Abstract
In the present investigation, alginate nanoparticles have been prepared and characterised by various techniques such as FTIR, SEM, particle size analysis and surface charge measurements. It was found from both the SEM and particle size analysis that average size of the particle was about 40 nm. The particles were loaded with insulin and the release kinetics of insulin was studied in PBS medium. The results indicated that when percent loading increases from 11.7 to 38.9, the released amount of insulin increased from 18% to 60% of the loaded drug. The effect of composition of nanoparticles, pH and temperature of the release medium was examined on the amount of released insulin. It was observed when that amount of alginate in the feed mixture was varied from 1.0 to 2.0 g, the prepared nanoparticles showed a decreasing tendency to release insulin. Similarly, upon increasing the concentration of crosslinker in the range 0.5–1.1 mM, the release of insulin constantly decreased. The chemical stability of the loaded drug was assessed especially under highly acidic conditions of artificial gastric juice and it was noticed that even in harsh acidic environment (pH 1.2) the insulin remains chemically stable. The invitro blood compatibility of nanoparticles was also investigated and it was found that for a definite composition of nanoparticles, protein adsorption and percent haemolysis were minimum which suggested for an optimum blood compatibility of alginate nanoparticles of definite composition. Thus, it can be conclusively stated that the calcium alginate nanoparticles prepared by emulsion crosslinking method show potential to be developed as oral formulation for insulin delivery.
1. Introduction
Recently major advancements in drug delivery technology of high molecular weight bioactive agents such as proteins and peptides are witnessed, and among these the insulin delivery is the most investigated one. Since oral drug administration is the most comfortable and effective means of drug delivery, exhaustive investigations have been directed to achieve a viable drug delivery formulation. Extensive efforts have been made to explore new administration routes to deliver poorly soluble macro drugs, such as insulin, which is often delivered to diabetic patients through subcutaneous injections and often cause itching, pain, allergic reactions and hyper insulinemia and lipodystrophy around the injection site Citation1,Citation2. Apart from these, the other disadvantages include low patient compliance, the high costs of a sterile manufacturing process and the need for qualified personal to administer the injections. Various administration routes of insulin delivery are also reported such as pulmonary delivery Citation3, transdermal delivery Citation4, nasal delivery Citation5 and oral delivery Citation6.
Pulmonary delivery of insulin makes use of inhalers which enable the drug to reach the deep lungs from where it can be quickly absorbed into the circulation. This administration route, however, has certain drawbacks such as adverse effects on pulmonary functions, low safety and poor tolerability.
The delivery of insulin through skin (transdermal delivery) could be another option for the drug administration but large size, hydrophilic nature and intracellular lipid layer of the stratum corneum make the drug permeation difficult. In intranasal delivery, the insulin is allowed to enter through nasal mucosa and thus directly enter the systemic circulation. The major obstacles for this type of administration include rapid mucociliary clearance, enzyme activity and low pH of the nasal epithelium.
It is widely accepted that among all the routes of drug administration the oral one is the most preferred one but at the same time its success is still a challenge to pharmacy and medical experts. However, its successful delivery may solve all the problems pertaining to the complications associated with its frequent administration. Thus, the fascination to design an oral insulin delivery system has resulted in extensive attempts to fabricate a formulation which could effectively and comfortably deliver insulin Citation7,Citation8. The success, however, is very little primarily due to the enzymatic degradation of protein and low levels of transport across the epithelial layer of the gastrointestinal (GI) tract, because of the large size, hydrophilicity and instability of these macromolecules Citation9.
In order to overcome these problems, many approaches have been adopted for protecting proteins such as encapsulation of the insulin within pH-sensitive hydrogels Citation10, liposomes Citation11, permeation enhancer, enzyme inhibitor Citation12 and nanoparticles Citation13. Among these approaches, nanoparticulate systems have attracted special interest for three reasons. First, nanoparticles are able to protect active agents from degradation Citation14. The second reason is related to improving drug transmucosal transport Citation15 and transcytosis by M cells. Third, the particulate systems can provide controlled release properties for encapsulated drugs Citation16.
Polymeric nanoparticles present a higher stability, when in contact with biological fluids and their polymeric nature allows obtaining the desired controlled and sustained release. The advantage that nanoparticles hold over other drug delivery systems is their submicron size which makes extravasations possible and occlusion of terminal blood vessel unlikely Citation17.
In last several years great efforts have been devoted to develop an oral delivery system for insulin. For example, Timmy and coworkers prepared β – cyclodextrin complexed insulin-loaded alginate microspheres Citation18 and examined the release profiles under varying conditions. A simple and versatile delivery platform for peptide and protein based on physically cross-linked poly (vinyl alcohol) (PVA) hydrogels containing insulin-loaded poly (lactic-co-glycolic acid) (PLGA) nanoparticles was successfully fabricated by Liu and coworkers Citation19. Ganorkar and coworkers prepared pH/thermo sensitive polymeric beads Citation20. Many natural polymers used for nanoparticle formulation include gelatin, chitosan, carboxy methyl cellulose and alginate. Huang and coworkers Citation21 employed low molecular weight chitosan nanoparticles as carriers for in vitro release of insulin and observed a ‘burst effect’ during the release of insulin. In order to inhibit the release of insulin at gastric pH, a derivative of chitosan, namely lauryl succinyl chitosan was used to develop nano and microparticles and in vitro release experiments of insulin were designed Citation22. Reis and coworkers Citation23 prepared dextran-alginate nanoparticles and studied in vitro release of insulin. The authors noted that the presence of dextran could suppress the release of insulin at gastric pH. In a novel approach, dextran nanoparticles were fabricated by Chalasani and coworkers Citation24 and conjugated to vitamin B12 molecules by surface modification of the nanoparticles. The prepared nanocarriers were examined for the release of insulin and various parameters were investigated.
In the present study, an attempt has been made to develop a swelling controlled carrier of insulin by preparing nanoparticles of alginate and loading them with insulin. Because of the anionic nature of alginate a lower release of insulin is expected in acidic medium and a larger release in alkaline. The present insulin delivery system is biocompatible, not-toxic, biodegradable and of low cost and works on a simple pH controlled swelling phenomenon Citation25,Citation26.
2. Results
2.1. FTIR spectral analysis
The structural characterisation of prepared insulin-loaded alginate nanocarriers has been done with the help of FTIR spectra. The spectra of native alginate and insulin-loaded alginate nanoparticles are shown in , respectively. The analysis of spectra indicates the presence of alginate and insulin in the loaded nanoparticles.
2.2. SEM analysis
The morphology of a drug carrier system is of immense significance as it greatly controls the extent of drug loading and subsequent kinetics of the drug release process. It is well known that when nanocarriers having voids, cracks, pores and unevenness on the surfaces come in contact with a drug solution during the loading process, greater number of drug molecules enter the nanoparticles in bulk via diffusion through the surface. A homogeneous surface with even morphology may offer lower drug entrapment while a heterogeneous normally results in a greater loading of the drug. Furthermore, shape and size of the nanoparticles also affect their stability in suspensions and eventually affect the dynamics of the drug release profiles. In the present investigation, the morphology of the nanoparticles was studied by recording the SEM image of the nanoparticles as shown in . It is clear form the SEM image that the surface of the nanoparticles appears even and suggests for its homogeneous nature. Moreover, the nanoparticles bear a regular and identical shape which implies for nearly varying dimensions of the nanoparticles.
2.3. Particle size analysis
The size of nanocarriers is an important parameter as it directly regulates the extent of loading as well as release dynamics of the drug. Moreover, the size of the particles determines the fate of the particles and its ultimate physiological impact. In this work, the particles size distribution curve obtained for the prepared particles is shown in which indicates that the size of the particles in not uniform and varies in the range about 40–150 nm. A broad range of particle size could be due to agglomeration of nanoparticles in the suspension.
2.4. Surface potential measurements
The charge carried by particles is of great importance as it decides the extent of drug–polymer interaction, release of the drug and stability of the carrier system under the existing conditions. In this study, the ξ potential of native alginate nanoparticles and insulin loaded are summarised in which suggests that after loading of the insulin, the positive charge over the alginate nanoparticles has increases.
Table 1. Surface potential of unloaded and loaded alginate nanoparticles.
2.5. Modelling and kinetic analysis of release mechanism
A hydrogel of any geometrical shape and size may be well imagined as a three-dimensional structure of macromolecular chains generating free volumes in between the chains. In this study also, the alginate nanoparticles contain voids or patches which are surrounded by the biopolymer chains and in these free spaces the insulin molecules are present as a loaded drug. When the drug-loaded devise is placed in the release medium, usually water or PBS, the water molecules penetrate into the loaded nanoparticles and allow the alginate chains to undergo frequent relaxation which consequently results in the diffusion of insulin molecules into the outer medium thus causing the beginning of the release process. The relative rates of chain relaxation and diffusion of drug molecules decide the fate of the release mechanism. It has been mathematically demonstrated, for instance Higuchi equation Citation27, that if n = 0.43 (for spherical devise like nanocarriers), the release is diffusion controlled (Fickian), when n = 0.84 the release becomes non Fickian (or case II) in nature and for n lying in the range 0.43–0.84, the drug release becomes anomalous. In same cases, however, the value of n has been found to exceed 0.84 and the mechanism is known as super case II Citation28. The values of n and d have been calculated from the Equations (Equation3) and (Equation4), respectively, as described in Section 3. The obtained data are summarised in .
Table 2. Data showing the release exponent and diffusion coefficient under varying experimental conditions.
2.6. Effect of percent loading on insulin release
There are many factors which profoundly influence the amount of the drug released in drug delivery process and one of such factors of prime significance is the percent loading which is often defined as the quantity of drug loaded onto the drug carrier. In this study, different extent of insulin was loaded onto the alginate nanoparticles by equilibrating them in drug solutions of known concentrations ranging form 11.7% to 38.9% (v/v). The drug-loaded swollen alginate nanoparticles were washed with distilled water to remove the excess drug, if any, and vacuum dried at room temperature for 1 week.
When the alginate nanoparticles loaded with different percent loading are left in the release medium, it is noticed that the amount of insulin released constantly increases with increasing percent loading as depicted in .
2.7. Effect of biopolymer on insulin release
The amount of drug delivered by nanocarriers is intimately related to chemical nature of the devise as well as the experimental protocol of the release experiments. There are several factors like the alginate and calcium chloride (crosslinker) contents of the nanoparticles which affect the overall size and surface properties of the particles. Moreover, the viscosity of the polymer solution (to prepare oil phase), agitation time and agitation speed are other prominent factors which alter the physical dimension of the nanoparticles and as a consequence influence the release profiles.
Figure 5. Effect of varying amount of alginate content in nanoparticles on release profiles of insulin for a definite composition of nanoparticles (alginate) = 1.0 g, (calcium chloride) = 0.5 mM, pH = 7.4, temperature = 37°C and % loading = 38.90%.
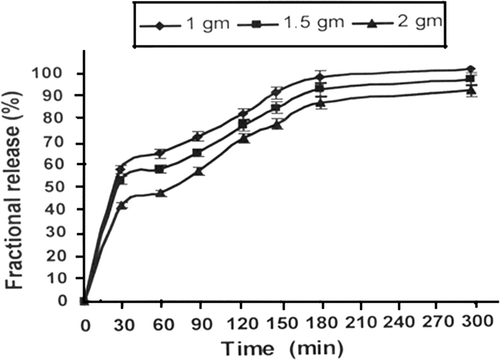
In order to observe the influence of alginate content in the nanoparticles on the released amount of insulin, the varying quantities of alginate were added into the feed mixture in the range 1.0–2.0 g and the nanoparticles were prepared. The resulting nanoparticles were loaded with insulin and the amount of released drug and swelling of nanoparticles were investigated. It is observed () that the amount of the insulin released decreases with increasing amount of the alginate. The water sorption capacity of the nanoparticles was also found to decrease with increasing amount of the biopolymer in the feed mixture of the nanoparticles as shown in the .
Table 3. Data showing the influence of composition and experimental condition on the equilibrium swelling ratio.
It is worth to mention here that with increasing quantity of alginate in the feed solution, a change in the size of nanoparticles is also expected. In this study also, the particle size distribution were obtained and it was noticed that the particle size distribution was insignificantly altered.
2.8. Effect of crosslinker
The crosslinking agent exerts a significant effect on the release patterns of the drug as a change in the number of crosslink in a network brings about a drastic change in the swelling properties of the polymer and consequently affects the release profiles of the drug. In this work, the concentration of calcium chloride was varied from 0.5 to 1.1 mM and the release results are presented in which reveals that the rate of insulin release decreases with increasing concentration of the crosslinker. The impact of increasing concentration of crosslinker on the water sorption capacity of the nanoparticles are also summarised in which also indicates a continuous fall in the swelling ratio of the alginate nanoparticles.
2.9. Effect of pH
A major problem normally encountered in the oral delivery of insulin like drugs is their enzymatic hydrolysis and digestion in the GI tract caused by the proteolytic digestive enzymes present in the harsh acidic environment of stomach. Furthermore, the poor bioavailability of insulin due to low permeation through the membranes puts another hurdle in the oral administration strategy. In this way, these two unfavourable consequences lead to poor therapeutic efficiency and need further efforts. In order to resolve these complexities some strategies have been suggested like use of pH change between the stomach and the small intestine within the GI tract Citation29 and exploitation of the bacterial enzymes localised within the colon Citation30.
The influence of pH on the water sorption capacity of the alginate nanoparticles and quantity of the released insulin has been examined by conducting swelling and release experiments at pH 1.2 and 7.4 which, respectively, represent the acidic and alkaline environments of stomach and blood. What actually happens is that upon oral administration of insulin it initially goes to acidic pH of stomach and then enters the alkaline pH range of intestine. These situations were simulated by first leaving the insulin-loaded alginate nanoparticles in acidic medium of pH 1.2 for 2 h and thereafter transferring them to a release medium of pH 7.4. The observed swelling and release results are shown in and , respectively.
2.10. Effect of simulated physiological fluids
In oral administration, the drug often comes in contact with biological fluids and it is, therefore, worth to see the effect of simulated biofluids on the water sorption capacity of the nanoparticles and the amount of drug released. To achieve the required results, the swelling and release experiments were conducted in different simulated biofluids and the obtained results are summarised in and , respectively. The results obtained form swelling and release experiments clearly shown that there is a significant fall in both the swelling ratio and released insulin in comparison to those obtained in the PBS.
Figure 8. Effect of physiological fluids on the released amount of insulin for a definite nanoparticles composition (alginate) = 1.0 g, (calcium chloride) = 0.5 mM, pH = 7.4 and % loading = 38.90%.
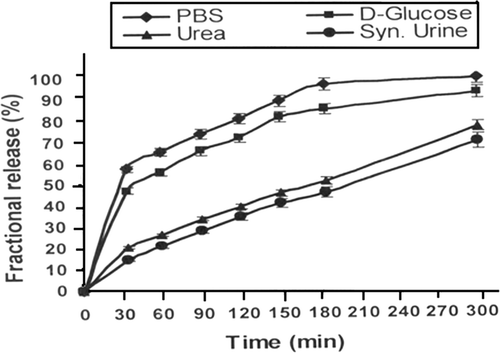
Table 4. Data showing the swelling ratio of the particle in various simulated physiological media.
2.11. Chemical stability of drug
If there is a change in chemical integrity of the released drug in acidic and alkaline environments, the delivered drug may not have comparable biological effect and the bioactivity of the insulin may be lost. Thus, it is worth to judge the chemical integrity of the drug in both the media. Although there are several methods to evaluate chemical integrity of the drug, in this study, however, a simple UV spectroscopic method has been adopted which involves recording the UV spectra of native and released insulin and comparing the λ max value and overall spectral pattern of the two drug solutions. For this purpose, UV spectra of insulin solution and insulin released at pH 1.2 and 7.4 was recorded and compared mutually.
2.12. Evaluation of biocompatibility
It is well recognised that all materials can not be considered as biomaterials until and unless they meet certain criterion and regulatory requirements. For instance, the material must be non-toxic, non-allergic, non-irritable and compatible with the tissues of the site where the implantation has to be placed in. Furthermore, the material implanted must be capable of establishing an adhesive contact with the living tissues. In this study, the biocompatibility of the prepared alginate nanocarriers has been judged by conducting two invitro tests such as protein adsorption and haemolysis assay as given below.
2.12.1. Bovine serum albumin adsorption
In order to evaluate the affinity of alginate nanoparticles for protein, namely bovine serum albumin (BSA), different nanoparticles of varying compositions were prepared and the amount of protein adsorbed was determined. The adsorbed amount of protein are summarised in which suggest that the quantity of protein adsorption increases with increasing content of alginate in the nanoparticles.
Table 5. Data showing the in vitro blood compatibility parameters for the gelatin nanoparticles.
2.12.2. Haemolysis test
When the alginate nanoparticles with varying biopolymer content are subjected to haemolytic activity experiments it is noticed that the extent of haemolysis increases with alginate content and the nanoparticles containing 1 g of alginate shown minimum haemolysis as depicted in .
2.13. Discussion
The FTIR spectra of (a) insulin-loaded and (b) unloaded alginate nanoparticles provide a direct evidence of the presence of functional groups of alginate and insulin in the drug-loaded nanocarriers. The spectral peak at 3618 cm−1 implies for the N–H stretching while the bands observed at 1681 cm−1confirm the −NH bending/carboxylate ion stretching and thus suggest for the presence of insulin in the drug-loaded nanoparticles Citation31. The spectral peaks also suggest for the presence of alginate as evident form the O–H stretching of hydroxyl at 3618 cm−1 and C(=O)2 stretching of carboxylate ion at 1591 cm−1, respectively Citation32.
The morphology of the alginate nanoparticles is depicted in and it clearly shows that the particles have identical dimensions and regular shapes. Whereas a good number of particles are seen to have a size as small as 20 nm, some particles do have a size greater then 40 nm.
The drug delivery through nanoparticles is a phenomenon that greatly depends on the size and size distribution of the nanoparticles employed as drug carrier. It is because of the fact that particle stability, biological fate, toxicity, extent of drug loading, membrane permeation potential, targeting ability and drug–tissue interaction of drug delivery system are mainly determined by dimension of the drug releasing particles. It is also well established that the nanoparticles having a size of a few nm are small enough to reach at the targeted cells, which is not possible with larger particles. In fact, it is the size of nanoparticles that eventually decides whether the particle may access the blood and other target sites such as liver, heart or blood cells. Since the smallest diameter of capillaries in the body is 4 µm, larger particles will be mainly captured and withheld in the lungs. Particles with larger size and aggregates of small particles may be trapped, causing emboli within the capillary bed of the lungs. In this way, smaller particles are always desired and in this study, the particle size distribution curve indicates that the size of the nanoparticles varies from 40 to 150 nm. A close examination of particle size distribution curve shows that about 90% particles have a particle size near 100 nm which is quite small to carry the insulin molecules in the circulatory system. This obviously indicates for the fair suitability of the prepared alginate nanoparticles for delivery of the drug. It is important to mention here that the results of SEM and particle size analysis are not much consistent with each other and this could be due to aggregation of particles in suspension state.
The surface potential measurements are quite helpful in ascertaining drug polymer interactions in drug delivery devises. In the present investigation, the drug used is insulin which is a positively charged molecule and, therefore, its binding to the alginate nanoparticles results in a change in the potential of the nanoparticles. The results reveal that at pH 1.2, 7.4 and 8.6 the positive potential carried by the particles increases from 262 to 297, 56 to 84 and −88 to −21.2 mV, respectively. The reason for the observed increase in positive potential is due to the binding of insulin molecules to the surfaces of the alginate particles. Another favourable point is that due to the potential of the drug-loaded nanoparticles, the repulsion will be operative and it will impart stability to the suspensions. This also provides an experimental evidence of loading of the drug to the alginate nanoparticles.
The percentage loading results reveal that when the loading is 11.7%, about 18% insulin is released in 30 min while 40% and 60% drug is released when loading increased to 25.5% and 38.9%, respectively. In the later case of higher loading, the abrupt release of insulin in first half an hour may be because of the ‘burst effect’ which is a commonly reported phenomenon in drug delivery through nanoparticles. The release profiles also reveal that beyond 30 min the release rate increases significantly. The reason for the observed increase is that when the extent of insulin loading is greater, the mobility of release medium front into the nanoparticles surface is faster and, therefore, greater amount of insulin is released. Another explanation may be that due to higher loading of positively charged insulin molecules the relaxation of macromolecular chains become rapid and release of insulin increases as reported by other workers also Citation33. It is interesting to see here that at lowest loading of 11.7%, an abrupt increase in insulin release is noticed from 30% to 80% after 150 min which may be attributed to the reason that due to lower loading, the drug–polymer interaction will be weaker and after the nanoparticles swells adequately, the release of insulin will be faster.
The increasing amount of alginate in the nanoparticles causes a fall in the swelling of nanoparticles which brings about a decrease in the release of insulin. It is clear form the release profiles that when the alginate content increases form 1.0 to 2.0 g, the release of insulin drops from 60% to 40% in half an hour. The release profiles also indicate that as the amount of alginate increases, the burst effect becomes more prominent. The observed results may be attributed to the reason that an increase in the amount of alginate enhances the interaction between the drug and alginate chains and these results in a lower water sorption and drug release. An alternate explanation may be that with increase in alginate content in the nanoparticles, the volume fraction of biopolymer increases and, therefore, both the water and insulin molecules have to adopt a lengthy path within the nanoparticles network for undergoing swelling and release processes. This clearly results in a lower release of insulin Citation34. It is noticeable here that for all the three composition of the nanoparticles, a ‘burst effect’ still prevails which is due to the fact that due to high content of biopolymer in the nanocarriers the loaded insulin may still move up to the nanoparticles surfaces through capillary action and results in the said effect.
In this study, the crosslinker employed was calcium chloride and it was observed that its increasing concentration tends to lower the swelling ratio as well as the insulin release. It is clear from the release profiles that when the concentration of crosslinker increases from 0.5 to 1.1 mM, the percent release decreases from 60% to about 20%. The results also reveal that when the crosslinker concentration is greatest, i.e. 1.1 mM, almost no burst effect is seen. Another remarkable point is that up to 3 h, almost constant delivery of insulin is observed with implies for a zero-order release pattern. The obtained decrease is quite expected as on increasing the amount of crosslinker the number of crosslink are enhanced which eventually reduce the mesh size of the nanoparticle network. Thus, the reduced free volumes in the alginate nanoparticle network hinder the inclusion of water molecules into the biopolymer network from the release medium and consequently the water sorption decreases. At the same time, the expulsion of insulin molecules from the nanoparticle network also become difficult and the amount of insulin release decreases. The observed decrease in the amount of released insulin is reported elsewhere also Citation35.
The results reveal that at pH 1.2, about 20% insulin is released while at pH 7.4 the released insulin goes to about 90%. What actually happens is that at low gastric pH the alginate forms a compact acid–gel structure which not only restricts the expulsion of insulin into the release medium but also provides chemical stability to the drug by protecting it from the harsh environment. Another reason may be that in highly acidic pH, the alginate biomacromolecules will be in undissociated form as the pK a of alginate lies between 3.4 to 4.4 and this will lead to a reduced water sorption and insulin release. However, at pH 7.4, which is well above the pK a of alginate, the alginate macromolecules will be present as dissociated carboxylate ions. Now, since the biopolymer chains are negatively charged, they will exert repulsion force on one another and gives rise to a frequent relaxation of biopolymer molecules of the nanoparticles. Thus, due to the enhanced relaxation of chains, more and more water molecules will enter the nanoparticles network and swelling will increase. At the same time, the molecules of insulin will be expelled into the release medium and the percent release will increase Citation36. The release profiles show that up to 2 h only 20% of the entrapped insulin is released at stomach pH while beyond 2 h as high as 90% of insulin is released in the intestinal pH of 7.4 which are pharmaceutically desired release quantifications for any oral delivery formulation. The observed results reveal that by properly manipulating the composition of the nanoparticles, the release of insulin in acidic medium could be further reduced from 20% to a much lower value.
When the swelling and release experiments are performed in simulated biofluids, a decrease is observed as compared to those in the PBS. The observed lower water sorption and drug release in these physiological fluids is attributable to the fact that the salt ions are present in the external medium and thus cause a decrease in the osmotic pressure of the system. This results in a reduced swelling of loaded nanospheres, which consequently lowers the insulin release. As may also be noted from the release profiles that a negligible ‘burst effect’ is seen when the release media is urea and synthetic urine while significant ‘burst effect’ appears in PBS and D-glucose media. The observed findings are due to the reason that in urea and synthetic urine solution the nanoparticles network remains compact and does not allow insulin to move along the capillaries and produce ‘burst effect’.
The chemical integrity of the insulin was experimented by leaving the drug-loaded alginate nanoparticles at pH 1.2 for 2 h and then the insulin was allowed to release at pH 7.4. The UV spectra of insulin released at pH 7.4 were compared with that of the insulin present in native solution at pH 7.4. The UV spectra obtained for insulin at two different conditions are shown in , respectively, and presents a fair resemblance in their shape which suggests that even after being present in acidic medium the chemical nature of the drug does not change. The observed stability of insulin in harsh acidic environment is due to the fact that the pK a of alginate molecules lies well above 3.4 and, therefore, in highly acidic medium alginate changes into alginic acid and do not allow acidic environment to affect chemical or biological activity of the drug. It was also noticed that the gastric juice medium does not cause any breakdown of the alginate nanoparticles and thus implies for a fair stability of the nanocarriers system which make them a suitable candidate for oral formulation of insulin.
Figure 9. UV spectra of (a) native insulin and (b) released insulin showing the evidence of chemical integrity of the drug.
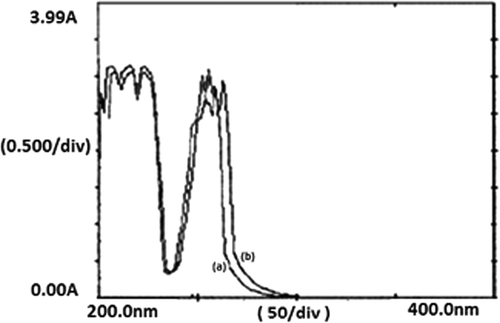
The results indicate that when the amount of alginate increases form 1.0 to 2.0 g, the protein adsorption marginally increases form 3.3 to 4.8 mg g−1. The increasing protein adsorption implies for enhanced thrombogenicity or reduced blood compatibility. The reason for the observed lower blood compatibility with greater alginate content may be due to increase in the amount of alginate, the charged groups present along the biomacromolecules will also increase and this will lead to a greater interaction between the blood components and charged centres of the alginate. This will result in lower antithrombogenic nature of the nanoparticles surfaces.
It is also clear from the haemolysis data that when the amount of alginate increases from 1.0 to 2.0 g, the percent haemolysis increases from 3.4 to 11.3. The observed findings are consistent with the protein adsorption results and suggest that the nanoparticles with greater amount of alginate may possess less blood compatibility. Thus, in order to design biocompatible nanoparticles the amount of alginate must be small.
The kinetic release data summarised in reveal that when the amount of alginate increases from 1.0 to 2.0 g, the value of n varies from 0.20 to 0.40 which show a Fickian type of release process. In a similar way, the kinetic parameters were also calculated for nanoparticle produced with varying concentrations of calcium chloride as shown in . It is clear form the table that when the concentration of crosslinking bath increases from 0.03 to 0.09 M, the value of n varies form 0.43 to 0.20. The data imply that at the lowest concentration of the crosslinker the release is diffusion controlled or Fickian in nature while with increasing concentration of calcium chloride (crosslinking bath), the value of n is quite less than 0.43 and suggests for nearly diffusion controlled nature of the release process. In fact, it is known that when the release exponent has lower values than those predicted from the Ficks equations, this could be due to either more water solubility of the drug or diffusion controlled nature of the releasing drug. In this case, also the values of n are lower than 0.42 and may be accounted for the diffusion controlled nature of the release process which belong to Fickian type of transport mechanism.
It is also clear form that the value of n is 0.15 and 0.20 at pH 1.2 and 7.4, respectively, which suggest for diffusion controlled release nature of the insulin at these pH media.
3. Experimental
3.1. Materials and method
Alginate was obtained from Research Lab, Pune, India, and calcium chloride (Loba Chemie) was used as a crosslinking agent. The oil phase was prepared with polymethyl methacrylate (Sigma Aldrich Co., Average Mw ∼ 3.5 × 105). The drug chosen for the study was insulin (Activity 40 IU mL−1) purchased from Torrent Pharmaceuticals Ltd. The simulated gastric juice of pH 2.0 was prepared with 0.01 mol L−1 HCl, 2.5 g sodium laurel sulphate and 2.0 g NaCl.
3.2. Preparation of nanoparticles
In this study, emulsion crosslinking method was followed for the preparation of nanoparticles as described here in brief.
The method basically involves preparing alginate and crosslinker (calcium chloride) emulsions (w/o) and mixing them intimately to crosslink alginate by calcium chloride. In order to prepare an aqueous phase, 1.0 g of alginate was dissolved in 20 mL of distilled water while the ‘oil phase’ was prepared by dissolving 3.5 g of polymethyl methacrylate into 30 mL of a mixture of chloroform (1 : 1, v/v) toluene. The emulsion was prepared by vigorous shaking (shaking speed 300 RPM, 0.5 HP motor capacity, Toshniwal, India) of aqueous and oil phases together for 30 min. Now, a 5 mL of calcium chloride emulsion was prepared by mixing 5 mL of aqueous solution of calcium chloride with 5 mL of oil phase. Now, the emulsions of alginate and calcium chloride were mixed with constant shaking. The crosslinking reaction was allowed to take place for 3 h at room temperature. After the crosslinking reaction is over, nanoparticles of alginate were cleaned by centrifuging and then resuspending five times in toluene and twice in acetone. The isolated nanoparticles were dried at room temperature under vacuum and stored in airtight polyethylene bags.
3.3. Characterisation of alginate nanoparticles
The prepared alginate nanoparticles were characterised by the following analytical techniques – the first one is physicochemical characterisation, and the other one is biopharmaceutical characterisation.
3.4. FTIR spectra
The FTIR spectra of insulin-loaded alginate nanoparticles and native alginate were recorded on a FTIR spectrophotometer (8400 S, Shimadzu spectrophotometer). The sample for recording FTIR spectra was prepared by mixing KBr pellets with the nanoparticles (1 : 10, w/w) followed by uniaxillary pressing the powder under vacuum. The spectra were obtained between 4000 and 400 cm−1 at 2 cm−1 resolution.
3.5. Scanning electron microscopy
The scanning electron microscopy images of alginate nanoparticles were recorded on a SEM machine (Philips 515). Prior to analysis, the sample of nanoparticles was coated with gold using ion sputtering and during the SEM study, the accelerating voltage was kept at 20 kV.
3.6. Particle size analysis
The particle size analysis of alginate nanoparticles was determined by dynamic light scattering using a particle size analyser (Malvern Mastersizer, 2000). The nanoparticles sample was freeze-dried and its suspension was prepared in an aqueous buffer solution. The incidence light scattered at 90° was measured and the obtained data were analysed in automatic mode.
3.7. Surface potential measurements
The charges carried by the insulin and alginate nanoparticles were measured by a digital potentiometer (Model No. 118, EI Product, Mumbai, India). The method involves preparing a dispersion of drug-loaded nanoparticles in 20 mL of respective pH solutions and measuring the potential of the suspension using a compound electrode system consisting of Pt and calomel electrodes. In a similar way, the charge carried by the native alginate nanoparticles was measured.
3.8. Swelling measurements
In this study, the water sorption capacity of nanoparticles was quantified by a conventional gravimetric method which includes immersing a known amount of drug-loaded nanoparticles in the PBS solution, separating them by centrifugation, and weighing it again after definite time period when the equilibrium swelling has reached. Prior to weighing the swollen nanoparticles, they were gently pressed in between the filter papers to remove excess of water. The weight–swelling ratio was calculated by
3.9. Loading of insulin on to the nanoparticles
In the most commonly adopted method of loading, the devise is allowed to swell in the drug till equilibrium. In this study also, this method has been used for loading of insulin onto the alginate nanoparticles.
In a typical experiment, 100 mg of native alginate nanoparticles (W 0) were allowed to swell in the insulin solution of known concentration and state of equilibrium was achieved. The swollen nanoparticles were washed, dried and finally weighed (W d). The following relation was used to calculate the percent loading,
It is important to mention here that during the drying of the drug-loaded device the drug molecules often move towards the surface due to capillary action through the polymer chains and give rise to the ‘burst effect’. In this study also, the ‘burst effect’ has been observed as will be discussed later on.
3.10. In-vitro release experiments
The release experiments were performed by allowing the insulin-loaded alginate nanoparticles of known weight (50 mg) to swell in a known volume of PBS (pH 7.4, 1.2 mM KH2PO4, 1.15 mM Na2HPO4, 2.7 mM KCl and 1.38 mM NaCl). The suspension of insulin-loaded alginate nanoparticles was mildly agitated to speed up the release process (Remi Shakers, India, 100 rpm). After half an hour of shaking, 5 mL of supernatant was withdrawn and replaced by 5 mL fresh PBS. The amount of released insulin was estimated spectrophotometrically by reading the absorbance of the solution at 271 nm (UV–Vis Spectrophotometer, Shimandzu 1700 Phama Spec).
3.11. Kinetics of release process
In order to monitor the progress of the insulin release process, a definite volume of release medium was withdrawn at predetermined time intervals and instantly replaced by the same volume of fresh release medium. The amount of released insulin was quantified in the aliquots taken following the spectrophotometer method as mentioned previously. The following equations were used to look into the mechanism of the release process Citation37 which enables one to calculate the release exponent ‘n’ and diffusion coefficient of the releasing drug from the knowledge of the fractional release of insulin (Wt /W ∞) at time ‘t’,
With the help of the above equations, the values of n and D may be calculated and a prediction of the release mechanism of the drug may be made.
3.12. Blood compatibility
The following test procedures Citation38,Citation39 were adopted to judge the invitro blood compatibility of the prepared alginate nanoparticles.
3.12.1. BSA adsorption
Adsorption of BSA onto the nanoparticles was performed by the batch process. For adsorption experiments, protein (BSA) solutions were made in 0.5 M PBS at physiological pH 7.4. A fresh solution of BSA was always prepared for every experiment. Prior to adsorption experiments, the particles were equilibrated with PBS for 24 h and then the adsorption was carried out by gently shaking a BSA solution of known concentration containing preweighed and fully swollen particles. By taking fully swollen particles, the possibility of soaking the BSA solution within the particle is minimised. Shaking was performed so gently that no froth was produced; otherwise it would have formed an air–water interface. After a definite time period, the particles were removed and the protein adsorption was assayed for the remaining concentration of BSA by recording the absorbance of protein solution at 272 nm on a UV–Vis Spectrophotometer (Shimandzu 1700 Phama Spec). The adsorbed amount of BSA was calculated by following the weight balance equation,
3.12.2. Haemolysis assay
Haemolysis assay experiments were performed on the surfaces of the prepared particles. In a typical experiment, known weights of particles were equilibrated in normal saline water (0.9% NaCl solution) for 24 h at 37°C, and human ACD blood (0.25 mL) was added up to the particles suspension. After 20 min, 2.0 mL of 0.9% sodium chloride (saline) was added to each sample to stop haemolysis and the samples were incubated for 60 min at 37°C. Positive and negative control were obtained by adding 0.25 mL of human ACD blood and 0.9% NaCl solution, respectively, and centrifuged for 45 min. The supernatants were taken and its absorbance was recorded at 545 nm. The percentage of haemolysis was calculated using the following relationship,
3.13. Chemical stability of drug
Since the insulin-loaded nanoparticles have to reside in the harsh environment (pH 1.2) of gastric juice, there may be possibility of loss of bioactivity of entrapped insulin which needs to be ascertained. Thus, in order to examine the chemical stability of insulin the loaded nanoparticles were left in simulated gastric juice (pH 1.2) for 2 h and then the loaded nanoparticles were taken out, mildly washed and then transferred to release medium (pH 7.4). The released fraction of insulin was scanned between 200 and 400 nm and the obtained UV spectra were compared with that of the native insulin in the same pH of 7.4.
3.14. Statistical analysis
In order to test the reliability of the experimental data, all experiments were done at least thrice and the curves drawn were shown along with the respective error bars.
4. Conclusions
In this work, alginate nanoparticles were produced by emulsion crosslinking technique and release profiles of insulin were investigated under different experimental conditions. The insulin-loaded alginate nanoparticles when examined with FTIR and SEM techniques reveal that the nanoparticles contain characteristic functional groups of alginate and insulin, and depict heterogeneous morphology with particle size varying in the range 40–150 nm.
The loading of nanoparticles by insulin molecules enhances positive charge over the nanoparticles surfaces as evident from surface potential measurements. This also provides an evidence for loading of insulin onto the alginate molecules. The insulin-loaded nanocarriers demonstrate fair stability even in harsh gastric environment and preserve the chemical nature of insulin due to encapsulation within the alginate matrix.
The extent of water uptake varies with pH and temperature and shows an optimum sorption at pH 7.4 and 37°C. The alginate nanoparticles swell to a lower extent when allowed to swell in simulated physiological fluids.
The release of insulin is a function of the percent loading of the drug and found to increase with increase in percent loading of the drug. When the loading of insulin is 11.7% then 18% insulin get released in half an hour while at 25.5% and 38.9% loading, the insulin releases up to 40% and 60%, respectively. It is also noticed that at higher loading of insulin a ‘burst effect’ is seen which almost disappears at low-percent loading of the drug. The fractional release also decreases with increasing alginate content and the ‘burst effect’ is also observed with both low and high alginate contents. In first 30 min about 60% insulin gets released when the alginate content is 1.0 g while for nanoparticles with 2.0 g of alginate, the released amount of insulin drops to just 40%. The insulin release is also suppressed when higher concentration of crosslinker is employed and it is worth to mention here that at higher concentration of the crosslinking agent no ‘burst effect’ is obtained.
The fractional release of insulin is quite sensitive to pH of the release medium. It is observed that pH 1.8 (simulated gastric pH) less then 20% insulin is released while at higher pH (intestinal pH) up to 90% release is obtained. It is also observed that at low pH no ‘burst effect’ is seen. The fractional release of insulin also decreases in simulated physiological fluids and it is found that in urea and synthetic urine media no ‘burst effect’ is present.
The nature of the release process of insulin when analysed in light of the Ficks power law is found to show diffusion-controlled mechanism at all the studied concentration range of alginate, calcium chloride (crosslinking agent) and pH.
The invitro blood compatibility of nanoparticles was also judged and it was found that, as the amount of alginate and crosslinker increases in the feed composition of the nanoparticles, both the protein adsorption and percent haemolysis increase which suggest for reduced in vitro blood compatibility.
Thus, the experimental results suggest that alginate-based formulation with highly crosslinked network containing greater amount of alginate do not show any ‘burst effect’ and release much less amount of the entrapped insulin at gastric pH. Moreover, the nanoparticles also ensure retention of chemical activity of the insulin and preserve the macrodrug well from the harsh acidic environment. Conclusively, the formulation shows potential to act as nanocarrier for oral delivery of insulin at least under in vitro conditions.
References
- Mishra , M , Kumar , H , Singh , RK and Tripathi , K . 2008 . Diabetes and nanomaterials . J. Nanomater. Biostruct. , 3 : 109 – 113 .
- Finucane , P and Popplewell , P . 2001 . Diabetes Mellitus and Impaired Glucose Regulation in Old Age: The Scale of the Problem, in Diabetes in Old Age , 2nd , New Jersey, USA : John Wiley and Sons .
- Kumar , MT and Misra , A . 2006 . Formulation and evaluation of insulin dry powder for inhalation . Drug Dev. Ind. Pharm. , 32 : 677 – 686 . doi: 10.1080/03639040600712862
- Park , EJ , Werner , J and Smith , NB . 2007 . Ultrasound mediated transdermal insulin delivery in pigs using a lightweight traducer . Pharm. Res. , 24 : 1396 – 1401 . doi: 10.1007/s11095-007-9306-4
- Davidson , MB , Mehta , AE and Siraj , ES . 2006 . Inhald human insulin: An inspiration for patients with diabetic mellitus . Cleve. Clin. J. Med. , 73 : 569 – 578 . doi: 10.3949/ccjm.73.6.569
- Torres-Lugo , M , Garcia , M , Record , R and Peppas , NA . 2002 . Physiological behavior and cytotoxic effects of P (MAA-g-EG) nanospheres for oral delivery of proteins . J. Control. Release , 80 : 97 – 205 . doi: 10.1016/S0168-3659(02)00027-5
- Heinemann , L , Pfutzner , A and Heise , T . 2001 . Alternative routes of administration as an approach to improve insulin therapy: Update on dermal, oral, nasal and pulmonary insulin delivery . Curr. Pharm. Des. , 7 : 1327 – 1351 . doi: 10.2174/1381612013397384
- Arbit , E . 2004 . The physiological rationale for oral insulin administration . Diab. Technol. Ther. , 6 : 510 – 517 . doi: 10.1089/1520915041705929
- Mishra , M , Kumar , H , Singh , RK and Tripathi , K . 2008 . Diabetic delayed wound healing and the role of silver nanoparticles . J. Nanomater. Biostruct. , 3 : 49 – 54 .
- Soppimath , KS , Aminnabhavi , TM , Dave , AM , Kumbar , SG and Rudzinki , WE . 2002 . Stimulus responsive smart hydrogels as novel drug delivery systems . Drug Dev. Ind. Pharm. , 28 : 957 – 974 . doi: 10.1081/DDC-120006428
- Leserman , L . 2004 . Liposomes as protein carriers in immunology . J. Liposome Res. , 14 : 175 – 189 . doi: 10.1081/LPR-200039198
- Liu , H , Tang , R , Pan , WS , Zhang , Y and Liu , H . 2003 . Potential utility of various protease inhibitors for improving the intestinal absorption of insulin in rats . J. Pharm. Pharmacol. , 55 : 1523 – 1529 . doi: 10.1211/0022357022052
- Cui , F , Tao , A , Cun , D , Zhang , L and Shi , K . 2007 . Preparation of insulin loaded PLGA-Hp 55 nanoparticles for oral delivery . J. Pharm. Sci. , 96 : 421 – 427 . doi: 10.1002/jps.20750
- Lowe , PJ and Temple , CS . 1994 . Calcitonin and insulin in isobutylcyanoacrylate nanocapsules: Protection against pro-teases and effect on intestinal absorption in rats . J. Pharm. Pharmacol. , 46 : 547 – 552 . doi: 10.1111/j.2042-7158.1994.tb03854.x
- Janes , KA , Calvo , P and Alonso , MJ . 2001 . Polysaccharide colloidal particles as delivery systems for macromolecules . Adv. Drug Rev. , 47 : 57 – 83 .
- Galindo Rodriguez , SA , Allemann , E , Fessi , H and Doelker , E . 2005 . Polymeric nanoparticles for oral delivery of drugs and vaccines: A critical evaluation of in vivo studies . Crit. Rev. Ther. Drug Carrier Syst. , 22 : 419 – 464 . doi: 10.1615/CritRevTherDrugCarrierSyst.v22.i5.10
- Barrat , G . 2003 . Colloidal drug carriers: Achievements and perspectives . Cell Mol. Life Sci. , 60 : 21 – 37 . doi: 10.1007/s000180300002
- Timmy , SA , Victor , SP , Sharma , CP and Kumari , V . 2002 . Betacyclodextrin complexed insulin loaded alginate microspheres-oral delivery system . J. Trends Biomater. Artif. Organs , 15 : 48 – 53 .
- Liu , J , Zhang , SM , Chen , PP , Cheng , L , Zhou , W , Tang , WX , Chen , ZW and Ke , CM . 2008 . Controlled release of insulin from PLGA nanoparticles embedded within PVA hydrogels . J. Mater. Sci. Mater. Med. , 18 : 2205 – 2210 . doi: 10.1007/s10856-007-3010-0
- Ganorkar , CR . 1999 . Modulating insulin release profile from pH/thermosensitive polymeric beads through polymer molecular weight . J. Control. Release , 59 : 287 – 298 . doi: 10.1016/S0168-3659(99)00006-1
- Huana , X , Du , Y-Z , Yuan , H and Hu , F-O . 2009 . Preparation and pharmacodynamics of low-molecular-weight chitosan nanoparticles containing insulin . Carbohydr. Polym. , 76 : 368 – 373 . doi: 10.1016/j.carbpol.2008.10.025
- Rekha , M and Sharma , CP . 2009 . Synthesis and evaluation of lauryl succinyl chitosan particles towards oral insulin delivery and absorption . J. Control. Release , 135 : 144 – 151 . doi: 10.1016/j.jconrel.2009.01.011
- Reis , CP , Ribeiro , AJ , Houng , S , Veiga , P and Neufeld , RJ . 2007 . Nanoparticulate delivery systems for insulin: Design, characterization and in vitro/in vivo bioactivity . Eur. J. Pharmaceut. Sci. , 30 : 392 – 397 . doi: 10.1016/j.ejps.2006.12.007
- Chalasani , KB , Russell-Jones , GJ , Yandrapu , SK , Diwan , PV and Jain , SK . 2007 . A novel vitamin B12-nanosphere conjugate carrier system for peroral delivery of insulin . J. Control. Release , 117 : 421 – 429 . doi: 10.1016/j.jconrel.2006.12.003
- Dong , Z . 2006 . Alginate/gelatin blend films and their properties for drug controlled release . J. Membrane Sci. , 280 : 37 – 44 . doi: 10.1016/j.memsci.2006.01.002
- Gomez , WR and Wee , SF . 1998 . Protein release from alginate matrices . Adv. Drug Deliv. Rev. , 31 : 267 – 285 . doi: 10.1016/S0169-409X(97)00124-5
- Higuchi , T . 1961 . Rate of release of medicaments from ointments bases containing drugs in suspension . Pharmaceut. Sci. , 56 : 874 – 875 . doi: 10.1002/jps.2600501018
- Siepmann , J and Peppas , NA . 2001 . Modeling of drug release from delivery systems based on hydroxypropyl methyl cellulose . Adv. Drug Deliv. Rev. , 48 : 139 – 157 . doi: 10.1016/S0169-409X(01)00112-0
- Wood , MK , Stone , GM and Peppas , NA . 2008 . Wheat germ agglutinin functionalized complexation hydrogels for oral insulin delivery . Biomacromolecules , 9 : 1263 – 1298 . doi: 10.1021/bm701274p
- Lancaster , CM and Wheatly , MA . 1989 . Drug delivery to the colon polymer susceptibility to degradation by colon contents . Polym. Prepr. , 30 : 480 – 481 . doi: 10.1016/0032-3861(89)90018-9
- Jain , D , Majumdar , DK and Panda , AK . 2006 . Insulin loaded Eudragit L100 microspheres for oral delivery . Biomaterials , 21 : 195 – 211 .
- Roy , A , Bajpai , J and Bajpai , AK . 2009 . Dynamics of controlled release of chloropyrifos from swelling and eroding biopolymeric microspheres of calcium alginate and starch . Carbhydr. Polym. , 76 : 222 – 231 . doi: 10.1016/j.carbpol.2008.10.013
- Bajpai , AK and Bhanu , S . 2003 . Mobility in hydrogels based on poly (acrylic acid) and macro-(IPN) . J. Macromol. Sci. Part A Pure Appl. Chem. , 40 : 265 – 292 . doi: 10.1081/MA-120018114
- Bajpai , AK and Mishra , A . 2005 . Preparation and characterization of tetracycline-loaded interpenetrating polymer networks of carboxymethyl cellulose and poly (acrylic acid): Water sorption and drug release study . Polym. Int. , 54 : 1347 – 1356 . doi: 10.1002/pi.1839
- Bajpai , AK and Bhanu , S . 2004 . In vitro release dynamics of insulin from a loaded hydrophilic polymeric network . J. Mater. Sci. Mater. Med. , 15 : 43 – 54 . doi: 10.1023/B:JMSM.0000010096.48608.5b
- Foss , AC and Goto , T . 2004 . Development of acrylic based copolymers for oral insulin delivery . Pharmaceut. Biopharmaceut. , 57 : 163 – 169 . doi: 10.1016/S0939-6411(03)00145-0
- Bajpai , AK and Saini , R . 2005 . Preparation and characterization of spongy cryogels of poly (vinyl alcohol)-casein system: Water sorption and blood compatibility study . Polym. Int. , 54 : 796 – 806 . doi: 10.1002/pi.1773
- Bajpai , AK . 2007 . Blood protein adsorption onto macroporous semi-interpenetrating polymer networks (IPNs) of poly (ethylene glycol) (PEG) and poly (2-hydroxyethyl methacrylate) (PHEMA) and assessment of in vitro blood compatibility . Polym. Int. , 56 : 231 – 244 . doi: 10.1002/pi.2137
- Bundela , H and Bajpai , AK . 2008 . Designing of hydroxyapatite-gelatin based porous matrix as bone substitute: Correlation with biocompatibility aspects . Express Polym. Lett. , 2 : 201 – 213 . doi: 10.3144/expresspolymlett.2008.25