Abstract
Nitrogen-doped multi-walled carbon nanotubes (N-MWCNT) were selectively grown on oxidised silicon substrate by means of catalytic chemical vapour deposition with decomposition of acetonitrile in the presence of ferrocene (FeCp2) at 900°C. The synthesised N-MWCNT film was initially treated with continuous ozone flow for various time periods and characterised by means of scanning electron microscopy and Raman spectroscopy. The electrochemical response of ozone-treated N-MWCNT film towards ferrocyanide/ferricyanide, [Fe(CN)6]3−/4− redox couple was investigated by means of cyclic voltammetry (CV) and electrochemical impedance spectroscopy. The results were compared with those obtained for untreated N-MWCNT film. The findings reveal the occurrence of structural defects on surface of ozone-treated N-MWCNT film due to oxidation of nanotubes. Furthermore, the handling of N-MWCNT film with ozone increases the barrier for interfacial electron transfer and slows down the kinetics of redox reaction occurring on this particular electrode.
1. Introduction
The carbon nanotubes (CNTs) are allotropes of carbon with cylindrical nanostructure that were recently discovered and attracted special attention due to their unique electronic and mechanical properties as well as to their resistance to acid/basic media Citation1–3. CNT can be synthesised by means of several techniques based on either chemical Citation4 or plasma Citation5 processes and applied as materials for the development of biological and chemical gas sensors Citation6,Citation7, supercapacitors Citation8, hydrogen storages Citation9–11, solar cells Citation12, drug deliveries Citation13 and electron field emitters Citation14. Furthermore, from CNT various carbon-based nano-electronic and magneto-electronic devices (e.g. one-dimensional GaN nanorods) can be produced Citation15–18. The modification of CNT leads to essential changes in their electronic structure as well as their functional properties and leads to the creation of novel materials with incredible interesting properties Citation19,Citation20. One of the simplest approaches in modification of CNT involves their oxidation with various oxidising agents and the functionalisation of the active oxygen-containing groups that are formed on their tube walls. The oxidation of CNT has gained a lot of attention for many scientists the last years since it enhances the chemical reactivity of the graphitic network. Specifically, it was found that through a treatment of pristine CNT with an oxidising agent, oxygen-containing groups are formed on graphitic surface, which facilitate the exfoliation of CNT bundles and increase their solubility in polar media Citation21,Citation22. It is well known that diluted HCl solutions remove the residues of the metal catalyst from CNT, but they do not affect considerably their structure Citation23. However, strong oxidising agents, such as HNO3, cause defects on the structure of CNT leading, consequently, to the formation of oxygen-containing groups, such as hydroxyl, carbonyl and carboxyl groups, on their tube walls via covalent functionalisation Citation24. The oxidised CNT can be later modified at their functional groups through various methods that allow organic molecules to be directly attached to the nanotube sidewalls. Depending on application for which the modified CNT are intended, methods such as cyclo-addition, electrophilic, nucleophilic or radical addition, as well as various polymerisation techniques, can be used for the functionalisation of CNT Citation25. The gas treatment for the oxidation of CNT is a simple and effective method that does not require the use of solvents and was already adopted in numerous works dealing with CNT chemistry Citation26. The handling with ozone was preferred from many scientists since it is one of the strongest known oxidants and although ozone itself is toxic, ozone treatment leaves no toxic residues that have to be treated or disposed Citation27. The reaction between ozone and CNT was already investigated theoretically Citation28 and experimentally Citation29. A main feature of ozonolysis is the preferential oxidation of CNT by ozone attack and the release of CO2. For multi-walled carbon nanotubes (MWCNT), the oxidation tends to start near the tips permitting the cutting of the tips and the opening of the tubes. The oxidation proceeds layer by layer, resulting in thinner tubes Citation30.
In this work, nitrogen-doped MWCNT (N-MWCNT) were grown on oxidised silicon substrate by using the chemical vapour deposition (CVD) technique, treated with continuous ozone flow for different time periods (60, 120 and 180 s), and characterised by means of scanning electron microscopy (SEM) and Raman spectroscopy. Furthermore, the electrochemical response of untreated and ozone-treated N-MWCNT film towards [Fe(CN)6]3−/4− was probed by means of CV and EIS techniques. The results are discussed in terms of structural changes of N-MWCNT occurring upon oxidation.
2. Experimental
2.1. Reagents and solutions
Potassium hexacyanoferrate(III), K3Fe(CN)6 (∼99.0%), potassium hexacyanoferrate(II) trihydrate, K4Fe(CN)6 · 3H2O (>98.5%) and potassium chloride, KCl (>99.0%) were purchased from Sigma-Aldrich and used as received without any further purification. The measured solutions consisted of K3Fe(CN)6/K4Fe(CN)6 mixture (1.0 × 10−3 mol L−1) were prepared by dissolving the appropriate amounts of the electro-active compounds in aqueous solution of KCl (0.1 mol L−1). The solutions were prepared immediately prior the electrochemistry experiments by using double-distilled water having specific conductivity 0.50 µS cm−1.
2.2. Apparatus and procedures
The scanning electron micrographs of N-MWCNT were obtained on FEI/Philips (model XL30 ESEM) computer-controlled SEM with an accelerating voltage of 20 kV and magnification factors in the range of ×500–×6000. The cyclic voltammograms (CVs) were recorded using a computer-controlled system Zahner (model IM6/6EX). The effect of the uncompensated resistance was reduced by applying the positive feedback technique. The electrochemistry measurements were carried out using a three-electrode cell configuration. The working electrode was either treated or ozone-untreated N-MWCNT (geometric area 1.0 cm2) film, the counter electrode was Pt plate (geometric area 2.0 cm2) and the reference electrode was Ag/AgCl (sat. KCl). For all the electrochemistry experiments, a three-compartment electrochemical cell specially designed to minimise the distances between the electrodes with a total solution volume of 20 mL, was used. Before each measurement process, the solution was purged with high purity argon in order to eliminate interference from oxygen. The CVs were recorded in the potential range from −0.2 to +0.8 V (versus Ag/AgCl) with scan rates ranging from 0.05 to 0.10 V s−1. All measurements were carried out at room temperature (20°C). The electrochemical impedance spectra (EIS) were recorded with a computer-controlled system Zahner (model IM6/6EX) by applying very small amplitude of the alternate voltage (10 mV) in the frequency range from 0.1 Hz to 100 kHz at the room temperature (20°C). All electrochemistry experiments were performed on either untreated or ozone-treated N-MWCNT (geometric area 1.0 cm2) film against the reference electrode Ag/AgCl (sat. KCl), while Pt plate (geometric area 2.0 cm2) was served as counter electrode. The EIS spectra were recorded at the half-wave potential of the investigated redox couple [Fe(CN)6]3−/4− (E 1/2 ≈ +0.21 versus Ag/AgCl). The recorded EIS spectra were analysed by means of the equivalent electrical circuit (R s + (C dl/(R ct + Z w))) Citation31,Citation32 (software Thales, version 4.15), which includes a resistance in series with a parallel combination of a capacitive path and charge- and mass-transfer paths. The elements of the electrical circuit are clarified as follows: the resistors R s and R ct represent the electrolyte and the charge transfer resistances, respectively, the capacitor C dl represents the double-layer capacitance and Z W is the Warburg impedance element Citation33. The mean and maximum modified impedance simulation errors were estimated in all cases to be less than 0.4% and 7%, respectively. The Raman scattering measurements were carried out using a Jasco RFT-6000 FT-Raman attachment coupled with an FT/IR-6300 spectrometer at an excitation wavelength of 1064 nm (excitation energy of 1.17 eV). The Raman spectra were recorded directly from either untreated or ozone-treated N-MWCNT film with a laser power of 50–200 mW and spectral resolution of 4 cm−1 in the backscattering geometry.
2.3. Fabrication and treatment of N-MWCNT
Vertically aligned MWCNT having diameter in the range of 30–150 nm were selectively synthesised on oxidised porous silicon wafer with geometrical area of 1.0 cm2 (Siegert Consulting e.K.) by means of CVD technique using acetonitrile (ACN) as carbon source and FeCp2 as catalyst. The used silicon wafer having a thickness of about 525 µm was fully covered with a SiO2-coating of about 1.7 µm thickness. The ACN solution including FeCp2 (1% w/w) was introduced to the furnace at 900°C through a syringe with a flow rate of 0.2 mL min−1. The synthesis process was performed using argon as a carrier gas. The average thickness of the synthesised N-MWCNT film was estimated to be about ∼27 µm. Elemental composition analysis of synthesised MWCNT performed by means of X-ray photoelectron spectroscopy reveal the existence of significant amount of incorporated nitrogen in nanotubes (7.0%). The MWCNT-based working electrode used for the electrochemistry experiments was prepared as follows: the produced N-MWCNT film was initially connected to platinum wire by using silver conducting coating and afterwards, the silver layer was fully covered with varnish protective coating. The scheme of CVD apparatus and additional details concerning the synthesis and characterisation of N-MWCNT were already reported in previous articles Citation34–36. Ozone was generated by an electric discharge in flow of pure oxygen (>99.99%) using an ozone-generator (Model 502, Fischer technology, Bonn, Germany) set at a flow rate of 50 L h−1. The ozone treatment time lasted from 60 to 180 s.
3. Results and discussion
Representative SEM micrographs taken for untreated and ozone-treated N-MWCNT film are shown in . In order to identify better the morphology of nanotubes, different level of magnifications was used form taking the SEM images. As was previously reported in literature, the nanotubes doped with nitrogen are bamboo-like shaped and perfectly ordered Citation37. The SEM images confirm that the nanotubes of untreated N-MWCNT are well ordered and separated with each other leading to well-organised film surface with insignificant faults (). The ozone-treated N-MWCNT film consists of closely packed and highly entangled CNT bundles. The surface of this particular film contains ‘holes’ having diameters in the range 5–40 µm (, ). In order to verify the micro-physical analysis from the SEM micrographs, a quantification of the defects was made by using Raman spectroscopy. Representative Raman spectra of untreated and ozone-treated N-MWCNT are presented in . The Raman shifts along with the Raman bands intensity ratios are listed in . As it can be seen in , the Raman spectra of N-MWCNT film consist of two main bands, namely the so-called D-band (∼1290 cm−1) and the G-band (∼1597 cm−1) Citation38, and their frequency is unaffected by ozone treatment. The G-band corresponds to the high frequency E2g first-order mode and can be attributed to the opposing movement of two-neighbouring carbon atoms in graphene sheet Citation39, while the D-band results from defects in curved graphene sheets, as well as from ends and finite size crystalline domains of CNTs Citation40. It is well known that the ratio of relative intensities of G- and D-bands (I
G/I
D) is a measure of the degree of structural order. For MWCNT with different surface modifications, the change in (I
G/I
D) intensity ratio can be attributed to the change in surface functionalities or local defects resulting from bond breakage between C–C bonds in nanotubes Citation41. In this work, the ozone treated N-MWCNT films were found to exhibit smaller value of intensity ratio (I
G/I
D) compared to the untreated film, indicating that the structural defects prevail in ozone handled films Citation42. The findings suggest that a certain amount of structural disorder in ozone-treated N-MWCNT was generated by the continuous decoration of functional groups on the sidewalls of the nanotubes. The covalent functional groups that were introduced to the sidewalls of the nanotubes are assumed to cause difficulties to the tubes to retain their perfect cylindrical shape Citation43. The destruction of the tubular morphology of nanotubes with the ozone treatment can probably explain the ‘holes’ with various geometric shapes and sizes that were observed on surfaces of the ozone-treated N-MWCNT films (). The (I
G/I
D) ratio progressively decreases with the ozonolysis time indicating an enhancement of the degree of defects with ozonolysis (). It is interesting that after a treatment for about 180 s insignificant variation of the (I
G/I
D) ratio occurs. Typical CVs obtained with the scan rate of 0.05 V s−1 showing the effect of ozonolysis time on electrochemical performance of N-MWCNT film are displayed in . The CVs were recorded in the presence of [Fe(CN)6]3−/4− (1.0 × 10−3 mol L−1) redox couple in KCl solution (0.1 mol L−1). The extracted CV parameters are presented in . A couple of well-defined symmetric redox peaks with equal cathodic () and anodic (
) peak currents (
/
≈ 1) were observed in CVs recorded on either untreated or ozone-treated N-MWCNT films. The findings exhibit that the amounts of oxidised and reduced forms of electro-active compound are equal and not consumed in coupled chemical reaction, confirming, that the charge-transfer process occurring on studied N-MWCNT films can be considered as Nernstian. Besides, the determined value of charge transfer coefficient of a = 0.5, which is a typical value for reversible reactions, supports further the last conclusion Citation44,Citation45. The half-wave potential (E
1/2) of [Fe(CN)6]3−/4− redox system was found to be independent of ozonolysis time. Nevertheless, an influence of ozone handling time of N-MWCNT film on anodic and cathodic peak potential separation ΔE
p (ΔE
p = E
p
ox − E
p
red) was observed, namely, an increase of ΔE
p with the ozone-handling time of N-MWCNT film occurs. Considering that ΔE
p is related to heterogeneous charge transfer rate constant (k
s) of redox reaction, the findings indicate an important falling of kinetics of [Fe(CN)6]3−/4− redox couple with ozonolysis. Specifically, the k
s values of [Fe(CN)6]3−/4−, determined by means of electrochemical absolute rate relation Citation46, reveal that an ozone treatment of N-MWCNT film for only 60 s leads to a decrease of more than 80% in kinetics of the redox reaction occurring on this particular electrode (). It is also remarkable that further ozonolysis slightly affects the kinetics of redox reaction. The specific surface area of the untreated N-MWCNT film determined as 1.71 cm2 increases sharply to 4.18 cm2 after an ozonolysis treatment for about 60 s, while further ozone handling causes a trivial improvement of the surface area, namely an increase of about 4% was observed for an additional ozone treatment for about 60 s (). In general, it was observed that the current density on ozone-treated N-MWCNT film is about 7% greater of that of the untreated film. The results suggest that the treatment of N-MWCNT film with ozone increases its specific area upon cutting off (opening) the nanotube tips by the oxidation process Citation47. Complementary information concerning the electrochemical response of either the untreated or ozone-treated N-MWCNT films towards [Fe(CN)6]3−/4− was obtained from EIS measurements. Representative EIS spectra illustrating the effect of ozonolysis on impedance performance of N-MWCNT film are shown in . In the EIS spectra the complex impedance is presented as a sum of the real (Z
re) and imaginary (Z
imag) components that originate mainly from the resistance and capacitance of the cell, respectively. The EIS spectra were analysed by means of the equivalent electrical circuit (R
s + (C
dl/(R
ct + Z
w))). The determined EIS parameters are included in . As it can be seen in , the EIS spectra include a semicircle portion that corresponds to the electron-transfer-limited process, and a linear part that is attributed to diffusion-limited electron transfer. The semicircle diameter, obtained by extrapolation of the semicircle on Z
re-axis, corresponds to the electron transfer resistance (R
ct), a parameter that is attributed to electrochemical reaction occurring on electrode's surface. As it can be observed in EIS spectra displayed in , the handling with ozone leads to an increase of the semicircle diameter, indicating that the electro transfer process occurring on ozone-treated N-MWCNT, is quite limited. It was observed that the R
ct values of the [Fe(CN)6]3−/4− redox system on N-MWCNT film tend to increase with the ozonolysis time. Specifically, the findings are in absolute accordance with the CV results and demonstrate that the barrier for interfacial electron transfer increases with the ozone handling time.
Figure 1. SEM micrographs taken for untreated (a) and ozone-treated N-MWCNT films (b, c, d). The SEM images were obtained with an accelerating voltage of 20 kV and magnification factors of 1600× (a), 500 × (b), 2000× (c) and 6000× (d).
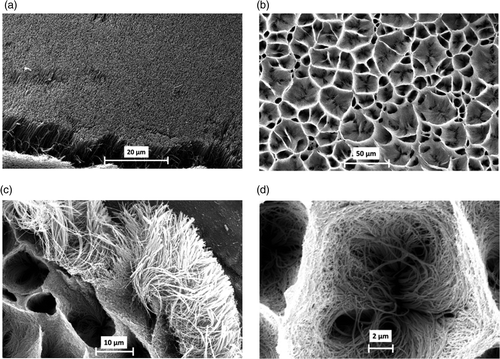
Figure 2. Raman spectra recorded for either untreated (a) or ozone-treated N-MWCNT film for 60 s (b) and 180 s (c). The symbols D and G represent the Raman D- and G-bands, respectively.
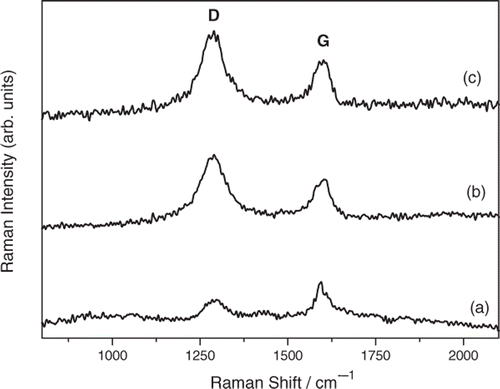
Figure 3. Variation of the intensity ratio of G- and D-bands (I G/I D) with the ozonolysis time of N-MWCNT film.
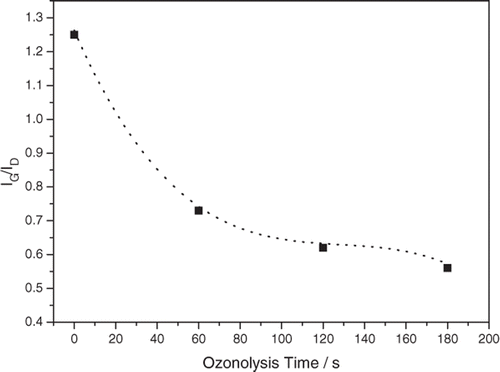
Figure 4. Representative CVs displaying the effect of ozonolysis time on electrochemical performance of N-MWCNT film. The CVs were recorded at v = 0.05 V s−1 in the presence of [Fe(CN)6]3−/4− (1.0 × 10−3 mol L−1) in KCl (0.1 mol L−1) on either untreated (dotted line) or ozone-treated N-MWCNT film for 60 s (dashed line), 120 s (dotted-dashed line) and 180 s (solid line).
![Figure 4. Representative CVs displaying the effect of ozonolysis time on electrochemical performance of N-MWCNT film. The CVs were recorded at v = 0.05 V s−1 in the presence of [Fe(CN)6]3−/4− (1.0 × 10−3 mol L−1) in KCl (0.1 mol L−1) on either untreated (dotted line) or ozone-treated N-MWCNT film for 60 s (dashed line), 120 s (dotted-dashed line) and 180 s (solid line).](/cms/asset/7b477ad5-3222-41c2-a85f-c8216d1d08af/tjen_a_664792_o_f0004g.gif)
Figure 5. Representative EIS spectra illustrating the effect of ozonolysis time on impedance behaviour of N-MWCNT film. The EIS spectra were recorded in the presence of [Fe(CN)6]3−/4− (1.0 × 10−3 mol L−1) in KCl (0.1 mol L−1) either on untreated (filled square) or ozone-treated N-MWCNT film for 60 s (filled circle), 120 s (filled upward triangle) and 180 s (filled downward triangle). For better readability, the solution ohmic resistance (∼10 Ω) was subtracted from the real part of the impedance data. The EIS spectra were recorded at the half-wave potential of [Fe(CN)6]3−/4− (E 1/2 ≈ +0.21 V versus Ag/AgCl).
![Figure 5. Representative EIS spectra illustrating the effect of ozonolysis time on impedance behaviour of N-MWCNT film. The EIS spectra were recorded in the presence of [Fe(CN)6]3−/4− (1.0 × 10−3 mol L−1) in KCl (0.1 mol L−1) either on untreated (filled square) or ozone-treated N-MWCNT film for 60 s (filled circle), 120 s (filled upward triangle) and 180 s (filled downward triangle). For better readability, the solution ohmic resistance (∼10 Ω) was subtracted from the real part of the impedance data. The EIS spectra were recorded at the half-wave potential of [Fe(CN)6]3−/4− (E 1/2 ≈ +0.21 V versus Ag/AgCl).](/cms/asset/878f3025-f298-4a45-9151-75f26b810e20/tjen_a_664792_o_f0005g.gif)
Table 1. Raman D-bands, G-bands and intensity ratios of G- and D-bands (I G/I D) for the untreated and ozone-treated N-MWCNT for different time periods.
Table 2. Anodic (E
p
ox) and cathodic (E
p
red) peak potentials,a peak potential separations (ΔE
p), anodic peak current densities (
), cathodic and anodic peak current ratios (
/
), half-wave potentials (E
1/2),a active surface areas (A), heterogeneous electron transfer rate constants (k
s),b charge transfer coefficients (a),c double layer capacitances (C
dl),d charge transfer resistances (R
ct)d and Warburg parameters (σ)de for [Fe(CN)6]3−/4− (1.0 × 10−3 mol L−1) on untreated and ozone-treated N-MWCNT film for various time periods.
It is very interesting that analogous findings were obtained on ozone treated pristine MWCNT. Namely, a film consists of pristine MWCNT was produced with decomposition of benzene by means of CVD Citation34–36 and the effect of the ozone treatment on its electrochemical response towards [Fe(CN)6]3−/4− was investigated. The electrochemical behaviour of [Fe(CN)6]3−/4− on ozone treated pristine MWCNTs was comparable to that observed on N-MWCNTs. Specifically, an increase of peak potential separation of about 150% and decrease of heterogeneous rate constant of about 85% was observed on pristine MWCNTs after an ozone treatment for about 180 s. The findings reveal that the handling with a very powerful oxidising agent such as ozone causes nearly the same structure effects on both nanotube materials (pristine and N-MWCNT).
4. Conclusions
A novel N-MWCNT/oxidised silicon film was synthesised by means of CVD with decay of ACN in the presence of FeCp2 at 900°C. The N-MWCNT film was treated with ozone and applied as working electrode for the electrochemical analysis of [Fe(CN)6]3−/4− redox couple. The findings reveal the occurrence of structural defects on surface of ozone-treated N-MWCNT film. The defective surface is probably resulted from the C–C breakage and the formation of carboxyl and hydroxide groups on sidewalls of the nanotubes. The findings demonstrate that the ozone treatment leads to significant increase of the film's active surface and enhances the current density. Moreover, the treatment of N-MWCNT film with ozone increases the barrier for interfacial electron transfer and slows down the kinetics of redox reaction occurring on this particular electrode. It is remarkable that an ozonolysis for about 60 s leads to decrease for about 80% of the kinetics of investigated redox system.
Acknowledgments
The authors would like to thank Mrs. D. Schneider and Mrs. Risch (TU Ilmenau).
References
- Chen , S , She , W , Wu , G , Chen , D and Jiang , M . 2005 . A new approach to the functionalization of single-walled carbon nanotubes with both alkyl and carboxyl groups . Chem. Phys. Lett. , 402 : 312 – 317 . doi: 10.1016/j.cplett.2004.12.035
- Tersoff , J and Ruoff , RS . 1994 . Structural properties of a carbon-nanotube crystal . Phys. Rev. Lett. , 73 : 676 – 679 . doi: 10.1103/PhysRevLett.73.676
- Serp , P , Corrias , M and Kalck , P . 2003 . Carbon nanotubes and nanofibers in catalysis . Appl. Catal., A , 253 : 337 – 358 . doi: 10.1016/S0926-860X(03)00549-0
- Nasibulin , AG , Moisala , A , Jiang , H and Kauppinen , EI . 2006 . Carbon nanotube synthesis from alcohols by a novel aerosol method . J. Nanopart. Res. , 8 : 465 – 475 . doi: 10.1007/s11051-005-9027-8
- Zajíčková , L , Jašek1 , O , Eliáš , M , Synek , P , Lazar , L , Schneeweiss , O and Hanzlíková , R . 2010 . Synthesis of carbon nanotubes by plasma enhanced chemical vapor deposition in an atmospheric-pressure microwave torch . Pure Appl. Chem. , 82 : 1259 – 1272 . doi: 10.1351/PAC-CON-09-09-38
- Fleischer , M and Meixner , H . 1998 . Selectivity in high-temperature operated semiconductor gas-sensors . Sens. Actuators B , 52 : 179 – 187 . doi: 10.1016/S0925-4005(98)00271-8
- Kong , J , Franklin , NR , Zhou , C , Chapline , MG , Peng , S , Cho , K and Dai , H . 2000 . Nanotube molecular wires as chemical sensors . Science , 287 : 622 – 625 . doi: 10.1126/science.287.5453.622
- Stoller , MD , Park , S , Zhu , Y , An , J and Ruoff , RS . 2008 . Graphene-based ultracapacitors . Nano Lett. , 8 : 3498 – 3502 . doi: 10.1021/n1802558y
- Gao , H , Wu , XB , Li , JT , Wu , GT , Lin , JY , Wu , K and Xu , DS . 2003 . Hydrogen adsorption of open-tipped insufficiently graphitized multi-walled carbon nanotubes . Appl. Phys. Lett. , 83 : 3389 – 3391 . doi: 10.1063/1.1620675
- Liu , C , Fan , YY , Liu , M , Cong , HT , Cheng , HM and Dresselhaus , MS . 1999 . Hydrogen storage in single-walled carbon nanotubes at room temperature . Science , 286 : 1127 – 1129 . doi: 10.1126/science.286.5442.1127
- Chen , P , Wu , X , Lin , J and Tan , KL . 1999 . High H2 uptake by alkali-doped carbon nanotubes under ambient pressure and moderate temperatures . Science , 285 : 91 – 93 . doi: 10.1126/science.285.5424.91
- Wei , J , Jia , Y , Shu , Q , Gu , Z , Wang , K , Zhuang , D , Zhang , G , Wang , Z , Luo , J , Cao , A and Wu , D . 2007 . Double-walled carbon nanotube solar cells . Nano Lett. , 7 : 2317 – 2321 . doi: 10.1021/nl070961c
- Liu , Z , Chen , K , Davis , C , Sherlock , S , Cao , Q , Chen , X and Dai , H . 2008 . Drug delivery with carbon nanotubes for in vivo cancer treatment . Cancer Res. , 68 : 6652 – 6660 . doi: 10.1158/0008-5472.CAN-08-1468
- de Heer , WA , Bonard , JM , Fauth , K , Châtelain , A , Forró , L and Ugarte , D . 1997 . Electron field emitters based on carbon nanotube films . Adv. Mater. , 9 : 87 – 89 . doi: 10.1002/adma.19970090122
- Zhang , Y , Tan , YW , Stormer , HL and Kim , P . 2005 . Experimental observation of the quantum Hall effect and Berry's phase in grapheme . Nature , 438 : 201 – 204 . doi: 10.1038/nature04235
- Saito , S . 1997 . Carbon nanotubes for next-generation electronics devices . Science , 278 : 77 – 78 . doi: 10.1126/science.278.5335.77
- Chiang , WH and Sankaran , RM . 2009 . Linking catalyst composition to chirality distributions of as-grown single-walled carbon nanotubes by tuning NixFe1 − x nanoparticles . Nature Mater. , 8 : 882 – 886 . doi: 10.1038/nmat2531
- Han , W , Fan , S , Li , Q and Hu , Y . 1997 . Synthesis of gallium nitride nanorods through a carbon nanotube-confined reaction . Science , 277 : 1287 – 1289 . doi: 10.1126/science.277.5330.1287
- Hamada , N , Sawada , S and Oshiyama , A . 1992 . New one-dimensional conductors: Graphitic microtubules . Phys. Rev. Lett. , 68 : 1579 – 1581 . doi: 10.1103/PhysRevLett.68.1579
- Jouguelet , E , Mathis , C and Petit , P . 2000 . Controlling the electronic properties of single-wall carbon nanotubes by chemical doping . Chem. Phys. Lett. , 318 : 561 – 564 . doi: 10.1016/S0009-2614(00)00089-0
- Chen , J , Hamon , MA , Hu , H , Chen , Y , Rao , AM , Eklund , PC and Haddon , RC . 1998 . Solution properties of single-walled carbon nanotubes . Science , 282 : 95 – 98 . doi: 10.1126/science.282.5386.95
- Liu , J , Rinzler , AG , Dai , H , Hafner , JH , Bradley , RK , Boul , PJ , Lu , A , Iverson , T , Shelimov , K , Huffman , CB , Rodriguez-Macias , F , Shon , YS , Lee , TR , Colbert , DT and Smalley , RE . 1998 . Fullerene pipes . Science , 280 : 1253 – 1256 . doi: 10.1126/science.280.5367.1253
- Cravotto , G , Garella , D , Gaudino , EC , Turci , F , Bertarione , S , Agostini , G , Cesano , F and Scarano , D . 2011 . Rapid purification/oxidation of multi-walled carbon nanotubes under 300 kHz-ultrasound and microwave irradiation . New J. Chem. , 35 : 915 – 919 . doi: 10.1039/c0nj00892c
- Zhang , J , Zou , HL , Qing , Q , Yang , YL , Li , QW , Liu , ZF , Guo , XY and Du , ZL . 2003 . Effect of chemical oxidation on the structure of single-walled carbon nanotubes . J. Phys. Chem. B , 107 : 3712 – 3718 . doi: 10.1021/jp027500u
- Huang , WJ , Taylor , S , Fu , KF , Lin , Y , Zhang , DH , Hanks , TW , Rao , AM and Sun , YP . 2002 . Attaching proteins to carbon nanotubes via diimide-activated amidation . Nano Lett. , 2 : 311 – 314 . doi: 10.1021/nl010095i
- Tsang , SC , Harris , PJF and Green , MLH . 1993 . Thinning and opening of carbon nanotubes by oxidation using carbon dioxide . Nature , 362 : 520 – 522 . doi: 10.1038/362520a0
- Simmons , JM , Nichols , BM , Baker , SE , Marcus , MS , Castellini , OM , Lee , CS , Hamers , RJ and Eriksson , MA . 2006 . Effect of ozone oxidation on single-walled carbon nanotubes . J. Phys. Chem. B , 110 : 7113 – 7118 . doi: 10.1021/jp0548422
- Picozzi , S , Santucci , S , Lozzi , L , Valentini , L and Delley , B . 2004 . Ozone adsorption on carbon nanotubes: The role of Stone-Wales defects . J. Chem. Phys. , 120 : 7147 – 7152 . doi: 10.1063/1.1669381
- Byl , O , Liu , J and Yates , JT . 2005 . Etching of carbon nanotubes by ozone-a surface area study . Langmuir , 21 : 4200 – 4204 . doi: 10.1021/la040132w
- Cataldo , F . 2008 . A study on the action of ozone on multiwall carbon nanotubes . Fullerenes Nanotubes Carbon Nanostruct. , 16 : 1 – 17 . doi: 10.1080/15363830701779281
- Vetter , KJ . 1967 . Electrochemical Kinetics , New York : Academic Press .
- Omeiri , S , Bellal , B , Bouguelia , A , Bessekhouad , Y and Trari , M . 2009 . Electrochemical and photoelectrochemical characterization of CuFeO2 single crystal . J. Solid State Electrochem. , 13 : 1395 – 1401 . doi: 10.1007/s10008-008-0703-3
- Koeleli , F , Roepke , T and Hamann , CH . 2003 . Electrochemical impedance spectroscopic investigation of CO2 reduction on polyaniline in methanol . Electrochim. Acta , 48 : 1595 – 1601 . doi: 10.1016/S0013-4686(03)00076-8
- Szroeder , P , Tsierkezos , NG , Scharff , P and Ritter , U . 2010 . Electrocatalytic properties of carbon nanotube carpets grown on Si-wafers . Carbon , 48 : 4489 – 4496 . doi: 10.1016/j.carbon.2010.08.009
- Tsierkezos , NG and Ritter , U . 2010 . Synthesis and electrochemistry of multi-walled carbon nanotube films directly attached on silica substrate . J. Solid State Electrochem. , 14 : 1101 – 1107 . doi: 10.1007/s10008-009-0924-0
- Tsierkezos , NG , Szroeder , P and Ritter , U . 2011 . Multi-walled carbon nanotubes as electrode materials for electrochemical studies of organometallic compounds in organic solvent media . Monatsh. Chem. , 142 : 233 – 242 . doi: 10.1007/s00706-011-0454-1
- Jang , JW , Lee , CE , Lyu , SC , Lee , TJ and Lee , CJ . 2004 . Structural study of nitrogen-doping effects in bamboo-shaped multi-walled carbon nanotubes . Appl. Phys. Lett. , 84 : 2877 – 2879 . doi: 10.1063/1.1697624
- Ritter , U , Scharff , P , Siegmund , C , Dmytrenko , OP , Kulish , NP , Prylutskyy , YuI , Belyi , NM , Gubanov , VA , Komarova , LA , Lizunova , SV , Poroshin , VG , Shlapatskaya , VV and Bernas , H . 2006 . Radiation damage to multi-walled carbon nanotubes and their Raman vibrational modes . Carbon , 44 : 2694 – 2700 . doi: 10.1016/j.carbon.2006.04.010
- Nemanish , RJ and Solin , SA . 1979 . First- and second-order Raman scattering from finite-size crystals of graphite . Phys. Rev. B , 20 : 392 – 401 . doi: 10.1103/PhysRevB.20.392
- Choi , S , Park , KH , Lee , S and Koh , KH . 2002 . Raman spectra of nano-structured carbon films synthesized using ammonia-containing feed gas . J. Appl. Phys. , 92 : 4007 – 4011 . doi: 10.1063/1.1499233
- Tian , ZQ , Jiang , SP , Liang , YM and Shen , PK . 2005 . Synthesis and characterization of platinum catalysts on multi-walled carbon nanotubes by intermittent microwave irradiation for fuel cell applications . J. Phys. Chem. B , 110 : 5343 – 5350 . doi: 10.1021/jp056401o
- Sulong , AB , Azhari , CH , Zulkifli , R , Othman , MR and Park , J . 2009 . A Comparison of defects produced on oxidation of carbon nanotubes by acid and UV ozone treatment . Eur. J. Sci. Res. , 33 : 295 – 304 .
- Lee , SH , Jung , YC , Kim , YA , Muramatsu , H , Teshima , K , Oishi , S and Endo , M . 2009 . Optical spectroscopic studies of photochemically oxidized single-walled carbon nanotubes . Nanotechnology , 20 : 105708 – 105712 . doi: 10.1088/0957-4484/20/10/105708
- Maeda , Y , Sato , K , Ramaraj , R , Rao , TN , Tryk , DA and Fujishima , A . 1999 . The electrochemical response of highly boron-doped conductive diamond electrodes to Ce3+ ions in aqueous solution . Electrochim. Acta , 44 : 3441 – 3449 . doi: 10.1016/S0013-4686(99)00109-7
- Sun , Z , Hu , J , Lu , Y and Li , Q . 2003 . Studies on the electrochemical behavior of cytochrome c and its interaction with DNA at a Co/GC ion implantation modified electrode . Analyst , 128 : 930 – 934 . doi: 10.1039/b212292h
- Nicholson , RS . 1965 . Theory and application of cyclic voltammetry for measurement of electrode reaction kinetics . Anal. Chem. , 37 : 1351 – 1355 . doi: 10.1021/ac60230a016
- Ye , JS , Liu , X , Cui , HF , Zhang , WD , Sheu , FS and Lim , TM . 2005 . Electrochemical oxidation of multi-walled carbon nanotubes and its application to electrochemical double layer capacitors . Electrochem. Commun. , 7 : 249 – 255 . doi: 10.1016/j.elecom.2005.01.008