on mechanically bent samples, where a partial lift-off of the anodic layer occurred. Phase determination of the anodic layer was determined by X-ray diffraction (XRD) analysis by using Philips, PW 1729, operated at 45 kV and 40 mV.
The PEC properties of the samples were characterised using a three electrodes’ PEC cell with TiO2 nanotube arrays as the working photoelectrode, platinum rod as the counter electrode and saturated calomel electrode (SCE) was used as the reference electrode. 1 M potassium hydroxide (KOH) with 1 wt% of ethylene glycol solution was used as the electrolyte in this experiment. All the three electrodes were connected to a potentiostat (µAutolab III). A 800 W/m2 xenon lamp (Zolix LSP-X150) was used to produce a largely continuous and uniform spectrum and 100% transmittance of the light was permitted by the quartz glass as the xenon lamp shined on the TiO2 nanotube arrays (photoanode). The xenon lamp was switched on after the three electrodes were connected to the potentiostat. Then, a linear sweep potentiometry was sweeped from −0.5 to 1.0 V at a scan rate of 5 mV/s and corresponding photocurrent was measured.
3. Results and discussion
3.1. Effect of electrolyte pH on the formation of TiO2 nanotube arrays
In this part of experiment, the effect of electrolyte pH on the morphology of TiO2 nanotube arrays is discussed. shows the FESEM images of the cross-section morphology of the anodised Ti foils in electrolyte that consists of 1 M of glycerol (85 wt% of glycerol and 15 wt% of water) with 0.5 wt% of dissolved NH4F at 30 V for 30 min with different electrolyte pH value. Insets are the surface morphology of the TiO2 nanotube arrays. For sample anodised at pH 1 (), nanotube with diameter of ∼100 nm and length of ∼320 nm was produced. With further increase in the electrolyte pH to 3 (), the length of the nanotube became longer, ∼730 nm with an average diameter of 100 nm. The length of the nanotubes increased up to ∼1000 nm with the diameter of ∼105 nm when the electrolyte pH reached 5 (). In this condition, the shape of the nanotubes became much more uniform compared with those produced at a low pH value. Upon further increase of the electrolyte pH to 7 (), TiO2 nanotube arrays exhibited an average diameter of 105 nm and the length was ∼1100 nm. From these FESEM images, it is concluded that pH value of the electrolyte significantly affects the growth rate of the nanotube arrays but the diameter of the nanotube arrays is not strong dependence on the electrolyte pH value.
Figure 1. FESEM micrographs of the cross-section view of the TiO2 nanotube arrays formed by anodisation of titanium foil at different electrolyte pH values: (a) pH 1, (b) pH 3, (c) pH 5 and (d) pH 7. The inset is the top view showing the diameter of TiO2 nanotube arrays at different electrolyte pH values.
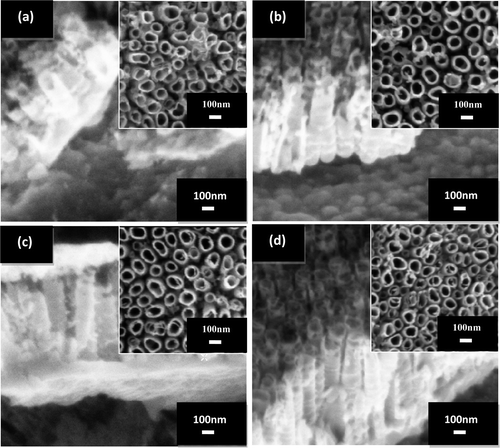
The TiO2 nanotube arrays length with electrolyte pH value is shown in . The growth rate of TiO2 nanotube arrays was accelerated with increasing electrolyte pH to neutral condition (pH 7). At initial stage of the anodisation, a compact oxide layer was formed due to the migration of the O2− ions towards the Ti/TiO2 interface which induces further growth of the barrier layer (Equations (1) and (2)). Pits were formed in the barrier layer and were attacked by the F− ions in the electrolyte to induce chemical dissolution (Equation (3)). The pits will grow inwards to form the tube structure. The formation of TiO2 nanotube arrays can be explained using the following equations Citation22,Citation23:
Low pH electrolyte (acidic condition) will produce high concentration of H+ ions, which reduces the hydrolysis ability of the electrolyte (Equation (1)). This subsequently results in lower concentration of oxygen and eventually leading to the formation of less dense oxide layer (Equation (2)). Therefore, the growth rate of nanotube arrays in lower pH (acidic condition) was decreased due to increasing chemical dissolution of TiO2 occurs at the tip and inside of the nanotubes (Equation (3)). The concentration of [TiF6]2− complex and H+ ions was higher at lower pH value (acidic condition), which eventually accelerate the chemical etching at the tip of the nanotube, leading to the formation of shorter tubes Citation24,Citation27. A schematic illustration regarding shorter nanotubes obtained at lower pH value (acidic condition) due to excessive H+ ions that increase the dissolution rate at the tip of nanotube and cause the local acidification and etching at the wall and inside of the tubes is shown in .
3.2. Effect of annealing temperature
In this part of experiment, XRD was used to investigate the effect of annealing temperature on the crystal growth and phase transition of TiO2 nanotube arrays. exhibits the crystal structure of the as-anodised and annealed TiO2 nanotube arrays at different annealing temperatures in air atmosphere. The result shows that the crystal structure of TiO2 is dependent on the annealing temperature. The XRD pattern of the as-anodised TiO2 nanotube arrays exhibited Ti phase only, which indicates the amorphous in nature of TiO2 (). The Bragg reflection of Ti substrate was detected at 2θ values of 35.09°, 38.43°, 40.17° and 53.01° in all the XRD patterns correspond to (1 0 0), (0 0 2), (1 0 1) and (1 0 2) of Ti substrate. However, the TiO2 nanotube arrays treated at 200°C showed only the Ti peaks and no characteristic peaks of anatase and rutile phase were observed. This result indicates that the annealing temperature was not sufficient to transform the amorphous phase into crystalline phase (). The presence of anatase phase was detected in the sample subjected to the annealing temperature 400°C (). The anatase phase present was identified by ICDD file 00-021-1272. At 2θ value of 25.5°, the peak corresponds to (1 0 1) anatase peak. In addition, several indistinct anatase peaks were found at 38.67°, 48.21°, 54.10° and 55.26° which correspond to (1 1 2), (2 0 0), (1 0 5) and (2 1 1) crystal planes for the anatase, respectively. The polycrystalline anatase- and rutile-type TiO2 were obtained by raising the annealing temperature up to 500°C (). Rutile phase is vaguely detected at 2θ value of 27.4° in the XRD spectra. It was reported that rutile grows at the interface between the barrier layer and Ti metal by thermal oxidisation of Ti metal because the constraints imposed by the nanotube walls make it difficult for the anatase crystals situated there to undergo phase transformation to rutile phase Citation28.
3.3. PEC response
To evaluate the PEC response, selected TiO2 nanotube arrays from the previous experiments were used as photoanode in the PEC process. The experimental conditions for the selected anodic samples with different nanotube length and crystal structure by varying the electrolyte pH and annealing temperature are summarised in . shows the photocurrent density in a linear sweep potential (−0.5 to 1.0 V) corresponding to light off and light on condition, with a light intensity of approximately 800 W/m2.
Figure 5. The I–V characteristics of TiO2 nanotube arrays with different tube length and crystal structure: (a) sample C, (b) sample D, (c) sample A and (d) sample B.
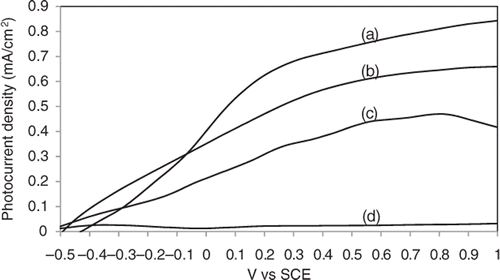
Table 1. Experimental conditions used to achieve different tube length and crystal structure of TiO2 nanotube arrays.
TiO2 nanotube arrays were immersed in 1 M of KOH with the addition of 1 wt% of ethylene glycol under irradiation. In this case, ethylene glycol was used as ‘sacrificial agent’ to reduce electron–hole recombination losses which significantly improved the photocurrent density of the integrated TiO2 nanotube photoanode Citation29. Electron–hole pairs were generated by the absorption of photons with energies greater than band gap and charges transfer occurred at the interface due to the difference in tendency of the two phases to gain and lose electrons Citation30. The net result is the formation of electric field of the depletion region which separates electron and hole. The excited electrons move to the counter electrode undergoes reduction process, whereas the generated holes move towards the semiconductor/electrolyte interface where water oxidation takes place Citation31.
All samples exhibited insignificant dark currents under light off condition. This indicates that no photo-induced electrons were transported through the sample due to inactive photocatalysis reaction Citation32. However, the photocurrent density increased under illumination (light on). Thus, TiO2 nanotube arrays can be concluded as a good photo-response semiconductor for the transfer and decay of the photo-induced electrons. Based on the results obtained, amorphous TiO2 nanotubes (Sample B) showed poor photocurrent density of 0.03 mA/cm2. The reason may be attributed to the low degree of anatase crystallinity, which increases the number of charge carrier for recombination centre. Besides, the amorphous phase comprises of various defects such as impurities, dangling bonds and micro-voids, which act as recombination centre and result in a decrease of photocurrent density. Most importantly, an amorphous TiO2 nanotube is basically unable to develop a regular depletion region. This suggests that improved crystallinity of the samples can effectively increase the photocurrent density due to the development of a depletion layer and the reduction in recombination centres Citation31,Citation33.
In contrast, the photocurrent density was found to increase for annealed samples. The sample C annealed at 400°C showed the highest photocurrent density throughout the potential window, suggesting that an efficient charge separation occurred and the photocurrent reached a high value of 0.85 mA/cm2 compared to sample D annealed at 500°C which is 0.65 mA/cm2. The increase in photocurrent density is likely attributed to the higher photogenerated electron/hole pairs caused by the higher content of anatase phase. This is in agreement with the results of Sclafani and Herrmann Citation34, which proposed that anatase phase is more photoactive than the rutile phase due to its larger specific surface area and easy hydroxylation Citation35. The difference in specific surface area, crystal size and pore size distribution between the anatase and rutile phase may cause defect sites. The charge transfer was obviously decreased due to the recombination centre of electron–hole pairs from the defect sites. This condition indicates that less photo-induced electrons can effectively transfer to the conduction band of TiO2 and eventually generates lower photocurrent response.
Among of the annealed samples, the shorter nanotube (sample A) fabricated in acidic electrolyte (pH 1) showed a lower photocurrent density, which implies that effective surface area plays an important role in PEC response. Sample A has shorter tube length (∼320 nm), which will eventually lead to a reduction in the effective surface area to a great extent and thus decreasing the number of active reactions sites. Based on this result, it can be concluded that less effective surface area of TiO2 nanotube arrays exhibited poor photon absorption from illumination. This is clearly to show that less photo-induced electrons have been effectively generated at both sides of the tube walls Citation36,Citation37.
3.4. Photoconversion efficiency
To estimate the quantitative correlation of light absorption to the different length and crystal structure of the TiO2 nanotubes, the photoconversion efficiency (η) of light to hydrogen energy was measured (). The photoconversion efficiency η was calculated based on Equation (4):
Figure 6. The corresponding photoconversion efficiencies of TiO2 nanotube arrays with different tube length and crystal structure: (a) sample C, (b) sample D, (c) sample A and (d) sample B.
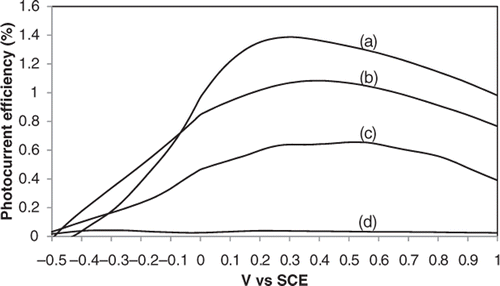
The highest photoconversion of about 1.4% was obtained for sample C annealed at 400°C. The decrease in photocurrent efficiency was as follows, 1.0%, 0.65% and 0.05% corresponding to sample D, sample A and sample B. The results show that there is a distinct dependency of the PEC properties on length of the nanotube and crystal structure of TiO2 nanotube arrays. In this aspect, the 400°C-annealed TiO2 nanotube arrays (sample C) exhibited the best PEC response.
4. Conclusion
From the experimental results of this research work, well-aligned and longer TiO2 nanotube arrays were successfully fabricated by anodisation in 1 M of glycerol with 0.5 wt% of dissolved NH4F at 30 V for 30 min under neutral condition (pH = 7). In neutral electrolyte, dissolution rate at the top of the nanotubes can be controlled by reducing the amount of H+ ions and thus longer TiO2 nanotube arrays can be fabricated at this pH value. Besides, it was found that by varying the annealing temperature on TiO2 nanotubes, it is possible to essentially modify the phase formation and transformation to tailor its PEC behaviour. Annealing at 200°C under air atmosphere did not reveal the crystalline peaks. However, transformation of the amorphous structure into crystalline anatase phase was observed after annealing at 400°C whilst polycrystalline anatase and rutile phase could be observed by heating up to 500°C. In this study, longer TiO2 nanotubes with high crystalline anatase phase showed higher photocurrent response with photoconversion efficiency of 1.4% and it has been acknowledged as the most efficient form for the PEC performance.
Acknowledgements
The authors thank Universiti Sains Malaysia for sponsoring this study under RU grant 814075 and the Fellowship USM and Research University Postgraduate Research Grant Scheme (Grant no. 80430146).
References
- Yu , K H and Chen , J H . 2009 . Enhancing solar cell efficiencies through 1-D nanostructure . Nanoscale Res. Lett. , 4 : 1 – 10 .
- Jefferson , M . 2006 . Sustainable energy development: Performance and prospects . Renewable Energy , 31 : 571 – 582 .
- Tromp , T K , Shia , R L , Allen , M , Eiler , J M and Yung , Y L . 2003 . Potential environmental impact of a hydrogen economy on the stratosphere . Science , 300 : 1740 – 1742 .
- Turner , J A . 1999 . A realizable renewable energy future . Science , 285 : 687 – 689 .
- Chen , G Y , Lee , M Y and Wang , G J . 2010 . Fabrication of dye-sensitized solar cells with a 3D nanostructured electrode . Int. J. Photoenergy , 2010 : 1 – 7 . Article ID 585621
- Kreuter , W and Hofmann , H . 1998 . Electrolysis: The important energy transformer in a world of sustainable energy . Int. J. Hydrogen Energy , 23 : 661 – 666 .
- Wang , H Z , Leung , D YC , Leung , M KH and Ni , M . 2009 . A review on hydrogen production using aluminium and aluminium alloys . Renew. Sustain. Energy Rev. , 13 : 845 – 853 .
- Mishra , P R , Shukla , P K , Singh , A K and Srivastave , O N . 2003 . Investigation and optimization of nanostructured TiO2 photoelectrode in regard to hydrogen production through photoelectrochemical process . Int. J. Hydrogen Energy , 28 : 1089 – 1094 .
- Mor , G K , Shankar , K , Varghese , O K and Grimes , C A . 2004 . Photoelectrochemical properties of titania nanotubes . Nano Lett. , 19 : 2989 – 2996 .
- Zhang , Z H , Hossain , M F and Takahashi , T . 2010 . Photoelectrochemical water splitting on highly smooth and ordered TiO2 nanotube arrays for hydrogen generation . Int. J. Hydrogen Energy , 35 : 8528 – 8535 .
- Yoong , L S , Chong , F K and Dutta , B K . 2009 . Development of copper-doped TiO2 photocatalyst for hydrogen production under visible light . Energy , 34 : 1652 – 1661 .
- Nagai , N , Takeuchi , M , Kimura , T and Oka , T . 2003 . Existence of optimum space between electrodes on hydrogen production by water electrolysis . Int. J. Hydrogen Energy , 28 : 35 – 41 .
- Fujishima , A and Honda , K . 1972 . Electrochemical photolysis of water at semiconductor electrode . Nature , 238 : 37 – 38 .
- Liu , Z Y , Pesic , B , Raja , K S , Rangaraju , R R and Misra , M . 2009 . Hydrogen generation under sunlight by self ordered TiO2 nanotube arrays . Int. J. Hydrogen Energy , 34 : 3250 – 3257 .
- Allam , N K , Shankar , K and Grimes , C A . 2008 . Photoelectrochemical and water photoelectrolysis properties of ordered TiO2 nanotubes fabricated by Ti anodization in fluoride-free HCl electrolytes . J. Mater. Chem. , 18 : 2341 – 2348 .
- Torres , G R , Lindgren , T , Lu , J , Granqvist , C G and Lindquist , S E . 2004 . Photoelectrochemical study of nitrogen-doped titanium dioxide for water oxidation . J. Phys. Chem. B. , 108 : 5995 – 6003 .
- Kitano , M , Matsuoka , M , Ueshima , M and Anpo , M . 2007 . Recent developments in titanium oxide-based photocatalysts . Appl. Catal., A , 325 : 1 – 14 .
- Grimes , C A , Varghese , O K and Ranjan , S . 2008 . Light, Water, Hydrogen: The Solar Generation of Hydrogen by Water Photoelectrolysis , New York : Springer .
- Ni , M , Leung , M KH , Leung , D YC and Sumathy , K . 2007 . A review and recent developments in photocatalytic water-splitting using TiO2 for hydrogen production . Renew. Sustain. Energy Rev. , 11 : 401 – 425 .
- Lee , N H , Oh , H J , Jung , S C , Lee , W J , Kim , D H and Kim , S J . Photocatalytic properties of nanotubular-shaped TiO2 powders with anatase phase obtained from titanate nanotube powder through various thermal treatments, Int. J. Photoenergy 2011 (2011), Article ID 327821, 7 pp. doi: 10.1155/2011/327821
- Baker , D R and Kamat , P V . 2009 . Disassembly, reassembly, and photoelectrochemistry of etched TiO2 nanotubes . J. Phys. Chem. C , 113 : 17967 – 17972 .
- Mor , G K , Varghese , O K , Paulose , M and Grimes , C A . 2005 . Transparent highly ordered TiO2 nanotube arrays via anodization of titanium thin films . Adv. Funct. Mater. , 15 : 1291 – 1296 .
- Ghicov , A and Schmuki , P . 2009 . Self-ordering electrochemistry: A review on growth and functionality of TiO2 nanotubes and other self-aligned MOx structures . Chem. Commun. , 40 : 2791 – 2908 .
- Macak , J M , Hildebrand , H , Marten-Jahns , U and Schmuki , P . 2008 . Mechanistic aspects and growth of large diameter self-organized TiO2 nanotubes . J. Electroanal. Chem. , 621 : 254 – 266 .
- Y.Q. Zhu, R. Whitby, R.Z. Ma, and S. Acquah, 1D nanomaterials, J. Nanomater. 2010 (2010), Article ID 597851, 3 pp. doi: 10.1155/2010/597851
- Zhu , W , Liu , X i , Liu , H , Tong , D , Yang , J and Peng , J . 2010 . An efficient approach to control the morphology and the adhesion properties of anodized TiO2 nanotube arrays for improved photoconversion efficiency . Electrochim. Acta , 56 : 2618 – 2626 .
- Sreekantan , S , Lockman , Z , Hazan , R , Tashihi , M , Tong , L K and Mohamed , A R . 2009 . Influence of electrolyte pH on TiO2 nanotube formation by Ti anodization . J Alloys Compd. , 485 : 478 – 483 .
- Mor , G K , Varghese , O K , Paulose , M , Shankar , K and Grimes , C A . 2006 . A review on highly ordered, vertically oriented TiO2 nanotube arrays: Fabrication, material properties, and solar energy applications . Sol. Energy Mater. Sol. Cells , 90, : 2011 – 2075 .
- Mohapatra , S K , Raja , K S , Mahajan , V K and Misra , M . 2008 . Efficient photoelectrolysis of water using TiO2 nanotube arrays by minimizing recombination losses with organic additives . J. Phys. Chem. C , 112 : 11007 – 11012 .
- Park , J H and Park , O O . Photoelectrochemical water splitting at titanium dioxide nanotubes coated with tungsten trioxide, Appl. Phys. Lett. 89 (2006), Article ID 163106, 3 pp. doi:10.1063/1.2357878
- Ahn , K S , Lee , S H , Dillon , A C , Tracy , E and Pitts , R . 2007 . The effect of thermal annealing on photoelectrochemical responses of WO3 thin films . J. Appl. Phys. , 101 Article ID 093524, 4 pp. doi: 10.1063/1.2729472
- Xie , Y , Zhou , L and Lu , J . 2009 . Photoelectrochemical behavior of titania nanotube array grown on nanocrystalline titanium . J. Mater. Sci. , 44 : 2907 – 2915 .
- Sun , L D , Zhang , S , Sun , X W and He , X D . 2010 . Effect of the geometry of the anodized titania nanotube array on the performance of dye-sensitized solar cells . J. Nanosci. Nanotechnol. , 10 : 4551 – 4561 .
- Sclafani , A and Herrmann , J-M . 1998 . Influence of metallic silver and of platinum-silver bimetallic deposits on the photocatalytic activity of titania (anatase and rutile) in organic and aqueous media . J. Photoch. Photobio. A – Chem. , 113 : 181 – 188 .
- Jaturong , J , Sarapong , P , Yoshikazu , S and Susumu , Y . 2007 . Synthesis and phohocatalytic activity for water-splitting reaction of nanocrystalline mesoporous titania prepared by hydrothermal method . J. Solid State Chem. , 180 : 1743 – 1749 .
- Wang , D , Liu , Y , Yu , B , Zhou , F and Liu , W . 2009 . TiO2 nanotubes with tunable morphology, diameter, and length: Synthesis and photo-electrical/catalytic performance . Chem. Mater. , 21 : 1198 – 1206 .
- Liu , Y B , Li , J H , Zhou , B X , Bai , J , Zheng , Q , Zhang , J and Cai , W . 2009 . Comparison of photoelectrochemical properties of TiO2-nanotube-array photoanode prepared by anodization in different electrolyte . Environ. Chem. Lett. , 7 : 363 – 368 .