Abstract
TbMnO3 rods-like morphology on nanoscale were prepared by chemical co-precipitation route and their magnetic properties were studied. We were able to synthesise nanorods measuring around 100 nm in average size. SEM image confirmed the morphology and average size of TbMnO3. Orthorhombic phase was indexed from XRD data. The formation of metal-O bond in the TbMnO3 was confirmed by FT-IR. The temperature dependence of the zero-field cooled (ZFC) and the field-cooled (FC) magnetisation for different magnetising field showed well-defined feature around 8 K corresponding to the antiferromagnetic ordering of Tb3+ moments. The irreversibility in magnetisation curves suggests that the TbMnO3 nanorods exhibit cluster glass behaviour, rather than spin glass behaviour. A slight magnetic hysteresis observed at 4 K, and the magnetisation is almost saturated at high field, which is due to a weak ferromagnetism of Mn arrays.
1. Introduction
Research into multiferroic materials continues at an intense rate, motivated by fundamental questions of structure–function relationships in strongly correlated systems. Efforts have been devoted to find new multiferroic materials or to investigating multiferroic properties in known oxides, such as BiCrO3, BiMnO3, BiFeO3, BiCoO3 and BiScO3 Citation1–6. Recently, multiferroic effects have been found in some rare earth manganites. Perovskite rare-earth manganites RMnO3 (R = Gd, Tb, Dy, Ho, Er, Tm, Yb, Lu, Y, etc.) are representative examples of multiferroic materials. The orthorhombic family of rare earth manganites, RMnO3, has been investigated extensively since the discovery of multiferroic behaviour in TbMnO3, with DyMnO3 and GdMnO3 also exhibiting similar behaviour Citation7,Citation8. Materials with perovskite-related structure, like manganites, possess a great variety of physical properties important for new technological developments. Their potential applications in information storage, spintronics, sensors and new novel devices demand an intensive investigation. Properties such as the multiferroic behaviour, in which ferro-electricity and magnetic order occur on the same phase, have been recently revealed in the perovskite compound TbMnO3. Such manganites present a distorted perovskite structure, which is closely related to the magnetic properties. It is well-known that the properties of functional materials depend highly on their morphologies, dimensions, sizes and defects. Especially at the nanoscale, the materials are expected to display enhanced properties, or a completely new set of properties which are usually absent in their bulk forms. Therefore, it is important and interesting to study the nanosized systems of multiferroic materials. For the famous multiferroic system TbMnO3, much work has been devoted to the two-dimensional nanofilms epitaxially grown on a range of substrates including SrTiO3, LaAlO3 and NdGaO3 Citation9–11. However, to date, there has been little work concerned with the synthesis of substrate-free nanostructures of TbMnO3 Citation12. Han et al. reported the synthesis of matchbox-like TbMnO3 particles via a convenient hydrothermal route, which, however, have a size generally in the order of 10 µm Citation13. In this present work, we report on the synthesis of TbMnO3 nanorods by chemical co-precipitation route and its magnetic properties showing cluster glass behaviour of TbMnO3 nanorods.
2. Experiments
For preparation of TbMnO3 nanorods, stoichiometric amounts of TbCl3·6H2O (99.9%, Aldrich) and MnCl2·4H2O (AR, Fishers), in double distilled water were mixed and stirred for 2 h. This solution of mixed salts was then drop-wise added to 0.05 M solution of KOH (GR, Merck) under vigorous magnetic stirring. After 17 h of magnetic stirring at room temperature, the colloidal solution was concentrated using vacuum evaporation. Precipitation was achieved by the addition of acetone to the concentrate and removing the supernatant. After drying at room temperature, the obtained powder sample was ground into a fine powder in an agate mortar. This sample was calcined at 800°C for 90 min, yielding TbMnO3 black colour powder. The phase composition of this powder sample was detected by X-ray powder diffraction (D8 Advanced Bruker instrument with Cu Kα radiation (1.54 Å) at a scanning rate of 1° min−1. The formation of metal-O bond in the TbMnO3 powder sample was confirmed by 3000 Hyperion Microscope with Vertex 80 FT-IR System. The morphology of the sample was observed using JSM-7600 F Field emission gun-scanning electron microscope (FEG-SEM). Temperature profile of the magnetisation of the samples was measured by Vibrating Sample Magnetometer, CRYOGENIC Inc., UK.
3. Results and discussion
shows the X-ray powder diffraction pattern of TbMnO3 powder calcined at 800°C. All the diffraction peaks are well-matched with the (JCPDS 72-0379) orthorhombic structure of TbMnO3 (space group: pbnm) with unit cell constants a = 5.3778 Å, b = 5.8933 Å, c = 7.3989 Å. The average crystallite size calculated using scherrer formula for these particles is found to be about 90 ± 10 nm. shows FT-IR spectra of TbMnO3 powder calcined at 800°C. Several absorption peaks between 600 and 400 cm−1, being characteristic for the Mn–O stretching, Tb–O stretching and O–Mn–O bending vibrations of TbMnO3 perovskite structure Citation12, are observed for the powder sample calcined at 800°C. The morphology of the powder sample was examined by SEM, as shown in . SEM image exhibits a rod-like morphology of TbMnO3 particles with average size 100 nm. We depict the formation mechanism of TbMnO3 nanorods in two steps: nucleation and growth. At the initial step of the reaction between TbCl3 and KOH, Tb(OH)3 precipitates. Now as excess KOH is added to the solution, the precipitated Tb(OH)3 dissolves and forms a homogeneous aqueous solution that contains enough ions. Next, the reaction between
and MnCl2 gives the growth unit of TbMnO3 and directly incorporated into TbMnO3 crystallites. These nuclei grow further to produce rod-like TbMnO3.
The temperature dependence of the zero-field cooled (ZFC) and the field-cooled (FC) magnetisation of TbMnO3 nanorods at different magnetising field and the irreversibility temperature in TbMnO3 nanorods at different magnetising field is shown in and , respectively. The anomaly in the magnetisation in TbMnO3 nanorods is observed near ∼8 K for both FC and ZFC curves, corresponding to the antiferromagnetic ordering of Tb3+ moments Citation14. The small anomaly around 27 K is observed in TbMnO3 nanorods for both ZFC and FC curves and is attributed to the sine wave ordering of Mn3+ moments and lock-in transition, respectively don’t appear to be strong in the rods-like morphology Citation7. The transition around 41 K (absent in this report) generally observed in single crystalline TbMnO3 and is attributed to the sine wave ordering of Mn3+ moments Citation7,Citation14. There is a possibility that the sine wave ordering of Mn3+ is localised due to nanodimensions of the rods. The rods-like morphology develop intrinsic strain gradient, which is responsible for softening of incommensurate Lock-in transition. Since this transition is related to longer length scale.
Figure 4. The temperature dependence of the zero-field cooled (ZFC) and the field-cooled (FC) magnetisation of TbMnO3 nanorods for different magnetising field (A–H) and the irreversibility temperature in TbMnO3 nanorods at different magnetising field (I).
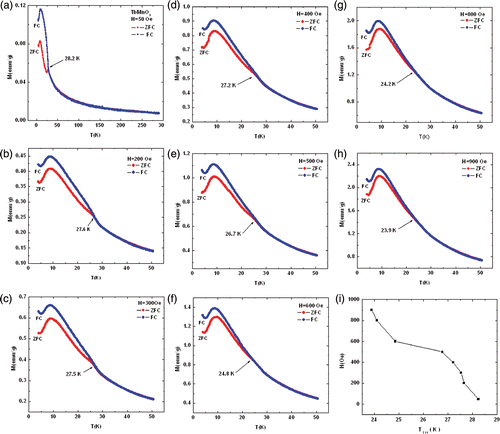
A separation between the ZFC and FC curves is observed below 30 K (). As the magnetising field (H) increases, the separation between ZFC and FC curves decreases and peak magnetisation values increase. There is almost no difference between the ZFC and FC curves above 30 K. Below 50 K, the Magnetisation curve increases dramatically owing to a weak ferromagnetism below a Neel temperature T N. also shows that a small spur is observed near the temperature where the separation between ZFC and FC curves occur, showing the existence of irreversibility temperature in TbMnO3 nanorods. The irreversibility temperature decreases from 28.2 to 23.9 K with the external magnetic field (H) increasing from 50 to 900 Oe, respectively (). The irreversibility phenomenon is a typical feature of spin glass and cluster glass-like systems, when a frustration between the antiferromagnetic and ferromagnetic ordering occurs. These interactions arise as the Mn–O–Mn bond angle gives rise to intermediate interactions between antiferromagnetic 180° super exchange and ferromagnetic 90° super exchange Citation15. Here in this present work, the irreversibility in magnetisation curves suggest that the TbMnO3 nanorods exhibit cluster glass behaviour rather than spin glass behaviour. In the spin glass system, irreversibility in general, is observed at low temperatures far below the magnetic transition, whereas in the cluster glass system, the irreversibility is observed close below to the magnetic transition Citation16.
shows the M(H) of TbMnO3 nanorods at 4, 50, 75 and 100 K. The M(H) at 4 K shows a two-step magnetisation process, which may be ascribed to the spin reorientation of Tb3+ ions in Mn3+ sites owing to Tb3+ spin reversal. A slight magnetic hysteresis is observed at 4 K, and the magnetisation is almost saturated at high field, which is due to a weak ferromagnetism of Mn arrays. The value of the magnetisation M and the coercivity H decreases with the temperature increasing, and disappears at 50, 75 and 100 K, indicating that phase transition from antiferromagnetic to paramagnetic occurred below 50 K, i.e. a linear increase in the magnetisation. In addition, with increasing external field, the dc magnetisation M(H) increases linearly in the region of higher field. This suggests that the co-existence of FM and AFM.
4. Conclusions
Nanorods of TbMnO3 were synthesised by chemical co-precipitation route. The prepared sample was indexed to the orthorhombic structure from the XRD data. The SEM image confirmed nanorods of diameter 100 nm. A separation between the ZFC and FC curves was observed below 30 K. As the magnetising field (H) increases, the separation between ZFC and FC curves decreases and peak magnetisation value increases. The irreversibility temperature decreases from 28.2 to 23.9 K and the external magnetic field (H) increases from 50 to 900 Oe, respectively. The irreversibility in magnetisation curves suggests that TbMnO3 nanorods exhibit cluster glass behaviour rather than spin glass behaviour. A slight magnetic hysteresis is observed at 4 K. This simple technique of chemical co-precipitation route may prove to be a potential tool for growing similar nanostructures of other perovskite oxide. According to our knowledge, the TbMnO3 nanorods of average size 100 nm, showing cluster glass behaviour is first time reported by us.
Acknowledgements
We thank Dr. A. Bharathi and A.T. Satya of Materials Science Group, IGCAR, Kalpakkam for the magnetisation measurements.
References
- Niitaka , S , Azuma , M , Takano , M , Nishibori , E , Takata , M and Sakata , M . 2004 . Crystal structure and dielectric and magnetic properties of BiCrO3 as a ferroelectromagnet . Solid State Ionics , 172 : 557 – 559 . doi: 10.1016/j.ssi.2004.01.060
- Baettig , P , Ederer , C and Spaldin , N A . 2005 . First principles study of multiferroics BiFeO3 . Bi2FeCrO6, and BiCrO3: Structure, polarization, and magnetic ordering temperature, Phys. Rev. B , 72 : 214105 – 214112 .
- Montanari , E , Calestani , G , Migliori , A , Dapiaggi , M , Bolzoni , F , Cabassi , R and Gilioli , E . 2005 . High temperature polymorphism in metastable BiMnO3 . Chem. Mater. , 17 : 6457 – 6467 . doi: 10.1021/cm051576w
- Wang , Y , Jiang , Q H , He , H C and Nan , C W . 2006 . Multiferroic BiFeO3 thin film prepared via a simple sol–gel method . Appl. Phys. Lett. , 88 : 142503 – 142505 . doi: 10.1063/1.2191947
- Belik , A A , Iikubo , S , Kodama , K , Igawa , N , Shamoto , S , Niitaka , S , Azuma , M , Shimakawa , Y , Takano , M , Izumi , F and Takayama-Muromachi , E . 2006 . Neutron powder diffraction study on the crystal and magnetic structure of BiCoO3 . Chem. Mater. , 18 : 798 – 803 . doi: 10.1021/cm052334z
- Belik , A A , Iikubo , S , Kodama , K , Igawa , N , Shamoto , S , Maie , M , Nagai , T , Matsui , Y , Stefanovich , S Y , Lazoryak , B I and Takayama-Muromachi , E . 2006 . BiScO3: Centrosymmetric BiMnO3-type oxide . J. Am. Chem. Soc. , 128 : 706 – 707 . doi: 10.1021/ja057574u
- Kimura , T , Goto , T , Shintani , H , Ishizaka , K , Arima , T and Tokura , Y . 2003 . Magnetic control of ferroelectric polarization . Nature , 426 : 55 – 58 . doi: 10.1038/nature02018
- Kimura , T , Lawes , G , Goto , T , Tokura , Y and Ramirez , A P . 2005 . Magnetoelectric phase diagrams of orthorhombic RMnO3 (R=Gd . Tb, and Dy), Phys. Rev. B , 71 : 224425 doi: 10.1103/PhysRevB.71.224425
- Kirby , B J , Kan , D , Luykx , A , Murakami , M , Kundaliya , D and Takeuchi , I . 2009 . Anomalous ferromagnetism in TbMnO3 thin films . J. Appl. Phys. , 105 : 1 – 3 .
- Cui , Y M , Wang , C C and Cao , B S . 2005 . TbMnO3 epitaxial thin films by pulsed-laser deposition . Solid State Commun. , 133 : 641 – 645 . doi: 10.1016/j.ssc.2005.01.002
- Wu , K H , Gou , I C , Luo , C W , Uen , T M , Lin , J-Y , Juang , J Y , Chen , C K , Lee , J M and Chen , J M . 2010 . Anisotropic electronic structure in single crystalline orthorhombic TbMnO3 thin films . Thin Solid Films , 518 : 2275 – 2279 . doi: 10.1016/j.tsf.2009.09.089
- Kharrazi , S , Kundaliya , D C , Gosavi , S W , Kulkarni , S K , Venkatesan , T , Ogale , S B , Urban , J , Park , S and Cheong , S-W . 2006 . Multiferroic TbMnO3 nanoparticles . Solid State Commun. , 138 : 395 – 398 . doi: 10.1016/j.ssc.2006.03.034
- Han , J T , Huang , Y H , Huang , W and Goodenough , J B . 2006 . Selective synthesis of TbMn2O5 nanorods and TbMnO3 micron crystals . J. Am. Chem. Soc. , 128 : 14454 – 14455 . doi: 10.1021/ja065520u
- Quezel , S , Tcheou , F , Rossat-Mignod , J , Quezel , G and Roudaut , E . 1977 . Magnetic structure of the perovskite-like compound TbMnO3 . Physica. B , 86–88 : 916 – 918 .
- Coey , J MD , Viret , M and von Molnar , S . 1999 . Mixed valence manganites . Adv. Phys. , 48(2) : 167 – 293 . doi: 10.1080/000187399243455
- Wu , J , Lynm , J M , Glinka , C J , Burley , J , Zheng , H , Mitchell , J F and Leighton , C . 2005 . Intergranular giant magnetoresistance in a spontaneously phase separated perovskite oxide . Phys. Rev. Lett. , 94 : 037201 – 037204 . doi: 10.1103/PhysRevLett.94.037201